ABSTRACT
Coproducts of n-3 fatty acid production from algae are a potential feed resource for cattle. A study was conducted evaluating feeding algal biomass to cattle. Crossbred cattle (20 steers and 20 heifers, 255 kg initial BW, SD = 14) were individually fed 4 inclusions of condensed algal residue solubles (CARS; 0, 2.5, 5, 7.5% of diet DM) displacing dry-rolled corn in the finishing diet for a minimum of 97 d. Increasing CARS inclusion in the diet quadratically increased DMI and ADG (P ≤ 0.01) and linearly increased G:F (P < 0.01), NEm, and NEg. Out of 27 organs measured, 6 had differences due to treatment in absolute weight and weight as a percentage of BW. Weight of the liver, pancreas, jejunum, and heart linearly increased (P ≤ 0.05) and weight of the thyroid and gall bladder quadratically increased (P ≤ 0.04) as CARS inclusion in the diet increased. However, organ weights were all within expected ranges, and histopathology analysis of organs revealed no differences due to treatment (P ≥ 0.24). Hemoglobin and hematocrit concentrations quadratically decreased (P = 0.05) and red blood cell distribution width linearly increased (P = 0.02) as CARS inclusion increased; no other differences were observed for hematology measures (P ≥ 0.11). Out of 21 blood chemistry measures, 8 were affected by treatment (P ≤ 0.02). Inclusion of up to 7.5% of diet DM as CARS had no adverse effect on cattle and improved performance when fed up to 5.0% of the diet DM.
Key words: algae, cattle, coproduct, feed safety, novel feedstuffs.
INTRODUCTION
With increasing interest in production of algae-derived n-3 fatty acids for both human food and animal feeds, coproducts from the algae industry could result in an alternative feed ingredient for cattle. Algal biomass is a potential source of protein, fiber, and fat, which could contribute essential nutrients in cattle diets. A condensed algal residue solubles (CARS; Veraferm, Veramaris, Delft, the Netherlands) product is being commercially produced from heterotrophic algae as a result of producing n-3 fatty acids for use in the animal feed industry, primarily aquaculture and pet foods. The CARS is produced by condensing the residue from algal fermentation of dextrose after the oil has been extracted from the algal cells without organic solvents and has a syrupy consistency.
Marine algae, commonly photoautotrophic, have been used in animal diets for many years (Lum et al., 2013). Heterotrophic algae, grown using complex organic substances for feedstuffs, may result in improved yields and growth efficiency, improving the economics of using algae as a livestock feedstuff (Ogbonna et al., 1997; Bryant et al., 2012). Van Emon et al. (2015) fed a heterotrophic microalgae meal (57% microalgae, 43% soyhulls) to growing cattle. They observed greater DMI and decreased G:F as algae meal increased from 0 to 45% of diet DM, replacing wet corn gluten feed. A similar algae meal (43% partially deoiled heterotrophic microalgae and 57% soyhulls) was fed to finishing cattle (Stokes et al., 2016) replacing corn at 0 to 42% of diet DM. They reported no change in HCW and a linear decrease in calculated dietary NEg content as inclusion increased in the diet. Morrill et al. (2017) supplemented residue from microalgae to finishing cattle in the last 35 d of the feeding period and observed an increase in DMI, no differences in HCW, and greater marbling scores. Little research has been conducted on algae as a feed ingredient, and no research has been conducted feeding CARS to cattle; therefore, the objectives of this study were to evaluate the safety of CARS as a feed ingredient in cattle diets and the performance response to increasing inclusion in the diet of finishing cattle.
MATERIALS AND METHODS
The experiment was conducted at the Eastern Nebraska Research and Extension Center (near Mead, NE), University of Nebraska Animal Science Complex (Lincoln, NE), and the University of Nebraska Veterinary Diagnostic Center (UNL VDC; Lincoln, NE). Animal handling and space for the experiment were in accordance with the Guide for the Care and Use of Agricultural Animals in Agricultural Research and Teaching (FASS, 2010). All procedures outlined as part of this study were approved by the University of Nebraska–Lincoln Institutional Animal Care and Use Committee (protocol number 1517). Because CARS is not currently approved by the FDA to be fed to cattle entering the human food chain, all tissues and carcasses were incinerated at completion of the experiment, following intensive sampling of tissues.
Experimental Design
A trial was conducted using 40 crossbred cattle (20 steers and 20 heifers, 255 kg initial BW, SD = 14). At receiving, all cattle were vaccinated with a Mannheimia haemolytica, bovine rhinotracheitis virus, bovine viral diarrhea (type 1 and 2), parainfluenza-3, and bovine respiratory syncytial virus combination vaccine (Bovi-shield One Shot, Zoetis, Florham Park, NJ); received a bacterin toxoid against 7 clostridial diseases and Haemophilus somnus (Ultrabac-7, Zoetis); received an intranasal vaccine containing bovine rhinotracheitis, parainfluenza-3, and bovine respiratory syncytial virus (Inforce 3, Zoetis); were dewormed with 1% wt/vol doramectin (10 mg/mL, Dectomax, Zoetis); and received 10 mL of gamma-cyhalothrin pour-on (StandGuard, Elanco, Greenfield, IN) for control of external parasites. Cattle were tagged with one 4-digit panel tag, a metal clip with the same 4-digit identification, and an electronic ID. All cattle were individually fed using the Calan gate system (American Calan Inc., Northwood, NH). All cattle were housed within 2 pens (one for steers and one for heifers). All treatments were equally represented in each pen (5 replications per pen) for a total of 20 animals per pen. The calves underwent a 3-wk training period to acclimate to the Calan gate system before trial initiation. Each animal had approximately 46 linear cm of bunk space. Daily observations of each individual animal were recorded after feeding by trained animal care staff at the research facility; daily observation forms were kept on record.
