Introduction
Amino acids (AAs) are natural substances containing both amino and acid groups. Proline, which contains an imino group but not an amino group, is loosely considered as an AA in nutrition and metabolism.1 Protein (the polymer of AAs) is the major component of growth in animals and has been a focus of nutritional research over the past century.2 Proteinogenic (protein-creating) in animals are alanine, arginine, asparagine, asparate, cysteine, glutamate, glutamine, glycine, histidine, isoleucine, leucine, lysine, methionine, phenylalanine, proline, serine, threonine, tryptophan, tyrosine, and valine. Selenocysteine that occurs in selenoproteins is formed from selenium and serine during the process of mRNA translation. AAs that are not precursors of proteins are called non-proteinogenic AAs, and some of them (e.g. taurine) fulfill essential physiological functions, but are either not synthesized by certain animals (e.g. cats) or inadequately synthesized in many species of fish.3 Note that animals have no requirements for pure nitrogen.2
Structural support and function of animals require collagens, which account for one-third of all proteins in the body. About 31% of proline residues in collagens undergo post-translational modification to 4-hydroxyproline and 3-hydroxyproline.4 The content of hydroxyprolines in animals increases with age until reaching sexual maturity, but the composition of all proteinogenic AAs is largely similar among different species of growing animals (Table 1), with exceptions for certain AAs (e.g. glycine and arginine) in some fish (e.g. largemouth bass and hybrid-striped bass)3 and shrimp,4 as well as glycine, cysteine, and methionine in rabbits.5 Like taurine, 4-hydroxyproline is a potent antioxidant and a scavenger of free radical species to protect the intestine and other tissues from oxidative damage.6,7 4-Hydroxyproline is a major substrate for glycine synthesis in tissues (e.g. the kidneys, liver, small intestine, and skeletal muscle) of animals.7 Of note, glycine, proline, and glutamate are the first, second, and third most abundant AAs, respectively, in the body. The intracellular synthesis of protein requires the simultaneous presence of all proteinogenic AAs in adequate amounts and proper ratios and, therefore, is limited by a deficiency of any proteinogenic AAs (including those that are synthesized de novo) at any given time.2 In other words, all proteinogenic AAs are physiologically and nutritionally (as shown below) essential for protein synthesis, growth, development, and health in animals, such as pigs, poultry, and fish. This article highlights the historical development of and recent revisions to the long-standing concept of “ideal protein” in the nutrition of the farm animals. Because they are not only species of agricultural importance, but also useful models to study the biology and diseases of humans and extinct animals in the world,8–10 our work applies to a more general readership than the nutritionists and producers of farm animals. In this article, proteinogenic AAs, except for glycine, refer to their l-isomers, unless specifically indicated.
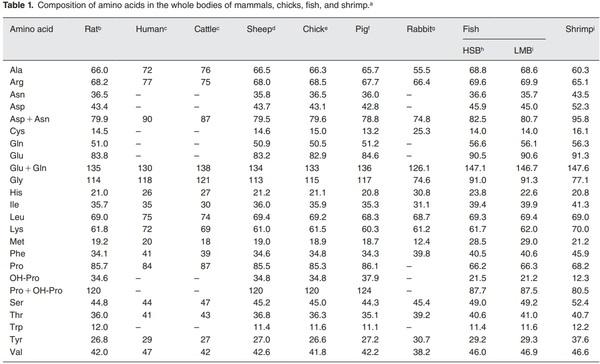
Values are mg amino acid/g protein.
a Unless indicated otherwise, calculations were based on the molecular weights of intact AAs.
b Adult rats (60day-old in the postabsorptive state) without intestinal lumen contents.11
c Data for human fetuses (days 160–280 of gestation) and cattle (12-week old) were obtained from Davis et al.12 It was not reported whether calculations were based on the molecular weights of intact AAs or AA residues.
