Heat stress pathophysiology
As birds are homeothermic animals, they are able to maintain a relatively constant body temperature by balancing the production and dissipation of heat within their body. The maintenance of a constant body temperature is regulated by thyroid hormones of which the concentration is regulated by the hypothalamus – pituitary – thyroid axis (HPT) of the neuroendocrine system. Upon stimulation of the thyroid gland by the thyroid stimulating hormone (TSH) from the pituitary, the main hormone released is thyroxine (T4). This prohormone is then converted either to 3,5,3’-triiodothyronine (T3) or revers T3 (rT3) which regulate and balance the animal’s body temperature (Darras et al., 2000; Helmreich and Tylee 2011; Melesse et al., 2011). Additionally, radiation, convection and conduction are used to release the excess of the heat produced from the body (Lara and Rostagno, 2013). Nevertheless, modern poultry genotypes, with great metabolic activity, are sensitive to elevated environmental temperature and humidity levels (Settar et al., 1999; Deeb et al., 2002). This leads to an imbalance between the body’s heat production and heat loss, a phenomenon also known as heat stress. As birds accumulate heat in their body, several physiological adaptations are induced i.e. water consumption will increase while feed intake will decrease. Additionally, they spend more time with their wings elevated and resting while panting is increased (Mack et al., 2013). Finally, if heat production exceeds the maximum heat loss threshold, either by intensity (acute heat stress) or duration (chronic heat stress) other changes affecting neuroendocrine and immune systems will occur (Glaser and Kiecolt-Glaser, 2005).
Heat stress and gut health
To allow the release of heat to the environment, the blood flow in birds subjected to heat stress is redirected from the core of the body to the periphery, e.g. the vascular system in the legs and feet. This system is regulated by shunts that bring cool venous blood into close proximity with arterial blood to promote heat dissipation to the environment. This cooling method requires an increase in blood circulation in the periphery, leading to a decrease in blood flow in the organs. Consequently, blood supply to the gut is decreased, with a significant deprivation in oxygen and nutrients leading to a leaky gut. This usually characterized by the translocation of bacteria and bacterial products such as lipopolysaccharides (LPS) (Cronjé, 2005). Leakage of LPS to the internal environment stimulates the immune response involving the production of pro-inflammatory cytokines such as IL-1α, IL-1β, IFN-ϒ and IL-6 (Lambert, 2014). The pro-inflammatory actions of IL-6 involve the activation of T-cells, B-cells and further inflammatory mediators, to eliminate damaged tissue or foreign material. However, IL-6 also signals directly to the hypothalamic-pituitary-adrenal (HPA) – axis, resulting in the secretion of adrenal corticosteroids which will attenuate the immune response of the animal (Beishuizen and Thijs, 2003; Silverman et al., 2005).
Heat stress and the sympathetic-adrenal-medullary system (SAM)
As a short-term stress response and thus during acute heat stress, the sympathetic-adrenal-medullary system SAM is one of the crucial components which acts to relieve the stress mechanism or to adapt to the stressful condition. Activation of SAM is a fast reaction where the hypothalamus activates the autonomic nervous system to initiate a defense reaction. SAM activation leads to adrenal medulla release of catecholamines with mainly adrenaline and noradrenaline. In turn, these hormones are able to increase the heart rate and blood pressure, stimulate the blood flow to the muscles in order to improve the panting, and to enhance the glyco- and lipolysis to boost the glucose levels and thus the energy supply. Additionally, receptors for these hormones are present on different immune cells and induce modulation of the immune’s system activity when triggered. It could lead to an increase in circulating lymphocytes, a shift in T helper cell proliferation from Th1 to Th2, and a transient increase in IgA antibodies and an increase in IL-6 production (Lara and rostagno, 2013; Tort and Teles, 2007).