Five days before the initiation of the trial, cattle were limit fed at 2% of BW a common diet of 50% Sweet Bran (Cargill corn milling, Blair, NE) and 50% alfalfa hay (DM basis; Watson et al., 2013). Cattle were weighed on 3 consecutive days before feeding to reduce error from gut fill, and the average was used as initial BW. Weights from days 1 and 2 were averaged, and cattle were blocked by initial BW strata into 10 blocks where blocks 1, 3, 5, 7, and 9 represented the heaviest to lightest steers and blocks 2, 4, 6, 8, and 10 represented the heaviest to lightest heifers with each treatment being represented in each block. On the third day of weighing, cattle were additionally ear tagged with the corresponding bunk ID number.
Four dietary treatments were assigned randomly to animal within block. Diets consisted of increasing inclusion of CARS (0, 2.5, 5, and 7.5% of diet DM; Table 1) displacing dry-rolled corn in the diet (70.0, 67.5, 65.0, and 62.5%). All diets contained 15% wet distillers grains, 10% grass hay, and 5% supplement (DM basis). Because of the high Na content of CARS (Table 2; 8.5% of DM), 2 supplements were formulated, one for the 0% CARS and another for the 7.5% CARS treatment. Both supplements were blended together for use in the 2.5% and 5% CARS diets. Supplements were formulated to limit dietary Na to 1% of diet DM. Supplements included limestone, urea, trace mineral premix, vitamin ADE premix, tallow, monensin (330 mg per animal daily; Rumensin, Elanco Animal Health), and tylosin (90 mg per animal daily; Tylan, Elanco Animal Health) with fine-ground corn as the carrier. Cattle were fed once daily (0700 h) to provide ad libitum access to feed.
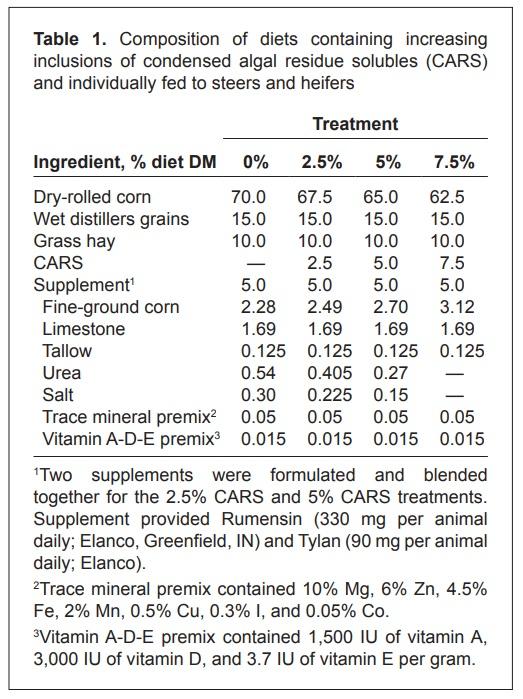
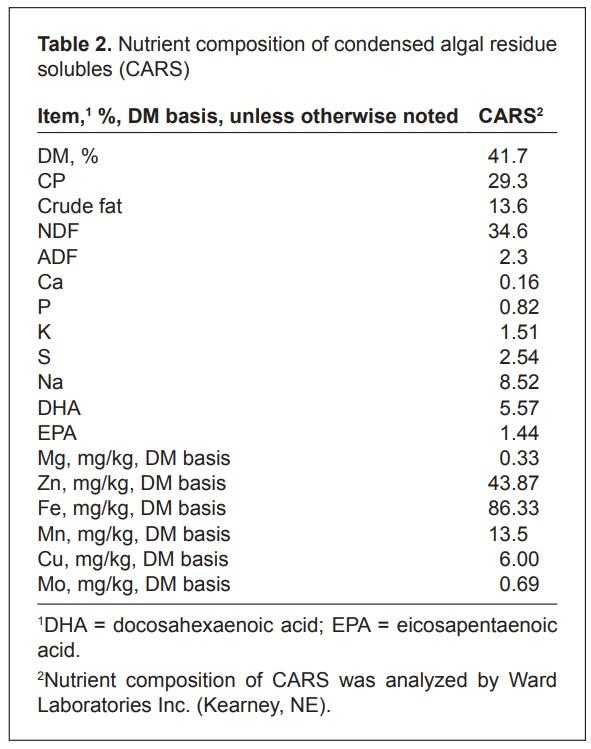
Feed refusals were collected weekly, weighed, and then dried in a 60°C forced-air oven for 48 h to calculate accurate DMI per individual. Approximately 400 g of each TMR and individual ingredients (CARS, dry-rolled corn, wet distillers grains, grass hay, and supplement) were sampled weekly. Samples were composited into 3-wk periods (6 composites of each of 4 diets and each ingredient) and subsequently analyzed for DM, OM, NDF, ADF, CP, macro- and micro-minerals (Ward Laboratories Inc., Kearney, NE), docosahexaenoic acid, and eicosapentaenoic acid (Eurofins Scientific, Des Moines, IA; Table 3). The docosahexaenoic acid and eicosapentaenoic acid levels in diets were used to confirm dosage of CARS because CARS was the only source of docosahexaenoic acid and eicosapentaenoic acid in the diets. Net energy calculations were calculated by the quadratic solution described by Zinn et al. (2003) with modifications developed by Vasconcelos and Galyean (2008).