d Adult sheep (12-month old) without intestinal lumen contents.11
e Chickens (10-day old chickens in the postabsorptive state) without intestinal lumen contents.11
f Pigs (30-day old in the postabsorptive state) without intestinal lumen contents.11
g Fifty-three-day-old New Zealand White rabbits (males and females).5
h Juvenile hybrid-striped bass (HSB; 50g of body weight) without intestinal lumen contents.3
i Juvenile largemouth bass (LMB; 50g of body weight) without intestinal lumen contents.3
j Whole body of the whiteleg shrimp (Litopenaeus vannamei; 15g of body weight) without intestinal lumen contents.13
Concepts of nutritionally essential AAs and nonessential AAs
Animal cells cannot synthesize the carbon skeletons of the following 11 proteinogenic AAs de novo: cysteine, histidine, isoleucine, leucine, lysine, methionine, phenylalanine, threonine, tryptophan, tyrosine, and valine.2 Therefore, they have been classified as nutritionally essential AAs (EAAs) and must be included in diets for maintenance, growth, development, and survival in all nonruminants. By contrast, animal cells can synthesize the carbon skeletons of the following eight proteinogenic AAs de novo: alanine, asparagine, aspartate, glutamate, glutamine, glycine, proline, and serine, and their nitrogen is derived primarily from EAAs.2 Whether arginine synthesis occurs de novo depends on animal species (see below). Dietary non-AA nitrogen (e.g. 2% urea or ammonia) is effectively used for AA and protein synthesis by ruminal microbes in ruminants.14–16
Growing nonruminant mammals (e.g. pigs and rats) can utilize a small amount of dietary ammonia-nitrogen for AASA synthesis when diets are severely deficient in AASAs. For example, nitrogen in the form of diammonium citrate (providing 1.45 and 2.9% crude protein [CP]) added to soybean meal- and cornstarch-based diets containing a low-CP level (only 10.19%) can be used for AASA synthesis in a dose-dependent manner by growing pigs (15–27kg body weight).17 Similarly, 2.15% diammonium citrate added to a purified diet containing 8% EAAs (low-AA level) enhanced the growth of young rats during weeks 0–4 postweaning.18 Similarly, young chicks can use dietary diammonium citrate (1.51 and 3.02%) for enhancing weight gain in a dose-dependent manner when fed purified diets containing low (10%) total AAs.19 Interestingly, under this feeding condition, adding 0.8% urea to the low-AA diets also improved the growth of chicks,19 likely due to the synthesis of AAs by intestinal microbes, but dietary supplementation with 1.5% urea had no further effect.19 Note that (1) animal cells lack urease, but the intestinal microbes of pigs and rats contain urease to hydrolyze urea into ammonia plus CO22 and (2) in all the previous studies involving low-CP or low-AA diets supplemented with a non-AA nitrogen source, young nonruminant animals had suboptimum growth rates.17–22
In contrast to ruminants, neither pigs20 nor rats21 can use dietary urea for a nutritionally significant synthesis of AAs or tissue growth. Indeed, adding 2% urea to wheat- and fishmeal-based diets (22% CP) for growing pigs (4–11 weeks of age) reduced their daily feed consumption, weight gain, and feed efficiency,20 possibly due to the toxicity of ammonia produced from the ingested urea by intestinal microbes. Likewise, adding 1% urea or 2.2% diammonium hydrogen phosphate to the basal starter diet (containing 20.6% CP; nearly adequate diet) of broiler chickens (0–4 weeks of age) depressed their growth and feed conversion as compared with the control diet (23.5% CP), whereas adding 0.5% urea and 1.1% diammonium hydrogen phosphate to the basal finisher diet (containing 18% CP; nearly adequate diet) of broiler chickens (4–7 weeks of age) did not affect their weight gain or feed conversion efficiency in comparison with the control diet (20% CP).22
The AAs that are synthesizable de novo in animals (AASAs) have been termed nutritionally nonessential amino acids (NEAAs) since the early 1910s,23 and they were thought to be sufficiently formed in animals and dispensable in their diets. However, this view has not been substantiated by experimental results from animal studies over the past 110 years.24–26 De novo synthesis of arginine in animals depends on their species, as this metabolic pathway exists in most mammals (including pigs, cattle, sheep, rats, and many breeds of dogs), is limited in cats, and is absent in some mammals (e.g. ferrets and minks), birds, and possibly many species of fish.27 It should be borne in mind that NEAAs and EAAs account for 60 and 40% of total AAs in animals, respectively.28 This indicates a greater requirement of animals for NEAAs than EAAs for protein synthesis in tissues. However, such a fundamental knowledge hadnot received much attention from nutritionists until recent years.3,11,13,26,29–31 The traditional view that animals have no dietary requirements for NEAAs must be refuted in light of the new knowledge of AA biochemistry, nutrition and physiology. The term “NEAAs” has now been recognized as a misnomer in nutritional science30 and, therefore, should be replaced by AAs that are synthesizable de novo in animal cells (AASAs).
