INTRODUCTION
Salmonella is a foodborne pathogen of great concern, and often, Salmonella outbreaks are found to originate from poultry (CDC, 2018; CDC, 2019). Every year in the United States, Salmonella is responsible for causing 1.35 million infections, >25,000 hospitalizations, and >400 deaths, and the majority of these illnesses are attributed to food (CDC, 2020). Salmonella's major risk factor in poultry meat and table eggs is its presence in live birds (Hugas and Beloeil, 2014). Poultry intestinal tracts colonized with Salmonella can potentially contaminate poultry meat during processing and cut-up, which could then result in foodborne salmonellosis in humans after consuming improperly cooked and/or handled contaminated product (Nayak et al., 2004). Salmonella is a commensal microorganism in poultry and has the potential to disseminate to the whole flock without any visible sign (Hugas and Beloeil, 2014). Salmonella can spread in poultry farms by both vertical and horizontal transmission. Potential sources for Salmonella spread within the poultry production chain include breeders, hatcheries, chicks, the poultry house environment, feed, insects, rodents, and wild birds (Liljebjelke et al., 2005).
Airborne transmission of Salmonella is an indirect horizontal method of spread and has previously been observed in several studies. Salmonella was transmitted from challenged hens in cages to nonchallenged molted hens when they were physically apart (1 m) from each other (Holt et al., 1998). Aerosolized S. Pullorum was demonstrated to infect one-day-old chicks (Cheng et al., 2020). Zhao et al. (2014) reported that on livestock farms, airborne microorganisms and dust have an interrelation indicating dust can carry microorganisms in the air, and the origin of dust and airborne microorganisms are usually from the same sources. Reduction of airborne dust concentration has been linked with a decrease in airborne bacteria (Mitchell et al., 2004). Moreover, it was reported that reducing airborne dust levels using negative air ionization may also help to limit the spread of S. Enteritidis in poultry flocks (Gast et al., 1999). Therefore, the identification of sources of microorganisms and dust in poultry houses can help to understand the mechanism of airborne transmission and to develop and implement new control strategies to prevent this transfer (Zhao et al., 2014).
Poultry house dust is comprised of feathers, skin debris, feed, litter, and feces, and all of these components may carry microorganisms (Madelin and Wathes, 1989). Occasionally, dust is also termed as an aerosol because of its ability to disperse in the environment and its fine particulate characteristics (Al Homidan et al., 2003). Litter is the main source for the large proportion of dust in floor housing systems (David et al., 2015). Similarly, broiler houses with litter have been associated with higher dust and airborne microorganism levels compared to litterless houses (Madelin and Wathes, 1989). Moreover, litter properties such as fresh bedding or used litter, as well as litter moisture levels, are also recognized to influence dust density and emission rate in tunnel-ventilated broiler poultry houses (Modini et al., 2010). Specifically, the increasing litter moisture content may reduce the dust emission; however, the higher litter moisture levels support adverse effects such as ammonia and odorant production and their emission in poultry facilities (Al Homidan et al., 2003; Ogink et al., 2012; Dunlop et al., 2016).
The presence of dust in poultry houses, its linkage with litter, and its capability to carry microorganisms in the air have been reported previously in the literature. However, the levels of Salmonella transfer to dust generated from contaminated litter and the effect of litter moisture levels on this transmission is still not well defined. Therefore, the objectives of this work were 1) To examine the potential for transfer of aerobic bacteria, Salmonella, E. coli, and coliforms to in vitro generated dust (settled or airborne) produced from poultry litter and 2) To examine the role of litter moisture content in litter to in vitro generated dust transfer of these specified microorganisms.
MATERIALS AND METHODS
Experimental Design
Experiment 1. To assess litter to air transfer of microorganisms, an in vitro method was developed. Approximately 3.5 kg of used litter was collected from one source (a single pen) to run all replications of this experiment. The litter collected for this experiment consisted of pine shavings that had hosted 2 previous broiler flocks and a third flock in the rearing stage. For each of 5 replications of this experiment, conducted on separate days, litter was mixed and then 4 batches of 110 g of litter were taken and individually delivered into 4 separate 2 L Erlenmeyer flasks. The fifth flask served as a negative (no litter) control and remained empty. Four flasks containing litter were separately inoculated with 10 mL of 4 different levels of a nalidixic acid-resistant strain of Salmonella Typhimurium. Four levels of inoculation were 102, 104, 106, or 108 CFU/mL for first replication and 103, 105, 107, or 109 CFU/mL for the subsequent replications. Salmonella inocula were applied in a dropwise manner onto the litter while simultaneously shaking the flask by hand. Dripping of Salmonella inoculums was done using transfer pipets (5 mL, VWR International, LLC, Radnor Corporate Center, PA). Following inoculation, each flask was covered with aluminum foil and acclimated for 24 h at room temperature. After 24 h, 20 g of litter from each flask was removed for litter moisture percentage determination and microbial analysis. The remaining litter (90 g) was used for in vitro dust generation that was further collected in buffered peptone water (BPW) (BBL, Becton Dickinson and Company, Sparks, MD) by using an impingement system (Figure 1). Following dust collection, BPW was used for microbial analysis. The total number of litter and dust samples used for microbial analyses were n = 20 and n = 25, respectively.