Blood and Urine Analysis
Interim BW, urine, blood, and physical observations by a trained veterinarian were obtained on d 0, 33, 61, and 90 and on slaughter day. On each collection day, cattle were processed through a chute, weighed, and visually appraised by a veterinarian. Veterinary observations were characterized as normal or abnormal and included behavior and general appearance, special senses (eyes, ears, nose, and mouth), integument (skin and hair), respiratory, gastrointestinal, musculoskeletal and feet, cardiovascular, urogenital, and neurological. Cattle were then dosed with furosemide (2 mL/45 kg of BW, Lasix, Validus Pharmaceuticals LLC, Parsippany, NJ), a diuretic, to stimulate urination. A 50-mL conical tube was used to capture a urine sample. Urine was chilled during collection, and samples were immediately transported to the UNL VDC (Lincoln, NE) for urinalysis including protein, pH, ketone bodies, bilirubin, urobilinogen glucose (Chemstrip 2 GP, 2 LN, 9, 10 with SG, Roche Diagnostics, Indianapolis, IN), and microscopic examination. Samples of blood were collected by jugular venipuncture with 2 Vacuette Tubes that were 6 mL with potassium EDTA (Greiner Bio-One GmbH, Monroe, NC) as an anticoagulant and 2 Corvac Integrated Serum Separator Tubes (Covidien, Mansfield, MA) per animal. Blood samples were chilled following collection and immediately transported to the UNL Ruminant Nutrition laboratory (Lincoln, NE). At the laboratory, blood serum tube samples were placed in a 4°C refrigerator for 1 h before centrifugation at 1,250 × g for 10 min at 4°C. Whole blood and blood serum samples were sent to Iowa State University Veterinary Pathology Laboratory (Ames, IA) overnight for common hematology and blood chemistry. Hematology included white blood cell count (WBC); red blood cell count; mean corpuscular volume; mean corpuscular hemoglobin; mean corpuscular hemoglobin concentration; red blood cell distribution width; mean platelet volume; platelet count; and neutrophil, lymphocyte, monocyte, eosinophil, basophil, plasma protein, fibrinogen, hematocrit, and hemoglobin concentrations. Blood chemistry measures included Na, K, Cl, Ca, P, Mg, BUN, creatinine, glucose, total protein, albumin, alanine aminotransferase, aspartate aminotransferase, alkaline phosphatase, gamma glutamyl transpeptidase, lactate dehydrogenase (LDH), creatine kinase, total bile acids, bicarbonate, and cholesterol.
Organ Harvest
Blocks were slaughtered at a target BW of 454 kg (419 ± 22 kg), with blocks 1 and 2 on d 97, 3 and 4 on d 104, 5 and 6 on d 111, 7 and 8 on d 118, and 9 and 10 on d 125. On each slaughter day, all cattle were individually weighed at 0630 h at the Eastern Nebraska Research and Extension Center before feeding. The 8 cattle to be slaughtered that day had blood samples from the jugular vein taken while in the chute and were then held in a sort pen. Remaining cattle were also weighed and then returned to their pen. Veterinary observations (same as previously described) were recorded on all animals. The 8 sorted animals were then transported to the University of Nebraska Animal Science complex (Lincoln, NE), where they were held in two 3.6 × 6 m pens (steers separate from heifers) with access to water. Cattle were transported by trailer from the Animal Science complex to the UNL VDC in groups of 2 for slaughter. Slaughter order was assigned randomly within block to avoid bias of timing of euthanasia. Steers were slaughtered before heifers. The cattle were injected with pentobarbital sodium (390 mg/mL, 2.2 mL/100 kg of BW, Fatal-Plus, Vortech Pharmaceuticals, Dearborn, MI) to euthanize the animal and exsanguinated.
A pathologist, blinded to treatment, supervised the necropsy and recorded gross findings. Feet were removed at the knee and the hock. The head was removed at the atlas, and the hide was skinned away from the thoracic cavity. Urine collection was done postmortem by needle and syringe directly from the bladder. After evisceration, the rest of the hide was removed. Organs were isolated, removed, washed, weighed, and then sampled in duplicate (approximately 10 g per sample). Organs and tissues evaluated included brain, spinal cord (2 segments), spleen, lung, pancreas, skeletal muscle, rumen reticulum, omasum, abomasum, duodenum, jejunum, cecum, colon, kidneys, urinary bladder, pituitary, thyroid, adrenal, liver, gall bladder, heart, mesenteric lymph node, skin, prostate, eye, bone and marrow, marrow smear, ileum, and thymus. For heifers, the ovaries, mammary gland, and uterus were also evaluated. After full tissue collection and necropsy, the cattle were incinerated at the UNL VDC.
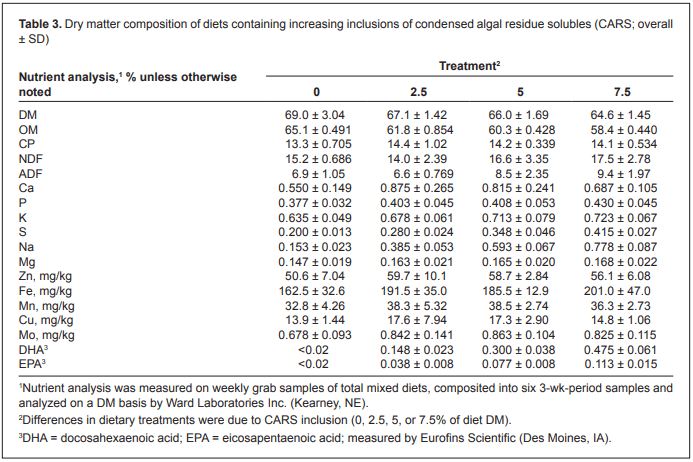
Due to mechanical failures with the rail and hoist system on the first slaughter day, block 2 heifers (4 animals) were held overnight at the University of Nebraska Animal Science complex. The heifers were individually penned, allowed access to water, and fed their respective treatment diet. The heifers were then slaughtered the next day following the same procedures. The remaining slaughter dates proceeded as planned with 8 animals slaughtered per day.