Historical development of the “ideal protein” concept in chicken nutrition
The concept of “ideal protein” (a protein with an optimal EAA pattern that precisely meets the physiological needs of animals) in nutrition was initially proposed in the late 1950s by poultry nutritionists at the University of Illinois for chicken diets.32,33 Guided by the textbook view of the time that animals sufficiently synthesized de novo all AASAs, the conceptualization of an “ideal protein” concerned arginine, histidine, isoleucine, leucine, lysine, methionine, phenylalanine, threonine, tryptophan, and valine, but not AASAs.33 The early researchers used the composition of EAAs in both casein and chicken eggs as the experimental basis to formulate purified diets for young chicks, but were largely unsuccessful for weight gain and feed efficiency partly because of the imbalances and excessive amounts of many EAAs. In 1960, based on EAA profiles in the chick carcass, Klain et al.34 revised the dietary content of AAs with the following crystalline AAs (% of diet) to feed 7-day-old male broilers for 5–7 days: arginine–HCl, 1.40; histidine–HCl, 0.51; lysine–HCl, 1.54; tyrosine, 0.80; tryptophan, 0.18; phenylalanine, 1.18; cystine, 0.40; dl-methionine, 0.20; dl-threonine, 1.16; leucine, 1.66; dl-isoleucine, 1.68; dlvaline, 1.92; glycine, 0.50; and glutamic acid (the sole source of nonspecific nitrogen), 15.00. The authors observed that the daily weight gain of the chicks was enhanced with the revised “ideal protein,” but remained largely unsatisfactory for maximum growth rate and feed efficiency.34 Note that the content of total EAAs in chicks and other animals is much lower than that of total AASAs (Table 1).5,11,12
During the late 1960s and the early 1970s, Baker et al.35,36 included proline along with cystine, glycine, and glutamic acid in purified diets for broilers, which led to an improvement in their growth performance. In birds, as in mammals, cysteine is generated from methionine in hepatocytes; glycine from serine, threonine, and choline; proline from arginine; and glutamate from glutamine and ammonia plus α-ketoglutarate in a cell- and tissue-specific manner.37,38 In those and the earlier studies, glutamic acid was used to provided nonspecific AA nitrogen, but was not considered to be required in poultry diets. These findings led to sequentially three versions of the “chick AA requirement standard” for 1- to 21-day-old broilers.39–41 In 1994, Baker and Han42 revised the reference values for EAAs in the diets of broilers to further improve their feed efficiency (Table 2). Note that all the proposed versions of the “ideal protein” for chick diets33–36,39–42 did not contain alanine, aspartate, asparagine, glutamine, or serine; differed substantially for glycine and proline and, to a lesser extent, for branched-chain AAs, histidine, and sulfur-containing AAs (Table 2); and were arbitrarily set to contain only a relatively small amount of proline and glycine. This is likely due to significant variations in the reported AA composition of the chicken body43,44 and the lack of crucial information on the content of many abundant AASAs (including proline plus 4-hydroxyproline, glutamate, glutamine, aspartate, and asparagine) in chicks during the 1950s–the 1990s.
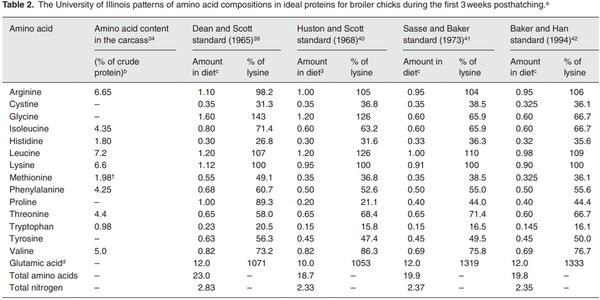
a These ideal protein models were developed for 0–21-day-old broilers using crystalline amino acids. It was assumed that all of these amino acids were 100% available for absorption into enterocytes in chicks. Except for glycine and methionine, all amino acids are l-isomers. dl-methionine is used herein.
b Average values for 1-week-old and 4–5-week-old chicks.
c % of diet (as-fed basis; 90% dry matter).
d Provided as the nitrogenous source for synthesis of all NEAA in chicks.
†This value refers to l-methionine.