Heat stress and the hypothalamus-pituitary-adrenal axis (HPA)
When the stressor is maintained, too high amounts of adrenaline can become toxic and the hypothalamus-pituitary-adrenal (HPA) axis becomes activated. The hypothalamus will release corticotropin releasing hormone (CRH) which will stimulate the pituitary gland to secrete some adrenocorticotropic hormone (ACTH). This ACTH will in turn act upon the adrenal cortex to stimulate the synthesis and release of steroids leading to cholesterol uptake. Cholesterol will further be converted into cortisol and corticosterone, the last one being the most important glucocorticoid in birds (Herman et al., 2016) (Fig.1). It has been shown that glucocorticoids are able to decrease serum levels of the thyroid hormones TSH, T3 and T4, which are not only able to regulate body temperature but are also involved in stimulation growth and development of birds (Melesse et al., 2011; Helmreich and Tylee, 2011).
Figure 1. Effect of stress on HPA-axis (S. Hiller-Sturmhöfel, 1998)
Next, glucocorticoid receptors exist in the cytoplasm of most cells including immune cell. This creating a bi-directional communication and feedback between the HPA and the immune system. For example, rising levels of systemic IL-6 will trigger the HPA axis leading to corticosterone production. Increasing plasma levels of corticosterone exerts a negative feedback on inflammatory cytokines secretion and its own production, allowing a return to homeostasis when the stressor is removed.
When heat stress is maintained for a long period, corticosterone has deleterious effects on the zootechnical performance and immunity (Dumbell at al., 2016; Herman et al., 2016).
It can be assumed that heat stress compromises the animal’s ability to mount an effective immune response to pathogens and must be considered as a risk factor for infections. High corticosterone levels cannot be maintained for long periods. So, when chronically exposed to high temperatures plasma corticosterone levels will decrease and, if no efficient countermeasures are taken to alleviate heat stress, birds will become hyperthermic and die.
Yeast paraprobiotic to compensate detrimental effects of heat stress
Exposure of production animals to high environmental temperatures in combination with high humidity levels results in high economic losses. For example, in the US it is estimated that the livestock industry loses about 1.69 to 2.36 billion dollars annually and that the poultry industry is responsible for about 128 to 165 million dollars (St-Pierre et al., 2003). Fortunately, recent studies have shown that the yeast paraprobiotic is able to alleviate the negative effects of heat stress in different animal species. For example, a trial performed in Mexico (Arce et al., unpublished data) showed that heat stress increased the body temperature of the animals, while supplementation with the selected yeast fraction was able to counterbalance this increase in body temperature when animals were raised under enhanced environmental temperatures (Graph 1).
Graph 1. Effect of yeast paraprobiotic on chick body temperature
Additionally, it was also shown that birds exposed to heat stress have better zootechnical performances (Graph 2 -3) and lower corticosterone concentrations (Graph 2) when they are receiving the selected yeast fraction (Sohail et al., 2012).
Graph 2. Influence of yeast paraprobiotic on body weight of broilers at 42 days of age
Graph3. Influence of yeast paraprobiotic on feed conversion ratio at 42 days of age
Graph 4. Influence of yeast paraprobiotic on survival rate at 42 days of age
Graph 5. Influence of yeast paraprobiotic on corticosterone concentrations (pg/ml) of birds raised under heat stress (adapted from Sohail et al., 2012)
Yeast paraprobiotic, gut health and pathogen binding
The intestinal barrier is made up of a layer of epithelial cells connected to each other by intercellular junctional complexes called tight junctions. The gut lining is covered by a mucus layer, which stimulates a better uptake of nutrients but also prevents the diffusion of unwanted substances. It was demonstrated that heat stress hampers the development of the intestinal morphology and function. In poultry, dietary supplementation with the yeast paraprobiotic increases goblet cell number and mucus thickness of the jejunal mucosa (Morales-Lopez et al., 2010) (Table 1). As a result, it may induce better gut morphology when birds are subjected to heat stress. Additionally, it has been confirmed that the selected yeast fraction can bind various pathogenic bacteria, making it difficult for them to colonize the intestine and to get in contact with the intestinal epithelial cells (Posadas et al., 2017) (Fig. 2).