Preliminary Murine Experiments
Before the cattle feeding study, the safety of this novel feed ingredient was evaluated using a bacterial reverse mutation assay (Ames test) and an in vivo micronucleus test in mouse immature erythrocytes as well as repeated-dose toxicity studies rats. All studies were conducted by Eurofins Product Safety Laboratories (Dayton, NJ) in accordance with the GLP Regulations issued by the US Food and Drug Administration (Title 21 of the CFR, Part 58; effective 1987) and followed the Organization for Economic Co-operation and Development Guidelines for the Testing of Chemicals and Food Ingredients, Section 4, Parts 471, 474, and 408 (USDA, 2016)
In the Ames test (Ames et al., 1973), CARS was investigated for its potential to induce gene mutations according to the plate incorporation test using Salmonella Typhimurium strains TA 98, TA 100, TA 1535, and TA 1537 and tester strain Escherichia coli WP2 uvrA. No toxic effects of the test item were noted in any of the 5 tester strains. No biologically relevant increases in revertant colony numbers were observed following treatment with CARS at any concentration level, neither in the presence nor absence of metabolic activation in both experiments, indicating lack of mutagenic potential of CARS.
The safety of CARS was also evaluated in a 14-d dietary toxicity study in rats followed by a subchronic 90-d dietary study in Sprague–Dawley rats. In the 90-d study (Organization for Economic Co-operation and Development Test Guideline 474; OECD, 2014), the test material was added to the basal diet at dietary levels of 0.5% (5,000 ppm), 1.5% (15,000 ppm), and 5.0% (50,000 ppm). There were no changes in BW, BW gain, feed consumption, or feed efficiency in male and female rats attributable to the administration of test substance. There were no test substance–related changes in hematology, coagulation, clinical chemistry, and urinalysis parameters. There were no CARS-related macroscopic or organ weight changes. Test substance–related microscopic findings consisted of pancreatic acinar cell hyperplasia observed in high-dose (50,000 ppm) males (found in 3 out of 10 animals).
Therefore, under the conditions of the study and based on the toxicological endpoints evaluated, the No-AdverseEffect Level for administration of CARS in the rodent diet was determined to be 1.5% of the diet (15,000 ppm), equivalent to an overall average CARS intake of 1,071 mg/ kg of BW daily for male and female rats. These preliminary experiments were completed before the current cattle feeding trial and suggested no toxic effects of CARS.
Statistical Analyses
Performance data (BW, ADG, DMI, G:F, HCW, dietary NEm, and NEg , and organ weights) were analyzed using the mixed procedure of SAS (SAS Institute Inc., Cary, NC) as a randomized complete block design with treatment, sex, and treatment-by-sex interactions as fixed effects, BW block as a random effect, and individual animal as the experimental unit. Interactions were removed from the model if not significant. Orthogonal contrasts were used to test significance for linear, quadratic, and cubic responses due to CARS inclusion. Blood and urine data were analyzed as repeated measures with an optimized covariate structure selected based on the lowest Akaike information criterion score suggesting the best model fit (Littell et al., 1998). For a few variables measured in the urine (epithelial cells, amorphous crystals, triple phosphate crystals, WBC, blood, protein, anisocytosis, acanthocyte, and echinocyte) qualitative data were collected and then transposed to numerals for analysis (0 = none, 1 = few, 2 = moderate, 3 = many). Probabilities less than or equal to 0.05 were considered significant; values less than or equal to 0.10 were declared tendencies.
RESULTS AND DISCUSSION
Cattle Performance
There were no interactions between sex and treatment (P ≥ 0.25) for performance data. Sex was significant for all variables (P ≤ 0.04), with steers having greater DMI, initial BW, ADG, HCW, and final BW compared with heifers. There were no differences in initial BW between CARS treatments (P ≥ 0.27). There was a quadratic response (P = 0.01; Table 4) observed for DMI, with cattle fed 2.5% CARS having the greatest DMI of 8.98 kg/d. There was a quadratic (P < 0.01) response for ADG, with cattle fed 2.5% and 5% CARS having the greatest numerical values of 1.40 and 1.37 kg, respectively. Live final BW responded quadratically (P < 0.01) and was the greatest for cattle fed 2.5% and 5% CARS, 428 and 427 kg, respectively. The cattle fed 7.5% CARS had the lowest DMI and ADG (P ≤ 0.01); however, this treatment elicited a greater G:F of 0.186, linearly (P < 0.01) increasing with increased algae inclusion in the diet. Both calculated NEm and NEg linearly increased (P < 0.01) with increasing inclusion of CARS. Cattle fed diets containing 2.5, 5, or 7.5% CARS had 4.2, 11.4, and 12.0% increases in G:F relative to the corn control diet. Improved performance is likely due to the CP and fat content of the CARS, although this has not been measured. A simple economic comparison using a diet cost of $0.15/kg for all treatments (assuming cost of CARS is equal to the corn it was replacing in the diet) shows that cost of gain (cost of total feed divided by total weight gained) linearly decreased (P < 0.01) from $1.01/kg for the 0% CARS to $0.91/kg for the 7.5% CARS treatment.