It is noteworthy that the dietary content of glutamic acid in the previous studies (e.g. 13 times that of lysine)41 to provide chicks with “nonspecific AA nitrogen” is particularly high. Glutamate shares the same transporters with aspartate, cysteine, and cystine in animal cells, and also actively participates in the metabolism of many AAs (including branchedchain AAs, glutamine, and proline).2 Thus, there is concern that a great excess of glutamic acid in diets may result in AA and acid–base imbalances, as well as abnormalities in transmembrane AA transport, interorgan AA metabolism, and neurological function in chickens. Such an issue was not addressed in all the studies of the University of Illinois researchers.33–36,39–42 Possibly due to the forementioned concern and the 1994 publication of the ninth revised edition of Nutrient Requirements of Poultry by National Research Council (NRC) of the United States,45 glutamic acid, glycine, and proline were excluded in 1997 by Baker46 from the final version of the “ideal protein” for the diets of 0–56-day-old broiler chickens (Table 3). This decision might have resulted from an insufficient understanding of avian AA metabolism before the 2000s. Sufficient dietary supply of glutamate, glycine, and proline is crucial for maximum growth and production performance in poultry, including chickens.13,26
Historical development of the “ideal protein” concept in swine nutrition
Like chickens, pigs are monogastric animals. Thus, the previous extensive research on developing the “ideal protein” for poultry diets provided useful framework for swine studies in the 1980s and 1990s. In 1980, by simulating AA composition in the carcass of growing pigs, Cole48 proposed “optimum” dietary ratios of EAAs (relative to lysine) for their feeding. This concept was accepted in the subsequent year by the Agricultural Research Council (ARC) of the United Kingdom49 and in 1988 by the NRC.50 However, the ARC’s version of the “ideal protein” for swine did not include two EAAs (histidine and arginine) and all AASAs (Table 4). The absence of histidine from the conceptualized “ideal protein” in swine diets lacked a sound biochemical and physiological basis because this AA is an essential constituent of all proteins but is not synthesized de novo in any animal cells.2 In addition, the exclusion of arginine from the study diet51 likely resulted from a lack of knowledge about arginine metabolism and nutrition in swine before the 1990s.25 Also, the conceptual foundation of the “ideal protein” on the composition of EAAs in the body or carcass is flawed, because the ratios of EAAs in animals (including swine) do not match those in diets due to the following factors.2,28 First, all AAs in the diet undergo catabolism at different rates in the mucosa (mainly enterocytes), microbes in the small intestine, or both. Due to the extensive first-pass metabolism of dietary AAs in the portal-drained viscera, the concentrations of AAs in plasma are very different from those in the ingested food (e.g. milk, forage, and corn- and soybean meal-based feed). Third, proteinogenic AAs in plasma are taken up by cells in animals at various rates and have various metabolic fates via diverse cell-specific metabolic pathways. Fourth, the types and AA composition of proteins in animals differ from those in plant-based foods. For these reasons, the usefulness of the early versions of the “ideal protein” for swine feeding is limited.
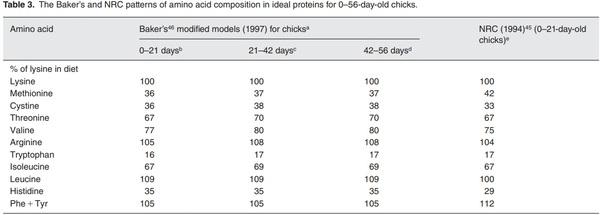
a These ratios are based on true digestible levels of amino acids in diet (as-fed basis; 90% dry matter). Adapted from Baker.46,47 Except for glycine, all amino acids are l-isomers.
b Patterns of amino acid composition in the ideal protein are the same for male and female chickens. The amounts of digestible lysine in diet (as-fed basis; 90% dry matter) are 1.12 and 1.02% for male and female chickens, respectively.
c Patterns of amino acid composition in the ideal protein are the same for male and female chickens. The amounts of digestible lysine in diet (as-fed basis; 90% dry matter) are 0.89 and 0.84% for male and female chickens, respectively.
d Patterns of amino acid composition in the ideal protein are the same for male and female chickens. The amounts of digestible lysine in diet (as-fed basis; 90% dry matter) are 0.76 and 0.73% for male and female chickens, respectively.
eThese ratios are based on total amino acids in a typical corn- and soybean meal-based diet.45 The amount of digestible lysine in diet (as-fed basis; 90% dry matter) is 1.2% for 0–21-day-old chicks.
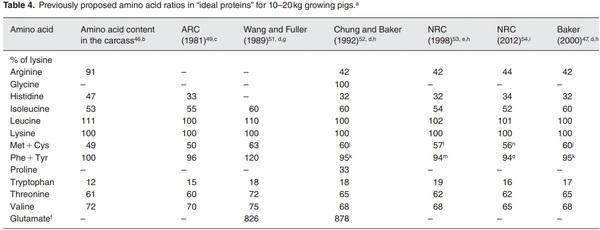
a These ratios are based on true digestible levels of amino acids in diet, except for ARC.49 Except for glycine, all amino acids are l-isomers.
b The body proteins in 20–45kg pigs contain 63g lysine/16g nitrogen.
c These ratios are based on total amino acids in the diet. The total level of lysine in the diet is 1.10% (as-fed basis; 90% dry matter).