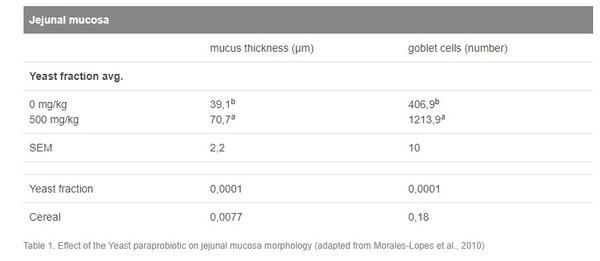
Table 1. Effect of the Yeast paraprobiotic on jejunal mucosa morphology (adapted from Morales-Lopes et al., 2010)
Figure 2. SEM images of pathogenic bacteria E. coli (A) and S. Typhimurium (B) bound to the yeast paraprobiotic. (Adapted from Posadas et al., 2017)
This leads to the hypothesis that the selected yeast fraction could counteract the negative effects of heat stress on the epithelial barrier by reducing the translocation of bacterial products, and limit the production of pro-inflammatory cytokines like IL-6 which may activate the HPA-axis (Fig. 3).
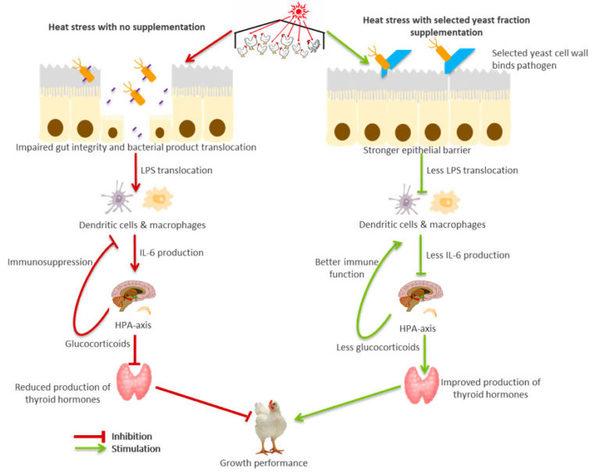
Figure 3. The selected yeast fraction mitigates detrimental effects of heat stress by binding pathogens and strengthening gut integrity
Yeast paraprobiotic and immunomodulation
Although a direct action of the yeast paraprobiotic on the HPA-axis to influence heat stress is excluded, an indirect modulation of the axis through the immune system could be postulated. It is well known that β-glucans and mannanoligosaccharides modulate the immune function. For example, research has shown that dietary addition of yeast products to broiler feed improves phagocyte activation as serum lysozyme content increased. Therefore, it was suggested that these yeast products would intensify the non-specific immunity in birds. Additionally, it has been demonstrated that yeast fraction is able to stimulate the humoral immunity as antibody titers to Newcastle Disease vaccination increase when birds are supplemented with yeast fraction (Gao et al., 2008). Furthermore, it was shown that yeast fraction supplementation decreases the physiological and immunological acute phase response in newly-received heifers following an endotoxin challenge i.e. vaginal temperatures were significantly lower in the yeast fraction supplemented groups while also cortisol and IL-6 significantly lowered in supplemented heifers (Burdick Sanchez et. al., 2013). It can be hypothesized that also in poultry species, when exposed to high environmental temperatures, selected yeast fraction is able to influence the acute phase response leading to lower amount of IL-6 production. As such the HPA-axis would become less activated with decreased production of corticosterone in the blood flow avoiding unwanted immunosuppression.
Conclusion
Safmannan® supplementation has an indirect role on the HPA-axis by improving the gut health of the animal. A strengthened gut epithelial barrier and an adequate pathogen binding will diminish the contact of bacteria with the immune system and decrease the production of IL-6. This induces better bird’s resistance towards acute and chronic heat stress.
Safmannan® yeast paraprobiotic has shown to alleviate the negative effects of heat stress.