The CARS evaluated in this trial differs from other algal-based feedstuffs fed to cattle and evaluated in previous research (Franklin et al., 1999; Drewery et al., 2014; Van Emon et al., 2015; Costa et al., 2016; Stokes et al., 2016). The nutrient profile is unique due to both the initial algae feedstock and the processing methods of CARS production. Much of the previous research has also fed the algal residue in combination with other feeds, such as soyhulls (Van Emon et al., 2015; Stokes et al., 2016), to growing cattle (Drewery et al., 2014; Van Emon et al., 2015; Costa et al., 2016) or as a supplement at the end of a finishing period (Morrill et al., 2017). In a trial with finishing cattle, a meal consisting of 43% partially deoiled microalgal residue and 57% soyhulls replaced up to 42% of the dietary dry-rolled corn (Stokes et al., 2016). Authors reported no differences in final BW or ADG but a linear decrease in G:F as the algal meal replaced corn in the diet. This resulted in a linear decrease in both dietary ME and NEg as algal meal inclusion increased. Results from the current trial suggest feeding algal residue up to 7.5% of dietary DM linearly increased G:F and dietary NEg . This would be a similar algae inclusion as the lowest inclusion of algae meal (14% diet DM) in the trial by Stokes et al. (2016). Morrill et al. (2017) supplemented an algal residue to finishing diets and reported lower NEm and NEg than measured in this trial, 1.54 and 0.94 Mcal/kg, respectively. Authors reported an increase in DMI and no differences in HCW compared with infusion of 1.0 kg of glucose daily. Algal residues are somewhat variable depending on the species grown and the manufacturing process used for production. The CARS product evaluated in the current trial appears to be a suitable replacement for corn in finishing diets, up to 7.5% of diet DM, and improved ADG up to 5% inclusion of the diet DM.
Organ Weights
Organ weights were analyzed as absolute organ weight as well as organ weight as a percentage of shrunk BW (SBW, final BW shrunk 4% to account for gut fill). Table 5 reports organ weights with the sex × treatment interaction removed from the statistical model for all organs. There were no significant differences (P ≥ 0.16) among treatments for organ weight of spleen, lungs, rumen, reticulum, omasum, ileum, cecum, kidneys, pituitary, adrenal, eye, thymus, uterus, ovaries, prostate, and seminal vesicle. Pancreas weight linearly increased (P = 0.02) as CARS inclusion increased in the diet; however, this could be attributed to the difficulty of distinguishing pancreas and fat connected to the pancreas. There was a quadratic response observed for brain weight (P = 0.04); cattle fed 5% CARS had the greatest brain weight of 387 g. Liver weight linearly increased (P < 0.01) as CARS inclusion increased in the diet. Thyroid weight had a quadratic response (P = 0.02), with cattle fed 2.5% CARS having the greatest weight of 31.8 g. There was a quadratic (P = 0.04) response for abomasum weight, with cattle fed 0% CARS having the lightest weight of 1.25 kg and cattle fed 5% CARS having the greatest weight of 1.41 kg. Similarly, there was a quadratic response (P = 0.03) for duodenum weight, with cattle fed 0% CARS having the lightest weight of 273 g and cattle fed 5% CARS having the greatest weight of 326 g. The difference in duodenum weight between treatments could be attributed to variation in discretion of where the duodenum ends and the jejunum begins. There was a cubic response observed for urinary bladder weight, with cattle fed 2.5% CARS having the greatest weight of 116 g and cattle fed 5% CARS having the smallest weight of 96.4 g. Differences in urinary bladder weight were small, and the cubic response suggests differences were due to variation and error in measurement, not biological differences due to treatment.
There was a tendency for a sex × treatment interaction (P = 0.08; Table 6) for jejunum weight, with steers fed 7.5% CARS having the greatest weight of 6.33 kg and heifers fed 5% CARS having the greatest weight of 5.69 kg. There was a sex × treatment interaction (P = 0.02) for gall bladder weight, with a quadratic (P < 0.01) response. Steers fed 2.5% CARS had the greatest weight of 81.6 g, and heifers fed 5% CARS had the greatest weight of 107 g. There was a sex × treatment interaction (P = 0.04) for heart weight, with steers fed 7.5% CARS having the greatest heart weight (2.21 kg) and heifers on the 5% CARS treatment having the greatest heart weight (2.07 kg). There was a sex × treatment interaction (P= 0.02) for colon weight, with steers fed 7.5% CARS having the greatest colon weight (4.38 kg) and heifers fed 2.5% CARS having the greatest colon weight (4.93 kg).
Organ Weight as a Percentage of SBW
There were no significant differences (P ≥ 0.07) among treatments in organ weight as a percentage of SBW for spleen, lungs, rumen, reticulum, omasum, abomasum, duodenum, ileum, cecum, kidneys, urinary bladder, brain, pituitary, adrenal, thymus, prostate, seminal vesicles, uterus, ovaries, and colon. A difference in liver weight as a percentage of SBW was observed, with a quadratic response (P < 0.01); cattle fed 7.5% CARS had the greatest weight (2.05 kg). There was a quadratic response (P = 0.04) for thyroid weight as a percentage of SBW, but differences due to treatment were small, varying from 0.006 to 0.008% of SBW. The weight of both the pancreas and eye linearly (P ≤ 0.01) increased as CARS inclusion increased in the diet.