d The diet contains 1.20% true digestible lysine (as-fed basis; 90% dry matter).
e The diet contains 1.01% true digestible lysine (as-fed basis; 90% dry matter).
f Provided as the nitrogenous source for synthesis of other NEAA in animals.
g Dietary requirements are for 25–50kg gilts.
h Dietary requirements are for 10–20kg pigs.
i Total amino acid in a typical corn- and soybean meal-based diet (as-fed basis; 90% dry matter). The diet contains 1.40% total lysine.54
j The ratio of l-methionine to l-cystine is 1:1.
kThe ratio of l-phenylalanine to l-tyrosine is 53:47.
l The ratio of l-methionine to l-cystine is 47:53.
m The ratio of l-phenylalanine to l-tyrosine is 64:36.
n The ratio of l-methionine to l-cystine is 51:49.
o The ratio of l-phenylalanine to l-tyrosine is 63:37
During the late 1980s, Wang and Fuller51 used young gilts (25–50kg) in feeding trials to revise EAA patterns in the earlier “ideal protein” by considering maintenance requirements for AAs. Disappointingly, the authors also did not consider arginine, histidine, and AASAs in the “ideal protein.” The semi-purified diets used in the study of Wang and Fuller51 included a high amount of glutamic acid as the sole source of nonspecific AA nitrogen (826% of the lysine content). Unfortunately, the content of glutamate in the feedstuffs used for all those previous studies was not determined due to technical difficulties.46,48–51 As in the earlier work with chickens noted previously, Wang and Fuller51 did not consider that (1) such a high intake of glutamate by swine may have possible adverse effects on animal metabolism and health (including intestinal health) and (2) growing pigs have a dietary requirement for glutamic acid and glutamine. Adequate provisions of these two AA in diets are crucial for the maximum growth, optimum intestinal health, and maximum production performance of pigs.55,56
During the 1990s, Baker’s group52 recognized some of the deficiencies in the “ideal protein” for swine diets and, therefore, conducted a series of feeding trials to determine optimum ratios of EAAs to lysine in the purified diets for 10–20kg pigs. In their study,52 the basal diet contained 1.2% true digestible lysine and the various ratios of the following five AAs relative to lysine (arginine, 0.42; glycine, 1.00; histidine, 0.32; and proline, 0.33, and glutamic acid (the sole source of nonspecific AA nitrogen), 8.78. The inclusion of arginine, glycine, histidine, and proline in the swine diets was a significant improvement over Wang and Fuller’s version of the “ideal protein” for gilts.51 However, the “ideal protein” of Chung and Baker52 for swine diets still did not include (1) cysteine and tyrosine, which are not synthesized de novo in any animal cells; and (2) many AASAs (alanine, aspartate, asparagine, glutamine, and serine), which are abundant in the body and actively participate in metabolism, including one-carbon metabolism and the synthesis of purine and pyrimidine nucleotides.2 In addition, although Baker’s group,41,52 like other researchers,34,51 used glutamic acid to formulate isonitrogenous diets for animal feeding, none of these investigators considered that animals have dietary requirements for glutamic acid and glutamine for optimal growth, intestinal health, and production performance.55,56 There is no experimental evidence that the supplemental glutamate supports an adequate synthesis of all other AASAs (including alanine, asparagine, aspartate, glutamine, and serine) in pigs. Furthermore, Chung and Baker52 did not explain why different amounts of arginine, glycine, histidine, and proline were included in the diets for growing pigs. Unfortunately, the 10th edition of the Swine Nutrient Requirements by the NRC53 did not recognize that young pigs have dietary requirements for proline and glycine. Possibly due to this official publication, Baker47 excluded these two AAs from his last version of the “ideal protein” for swine diets. Disappointingly, guided by the outdated concept of the ideal protein, the NRC54 also failed to recognize the important role of dietary glutamate, glycine, and proline in swine nutrition. These AAs, along with glutamine and arginine, are crucial for maximum growth and production performance in swine.2,25,55–57
Moving beyond the “ideal protein” concept
The “ideal protein” concept has greatly advanced the field of swine and poultry protein nutrition over the last four and six decades, respectively, resulting in the practice of dietary supplementation with crystalline EAAs to improve the growth, feed efficiencies and productivity of pigs and poultry.14,37,58–62 This nutritional concept has also been adopted by the dairy cattle industry to provide rumen-escape protein or EAAs for high-producing cows14 and highlighted in the literature concerning the nutrition of other farm animals, including fish and crustaceans.63 In addition, the “ideal protein” concept is now also used to feed zoo64 (e.g. carnivores) and companion65,66 (e.g. cats and dogs) animals. However, the “ideal protein” concept is imperfect in animal nutrition for the following reasons. First, the