There was a sex × treatment interaction (Table 6, P = 0.04) for jejunum weight as a percentage of SBW, which linearly (P < 0.01) increased as CARS increased in the diet. There was a sex × treatment interaction (P= 0.04) in colon weight, with steers fed 2.5% and 5% CARS having the smallest colon and an increase in colon weight for heifers fed 2.5% and 5% CARS (P ≤ 0.04). There was a tendency (P = 0.07) for colon weight as a percentage of SBW to be greater in heifers than steers. There was a sex × treatment interaction (P = 0.01) in gall bladder weight as a percentage of SBW, with steers fed 2.5% CARS having the greatest gall bladder weight and heifers fed 5% CARS having the greatest gall bladder weight. There was a sex × treatment interaction (P = 0.03) for heart weight as a percentage of SBW. Heart weight linearly increased (P = 0.01) from 0.444 to 0.554% of SBW in steers and from 0.454 to 0.515% in heifers as inclusion of CARS increased in the diet.
Absolute organ weights and organ weights as a percentage of SBW are similar to values published in the literature (Hersom et al., 2004; McCurdy et al., 2010). Differences due to CARS inclusion were relatively minor and likely due to nutrient load (Hammond and Janes, 1998; Meyer et al., 2015). Differences in liver, pancreas, and gall bladder weights between treatments were the most pronounced. These organs function in nutrient digestion and excretion of nutrients. With increasing inclusion of CARS, some minerals, primarily Na, were increased in the diet and would have been processed by the liver and the kidney. Principal function of the kidney is regulation of salt and water balance. Common bovine diets induce a slightly higher blood pH. Diets with a DCAD of greater than zero will result in slight metabolic acidosis and alkaline urine (Bain, 2003; George and Zabolotzky, 2011).
Hematology
Both hemoglobin and hematocrit concentrations quadratically decreased (P = 0.05) with increasing inclusion of CARS (Table 7). For both measures, minimum concentrations were observed for cattle fed 2.5% CARS. Red blood cell distribution width linearly increased (P = 0.02) from 20.9 to 22.0% with increasing inclusion of CARS. There was a linear tendency (P = 0.09) for monocyte concentrations to increase as CARS inclusion in the diet increased, but all treatments fell within the expected laboratory reference range. There was no difference due to sex (P = 0.80) and no treatment × sex interaction (P= 0.48) for monocyte concentrations.
Sex was not significant (P ≥ 0.16), and there were no treatment × sex interactions (P ≥ 0.42) for WBC; red blood cells; hemoglobin; hematocrit; mean corpuscular hemoglobin concentration; red blood cell distribution width; platelet count; mean platelet volume; lymphocyte, eosinophil, basophil counts; and fibrinogen concentrations (data not shown). Sex was significant (P = 0.02) for mean corpuscular volume, with heifers having an average volume of 40.8 fL, and steers having an average volume of 38.6 fL, but no treatment × sex interaction (P = 0.38) was observed. Sex was significant (P = 0.02) for neutrophil concentrations, with heifers having greater concentration of neutrophils at 3.57 × 103 /ul and steers having a concentration at 2.84 × 103 /ul, but there were no differences between treatments (P = 0.18). There was a difference due to sex (P = 0.02) in the concentration of plasma protein with heifers having a concentration of 8.36 g/100 mL and steers having a concentration of 8.09 g/100 mL, and there was tendency for a treatment × sex interaction (P = 0.08), but no differences among treatments (P = 0.11).
Laboratory reference ranges of hematology variables measured in cattle are presented in Table 7 as expected ranges (Veterinary Pathology, 2011). Almost all variables were within the prescribed expected range. The red blood cell distribution width was greater than expected, averaging 21.4% for all treatments with 8.0 to 15% considered the expected range. Fibrinogen concentrations were slightly elevated above the laboratory reference range for cattle fed 0% and 2.5% CARS at 516 and 582 mg/100 mL, respectively. The maximum upper limit of the laboratory reference range is 500 mg/100 mL. The mean corpuscular volume value for cattle fed 2.5% CARS was slightly lower than expected at 38.9 fL, with the lower end of the expected range at 40.0 fL. The mean platelet volume of cattle fed 7.5% CARS was greater than expected at 8.27 fL, with the upper end of the expected range at 8.0 fL. Plasma protein concentrations of all treatments were greater than expected, averaging 8.22 g/100 mL, with the upper end of the expected range at 7.7 g/100 mL. These expected ranges may have been established using different animal populations that may not be representative of normal feedlot animals on a finishing diet. Daily cattle observations and visual health observations all suggested cattle were healthy and showed no adverse effects to any dietary treatment.
Blood Chemistry
There were no differences due to sex (P ≥ 0.11), no treatment × sex interactions (P ≥ 0.29), and no differences among treatments (P ≥ 0.10) observed for blood Na, blood K, blood P, blood Ca, BUN, blood glucose, total bile acids, and aspartate aminotransferase concentrations. There was a tendency for a linear decrease (Table 8; P = 0.06) in aminotransferase concentration as CARS inclusion increased. There were no treatment × sex interactions (P = 0.46) and no differences due to sex (P = 0.47) for aminotransferase concentration. There was a linear decrease (P ≤ 0.01) in blood Cl concentration as CARS increased in the diet and a difference due to sex (P ≤ 0.01), with heifers having a concentration of 101 mEq/L and steers having a concentration of 100 mEq/L (SEM = 0.204). There were no treatment × sex interactions (P = 0.45) for Cl concentration, and blood Cl concentrations were within the expected ranges for cattle. There was a linear increase (P < 0.01) in blood bicarbonate concentration as CARS increased in the diet and a difference due to sex (P = 0.03), with heifers having a lower concentration than steers, 27.7 and 28.5 mEq/L, respectively. There were no treatment × sex interactions (P = 0.55) for blood bicarbonate concentration, and measured values were within the expected ranges for cattle. There was a cubic response (P = 0.03) for blood Mg, with cattle fed 5% CARS having the highest blood Mg concentration of 2.07 mg/100 mL. There was no difference due to sex (P= 0.11) and no treatment × sex interaction (P = 0.50) for blood Mg concentration. Stokes et al. (2016) reported no differences due to algal-meal inclusion in the diet on plasma Mg levels; values they reported are similar to the current trial averaging 2.36 mg/100 mL. There was a tendency for a cubic response (P = 0.09) for blood albumin concentrations, with cattle fed 5% CARS having the greatest concentrations of 3.27 g/100 mL; all treatments were within the expected range for cattle. Blood creatinine concentration linearly increased (P < 0.01) from 1.07 to 1.16 mg/100 mL as CARS inclusion increased in the diet. There was a tendency for a treatment × sex interaction (P = 0.09) in total protein concentration; however, there were no differences among treatments (P ≥ 0.10) and measured values were within the expected range for cattle. Sex was not significant (P = 0.50) for blood creatine kinase concentrations. However, there was a tendency for a treatment × sex interaction (P = 0.10), and a quadratic decrease (P = 0.02) was observed, with cattle fed 7.5% CARS having the greatest concentration of 217 IU/L. The creatine kinase concentration for all treatments was within the expected range for cattle. Alkaline phosphatase concentrations decreased linearly (P < 0.01) from 65.4 to 43.7 IU/L as CARS inclusion increased in the diet but were within the expected range for cattle. There was a tendency for a difference due to sex (P = 0.08) for gamma glutamyl transpeptidase, and a quadratic (P < 0.01) response was observed with cattle fed 0 and 7.5% CARS having the greatest concentrations of 46.8 and 45.1 IU/L, respectively. Total bilirubin concentration had a cubic response (P < 0.01), with cattle fed 5% CARS having the greatest concentration at 0.366 mg/100 mL. Sex was significant (P = 0.04) for total bilirubin, with heifers having a greater concentration at 0.351 mg/100 mL and steers at 0.323 mg/100 mL. All treatments had higher concentrations than would be expected for cattle, with the upper limit being 0.18 mg/100 mL. There was a tendency (P = 0.08) for steers and heifers to be different in total bile acids; steers had a concentration of 38.8 μmol/L and heifers 29.4 μmol/L. However, no differences among treatments existed (P ≥ 0.10). There was a tendency for a treatment × sex interaction (P = 0.09) in cholesterol, but there were no differences due to sex (P = 0.70). Cholesterol had a tendency to linearly increase (P = 0.07) as CARS inclusion in the diet increased. There was a difference due to sex (P = 0.02) for LDH levels; heifers had LDH levels of 4,390 IU/L and steers had levels of 4,120 IU/L. There was a quadratic (P = 0.04) response observed for LDH, with cattle fed 7.5% CARS having the greatest concentration of LDH at 4,494 IU/L, which is above the upper limit of the expected range, 410 IU/L. Feedlot cattle have large metabolic activity due to the high energy diets they are fed. This may lead to greater hepatocellular swelling and leakage, which is a primary source of LDH (Bain, 2003). Also, younger animals generally have greater levels of LDH. The expected range was developed from a mix of cattle, likely, cows on forage-based diets, because cattle on all treatments had elevated LDH concentrations relative to the expected range.