composition of AAs in the diet is not the same as that in the whole body due to the catabolism of dietary AAs by the mucosa, microbes, or both of the small intestine at various rates, as indicated previously.28 In addition, the composition of AAs (particularly glycine, proline, and hydroxyproline) in the animal body varies with age4 and, therefore, data on carcass or muscle AA composition at one particular age may not be applicable to other ages. Second, EAAs are degraded in extraintestinal tissues at various rates via a cell-specific manner, resulting in the synthesis of AASAs (particularly glutamate, glutamine, glycine, and proline) at various rates.2 Third, the amounts of EAAs and the ratios of EAAs to lysine in diets are only some of the factors that affect protein nutrition in animals. As noted previously, animals have dietary requirements for AASAs for realizing their genetic potentials for maximum growth and production performance (e.g. embryonic survival, milk yield, and postweaning survival) and optimum health (e.g. intestinal health in weanling piglets, antioxidative responses in endotoxin-challenged pigs, and the resistance of pigs and rodents to pathogens).67 Sufficient AASAs must be provided in diets. Fourth, the “ideal protein” concept does not take, into consideration, the needs of animals for dietary AASAs for optimum metabolic processes and intestinal microbial metabolism.
Fifth, the “ideal protein” concept ignores the nutritional and physiological needs of the small intestine, the reproductive system, and immunocytes of animals (including pigs, chickens, and fish) for AASAs, which are not only major energy sources for enterocytes but also key regulators of gene expression, gut integrity, spermatogenesis, embryonic survival, immunity, and health.3,13,29,56,67–69 For example, dietary glutamate, glutamine, glycine, and proline protect the small intestine from oxidative stress and atrophy in weanling pigs,2,55–57 whereas dietary arginine enhances placental water transport and nitric oxide (NO) synthesis, as well as embryonic and fetal survival in gestating swine.69–71 Furthermore, a lack of glutamate or glutamine in diets results in high rates of mortality in juvenile hybrid-striped bass.72 Thus, although poultry and swine nutritionists have adopted the “ideal protein” concept for animal feeding for over 60 and 40 years, respectively, the production performance and feed conversion efficiencies of swine and poultry remain suboptimum.73–75 For example, adequate provision of arginine, glutamate, glutamine, glycine, and proline in diets are required to improve health (including intestinal and reproductive health), growth performance, lactation, and productivity of swine.25,28,68,73 In addition, sufficient dietary glutamate and glutamine are also essential for maximum lean tissue gain in broiler chickens particularly under stress conditions.26,29 Furthermore, although there have been good progress in the manufacturing of environmentally sustainable, low-CP diets based on the “ideal protein” concept to meet the requirements of swine and poultry for all EAAs, all the attempts without the inclusion of AASAs in diets have not been successful for the maximum growth or productivity of the animals.76–79
In view of the foregoing, we suggest that the “ideal protein” concept be replaced with “optimum ratios and amounts of all proteinogenic AAs” for animals. This new concept provides the much-needed scientific basis for formulating low-protein diets with AASAs, such as glutamate, glutamine, glycine, proline, and serine. Dietary provision of these AAs that are highly abundant in the body will minimize the needs for both dietary EAAs and energy to synthesize AASAs via cell-specific metabolic pathways. Disappointingly, AASAs are currently not considered in most studies for reducing CP content in animal diets. Readers are referred to recent excellent reviews on this topic.62,80 The revision of the “ideal protein” concept by the integration of AASAs can improve AA provision in the diets of animals. Because animals have dietary requirements for AAs but not protein,2 a term “optimal ratios and amounts of all proteinogenic AAs” is scientifically more accurate and practically more useful than the “ideal protein.” In the case of carnivorous species, taurine should also be provided in their diets to prevent damage to the eyes, heart, and other organs. Of note, there has been increasing interest in the use of protein hydrolysates (including small peptides) and functional AAs (in the crystalline or liquid form) to feed animals.2,62
Optimal ratios and amounts of all proteinogenic AAs in diets for swine and chickens, as well as companion and zoo animals
The proportions of skeletal muscle, connective tissue, white adipose tissue, and other tissues in animals (such as pigs and rats) change with the increase of age and may differ among different breeds of the same species.2,28 In addition, the proportions of specialized proteins (e.g. collagens) in animals increase with their growth.4 Thus, although the composition of AAs in individual proteins is generally not influenced by diet and disease, the composition of AAs and the rates of AA metabolism in animals (expressed per kg body weight) vary during their life cycles. Therefore, the requirements of animals for proteinogenic AAs and, in the case of carnivores, also taurine are not fixed but rather undergo dynamic changes during different physiological stages and in response to alterations in genotypes and environmental factors (including dietary composition, ambient temperatures, and infections). Furthermore, with improvements in animal-breeding techniques to produce new breeds of livestock, poultry, and fish, their lean tissue grows much faster than the previous breeds, and in the case of swine, a marked increase in both litter size at birth and skeletal muscle gain.73–75 Also, considerations for sustainability and value chain in animal agriculture call for precision nutrition in feeding practices. Adequate knowledge of AA metabolism and nutrition is necessary to meet the needs of animals for dietary AAs.81
Our guiding principles for the development of new optimal patterns of all AAs in diets for swine and chickens at a production stage are (1) endogenous syntheses of AASAs from EAAs are inadequate in highly productive animals, (2) EAAs and AASAs are needed in optimal proportions and amounts for maximum productivity and optimum health, (3) dietary AAs are metabolized at different rates in the small intestine of animals, and (4) dietary requirements of animals for AAs depend on tissue-specific protein turnover and AA metabolism. Since 1992, extensive research has been conducted to improve AA patterns in diets for gestating, lactating, suckling, weanling, and finishing swine through both basic research on AA metabolism and dietary supplementation with functional AAs (including arginine, glutamine, glutamate, proline, or glycine).2,25,28,68,69,82 Research to refine dietary requirements of lactating sows for AAs (including AASAs) has also been advanced through the determination of their mammary gland growth and development, wholebody composition of all proteinogenic AAs, as well as milk composition and production.2,12,28,59,61 The quantifiable outcomes are increases in the number and litter weight of live-born piglets, the growth and survival rates of neonatal and postweaning piglets, the milk yield of lactating sows and their subsequent pregnancy rates, as well as immunity and resistance to infectious pathogens. Similarly, the past 30 years have witnessed remarkable progress in the productivity of birds (e.g. growth rate, feed efficiency, and egg production).26,37,60,75
With advances in our understanding of AA biochemistry and nutrition over the past three decades, Wu and coworkers have proposed optimal ratios and amounts of true digestible AAs in diets for swine (Table 5) and chickens (Table 6) during the different phases of their growth and production. Values for cats83 as well as carnivorous and omnivorous zoo animals31 have also been proposed in recent years. Because the dynamic requirements of animals for dietary AAs are influenced by a plethora of factors as indicated previously, the data in Tables 5 and 6 as well as the literature31,83 serve only as references to guide feeding practices and nutritional research. Diets should be formulated for animals on farms under their practical production conditions for optimum nutrient efficiencies. Note that (1) the recommendations include AASAs in the optimal ratios and amounts of dietary AAs for swine and poultry, as well as other animals at various production stages and (2) animals have particularly high requirements for dietary arginine, glutamate, glutamine, glycine, and proline for optimum intestinal health, and these AAs are very abundant in animal-sourced feedstuffs, including blood meal, chicken visceral digest, feather meal, insect meal, intestinal mucosal and peptone products, meat and bone meal, and poultry by-products.84 These ingredients can increase both the sustainability of animal agriculture and value chain in animal nutrition. Of particular note, AASAs have now been used to cost-effectively manufacture diets for swine,25,69,85 poultry,86,87 fish,88 and crusyacean13 to enhance the efficiency of lean tissue growth. The optimal ratios and amounts of AAs in animal diets can reduce their protein content and feedstuff costs as well as the excretion of nitrogenous wastes and release of greenhouse gasses (e.g. methane and CO2) to the environment, while sustaining global animal agriculture.2,78,89-91 Additional benefits are improvements in growth, production performance, and food efficiency in livestock and companion animals, as well as the survival and expansion of extinct animals in the world.2,31 Furthermore, because the current Institute of Medicine publication, which does not adopt the “ideal protein” concept for the requirements of humans for dietary AAs, ignores the requirements of humans for AASAs,92 findings from farm and laboratory animals may have important implications for improving the nutrition of infants (particularly preterm babies), children, and adults (particularly the elderly).