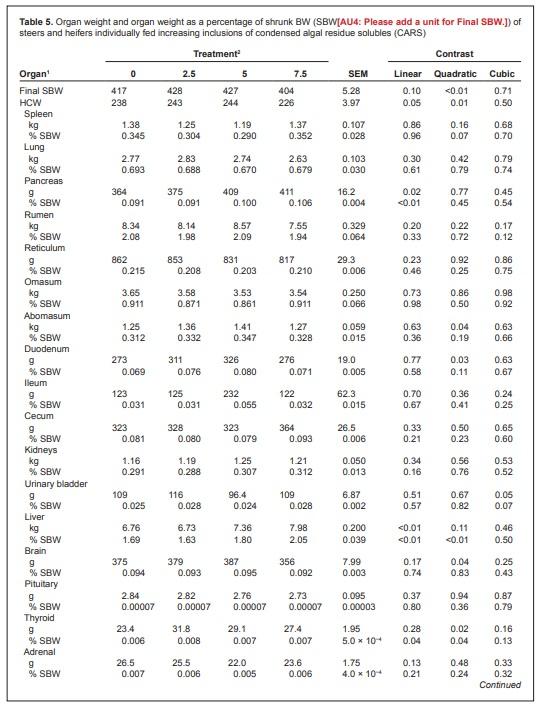
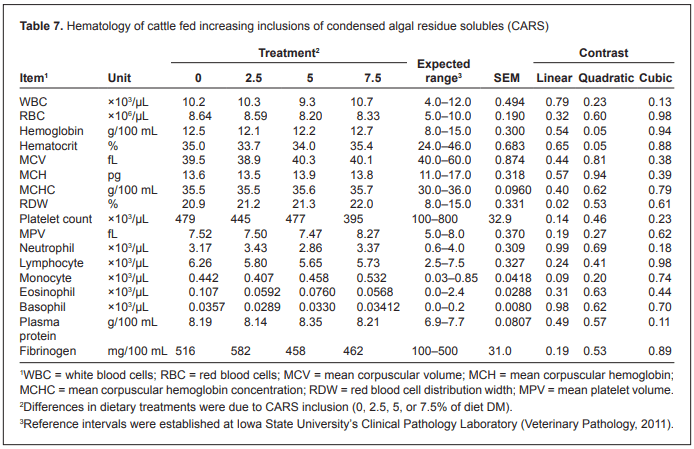
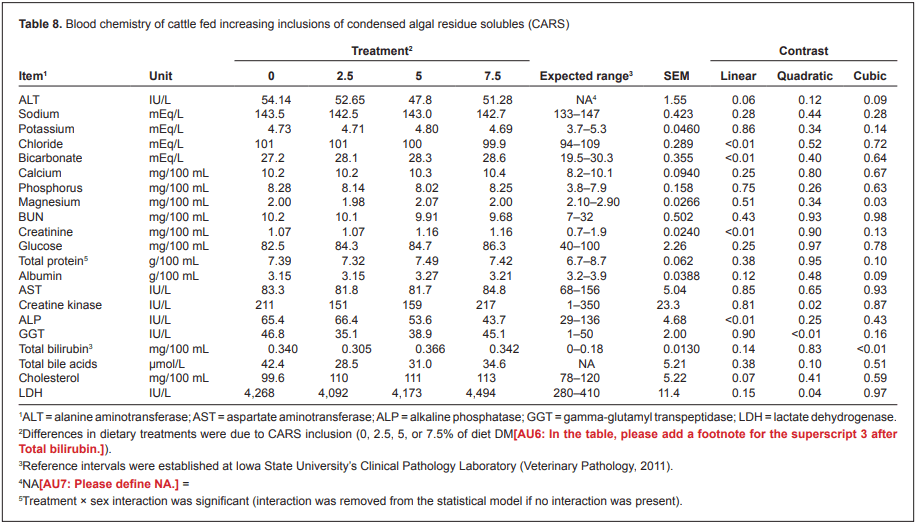
Laboratory reference ranges for blood chemistry variables measured in cattle are presented in Table 8 as expected ranges. Almost all variables were well within the prescribed expected range. However, these expected ranges may have been established using different animal populations that may not be representative of normal feedlot animals on a finishing diet. Total bilirubin was greater than expected, averaging 0.338 mg/100 mL, with the upper end of the expected range being 0.18 mg/100 mL. Blood concentrations of Ca and P were also greater than expected, averaging 10.3 and 8.17 mg/100 mL, with the upper expected limits of 10.1 and 7.9 mg/100 mL. Blood Mg concentration averaged 2.00 mg/100 mL, less than the expected value of 2.10 mg/100 mL. Daily cattle observations and visual health observations all suggested cattle were healthy and showed no adverse effects of any treatment.
Urine Analysis
Sex did not affect pH (P = 0.45) or specific gravity (P = 0.67) of urine. Urine pH did not have a treatment × sex interaction (P = 0.21), but there was a quadratic response (Table 9; P < 0.01) as CARS increased in the diet, with cattle fed 5% CARS having the greatest pH (8.70). There were no differences among treatments (P ≥ 0.69) for specific gravity.
There were no differences among treatments and no treatment × sex interactions (P ≥ 0.17) for epithelial cells, amorphous crystals, WBC, or protein or blood measured in the urinalysis. Epithelial cell count was quantified as few (1 to 10 cells per field) in all treatments. Amorphous crystals, WBC, and protein and blood were all quantified as none for all treatments. Triple phosphate crystals had a numerical difference of none for cattle fed 0% CARS and few (1 to 10 crystals per field) for cattle fed 2.5, 5, or 7.5% CARS, but there was no statistical difference between treatments (P = 0.10).
Histopathology
Two treatments (cattle fed 0% CARS and 7.5% CARS) were compared for all histopathology analysis. The pathologist was blinded to treatments, and slides from tissues of cattle fed 0 and 7.5% CARS were evaluated as either 0 = normal or 1 = abnormal. There were no significant differences due to treatment (P ≥ 0.24) for brain (5 slides evaluated), spinal cord (2), eye, spleen, left cranial lung, right caudal lung, pancreas, longissimus (skeletal muscle), brisket (skeletal muscle), rumen (3), reticulum, omasum, abomasum, duodenum, jejunum (3), cecum, ileum, thymus, colon (2), right kidney, left kidney, urinary bladder, pituitary, thyroid, adrenal, left liver, right liver, gall bladder, left side of heart, right side of heart, mesenteric lymph node (2), prostate, ovary (2), skin, hoof c band, hoof wall, hoof sole, and bone marrow. It was assumed that with no differences between the 2 extreme inclusions of CARS (0 vs 7.5%), the intermediate treatments were also not affected. Histology results from 0 and 7.5% CARS suggest that there were no differences in tissue health of the cattle whether CARS was included in the diet or not.
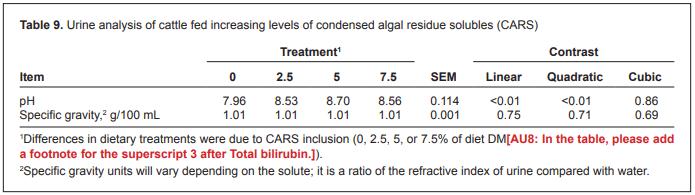
IMPLICATIONS
The feedstuff CARS demonstrated to be a safe and efficacious feed ingredient in cattle diets. Feeding CARS to finishing cattle improved G:F as inclusion in the diet increased up to 7.5% of diet DM. Cattle HCW, ADG, and DMI all increased quadratically and were maximized when cattle were fed 2.5 or 5% CARS. No adverse effects of feeding CARS were observed in hematology, blood chemistry, or histopathology analyses. An increase in organ weight was observed for the liver, thyroid, gall bladder, pancreas, jejunum, and heart when CARS was fed, but no effect on health was observed and no differences in tissues were found. Further research is needed to determine the optimal inclusion of CARS on performance and carcass traits when fed an entire feeding period, as well as potential for CARS to be used in growing cattle diets.
ACKNOWLEDGMENTS
Funding and products were provided by DSM Nutritional Products (Parsippany, NJ) and Evonik Industries (Hanau, Germany).