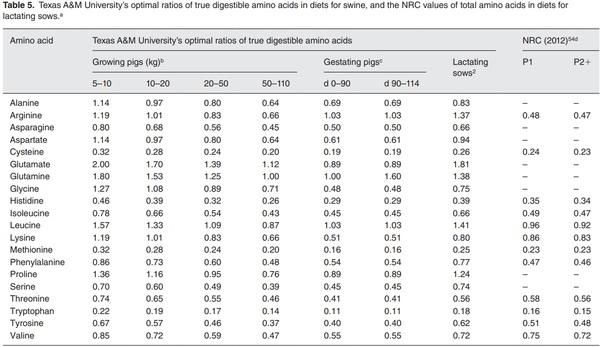
a Data for Texas A&M University’s optimal ratios of true digestible amino acids are taken from Wu.67 Except for glycine, all amino acids are l-isomers. Values are based on true ileal digestible amino acids. Crystalline amino acids (e.g. feed-grade arginine, glutamate, glutamine, and glycine), whose true ileal digestibility is 100%, can be added to a diet to obtain their optimal ratios. The molecular weights of intact amino acids were used for all the calculations. The content of dry matter in all the diets was 90%. The content of metabolizable energy in the diets of growing pigs, gestating pigs, and lactating pigs is 3330, 3122, and 3310kcal/kg diet, respectively.
b Fed ad libitum (90% dry matter).
c Fed 2kg/day on days 0–90 and 2.3kg/day on days 90–114 (90% dry matter).
d Data from National Research Council (NRC 2012) refer to total amino acids in a typical corn- and soybean meal-based diet.54 Dry matter content of the diet is 90%. P1=parity 1; P2+=parity 2 or greater.
Conclusions
As the term “ideal protein” implies for nutrition, it should be a protein with optimal ratios of all AAs required for maximum growth and productivity, as well as optimum metabolic processes, intestinal microbial metabolism, and health in animals. However, the “ideal protein” concept, which was originally proposed in the 1950s and based solely on EAAs but ignored AASAs, is imperfect in animal nutrition. The long-standing term “NEAAs” has recently been recognized as a misnomer in nutritional science and, therefore, replaced by AASAs. Not all AASAs are sufficiently synthesized by mammals, birds, fish, and crustaceans for their maximum productivity. An ideal diet must provide all EAAs and AASAs (in the cases of carnivores, also taurine) in proper ratios and sufficient amounts. AASAs must be adequate in diets for minimizing the environmental impact of animal production. Animal-sourced feedstuffs supply abundant EAAs and AASAs (including glutamate, glutamine, glycine, proline, 4-hydroxyproline, and taurine) for the diets of both vertebrates and invertebrates to improve their growth, development, reproduction, and health. Nutritionists should think outside the box and move beyond the “ideal protein” concept to consider optimum ratios and amounts of all proteinogenic AAs in animal foods, and in the case of strict carnivores, also taurine in diets. This will help formulate effectively low-protein diets for livestock (including swine and high-producing dairy cattle), poultry, fish, and crustaceans, as well as zoo and companion animals, while sustaining global animal production.
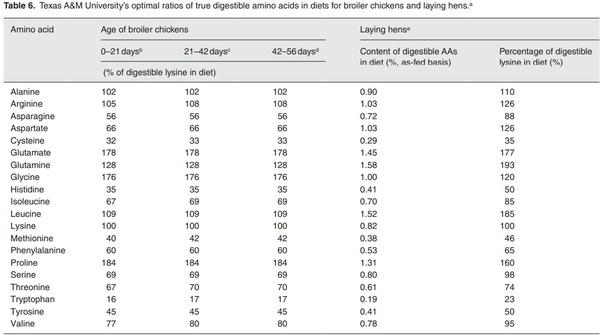
Adapted from He et al.26
a Except for glycine, all amino acids are l-isomers. Values are based on true ileal digestible amino acids.
b Patterns of amino acid composition in the ideal protein are the same for male and female chickens. The amounts of digestible lysine in diet (as-fed basis; 90% dry matter) are 1.12 and 1.02% for male and female chickens, respectively.
c Patterns of amino acid composition in the ideal protein are the same for male and female chickens. The amounts of digestible lysine in diets (as-fed basis; 90% dry matter) are 0.89 and 0.84% for male and female chickens, respectively.
d Patterns of amino acid composition in the ideal protein are the same for male and female chickens. The amounts of digestible lysine in diets (as-fed basis; 90% dry matter) are 0.76 and 0.73% for male and female chickens, respectively.
e A diet that consists of 60% corn grain (containing 9.3% crude protein) and 24% soybean meal (43.5% crude protein), and is supplemented with 0.2% glycine and 0.1% l-methionine can meet the requirements of laying hens for all amino acids.
This article was originally published in Experimental Biology and Medicine 2022; 247: 1191–1201. DOI: 10.1177/15353702221082658. This is an Open Access article licensed under a Creative Commons Attribution License.