Figure 1. Schematic view of mechanism of impingement for Experiments 1 and 2.
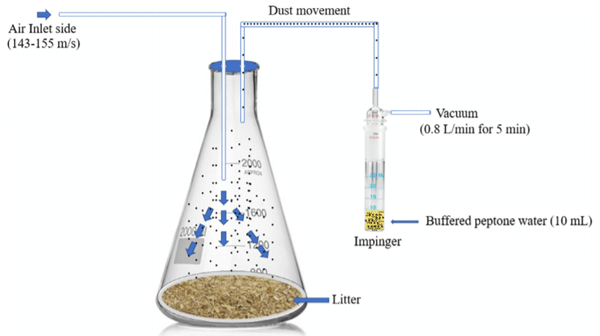
Experiment 2. The in vitro setup developed for Experiment 1 was used to assess litter moisture effect on the litter to air transfer of microorganisms. Approximately 3.5 kg of litter was gathered from one source (a single pen) to run all replications of this experiment. The litter collected for this experiment consisted of pine shavings that had a single broiler flock in rearing stage. For each of the 5 replications of this experiment, conducted on separate days, 5 batches of litter weighing 110 g were prepared and individually added to 5 separate 2 L flasks for each replication. The sixth flask did not contain litter (negative control). Four flasks containing litter were separately inoculated with 10 mL of a nalidixic acid-resistant strain of S. Typhimurium (109 CFU/mL). To adjust different litter moisture levels, increasing amounts of sterilized water (10 mL, 15 mL, and 20 mL) were added to 3 of the 4 inoculated flasks. The fifth flask containing litter was not inoculated. The flasks receiving dropwise inoculum or sterilized water were simultaneously shaken by hand. After inoculation or moisture addition, each flask was covered with aluminum foil and then held 24 h at room temperature. After 24 h, 20 g of litter from each flask was removed for litter moisture percentage determination and microbial analysis. The remaining litter (90 g) was used for dust generation that was further collected into BPW through the impingement system. Following impingement, collected dust samples in BPW were used for microbial analysis. The total number of litter and dust samples used for Experiment 2 microbial analyses were n = 25 and n = 30, respectively.
Mechanism of Impingement System for Each Replication of Experiments 1 and 2
An in vitro dust generation and collection system was designed and used for experimental testing (Figure 1). For sampling, each flask was covered by 4 layers of folded cheesecloth (VWR, Cheesecloth Wipes, CAT. NO. 21910-105 Radnor, PA), which had 2 holes for tubing connection to allow for equalization of pressure. For dust generation, air was blown onto the litter at 143 to 155 m/s, measured by flow meter (SKC Inc., Eighty Four, PA), using a 3.8 m tube (Tygon S3, OD= 9.5 mm, ID= 6.4 mm, Akron, OH) with one end connected to a laboratory airport and the other end equipped with a pipet (VWR 10 mL Serological Pipet, VWR International, LLC, Radnor, PA) as a tip. The air speed of 143 to 155 m/s was selected based on the speed of air necessary to generate visible dust within the flask that could simulate high levels of dust production in poultry houses, which are likely to be produced during times of increased bird activity such as during catching for harvest. The tip was continuously swirled by hand while blowing air onto the litter. Simultaneously, the generated dust was collected through a 0.5 m long tube, with the collection end equipped with a 12.7 cm long pipet tip (VWR 5 mL Serological Pipet, VWR International, LLC, Radnor, PA), by impingement (ACE Glass Incorporated, 7531 − 10 Midget Impinger Comp., Vineland, NJ) into 10 mL BPW (0.8 L/min for 5 min). The pipet end of the dust collection tube was covered with 2 layers of cheesecloth, except for the first replication of Experiment 1 in which the tip was covered with 4 layers of cheesecloth, to prevent large particles from entering and clogging the impingement system.
Salmonella Typhimurium Inoculum Preparation
Salmonella enterica serovar Typhimurium used for Experiments 1 and 2 was developed at the US National Poultry Research Center in Athens, GA, and had naturally induced resistance to nalidixic acid. This marker strain, stored in glycerol stock at -80°C, was first grown on plate count agar for 24 h at 37°C. The colonies were then collected and suspended into sterile saline solution to achieve an optical density for 8 log10 CFU/mL (for the first replication of Experiment 1) or 9 log10 CFU/mL (for the remainder of replications of Experiment 1 and Experiment 2). The actual log10 CFU/mL was further confirmed by plating appropriate inoculum dilutions on Xylose Lysine Tergitol-4 (XLT4) (Criterion, Hardy Diagnostics, Santa Maria, CA) agar plates containing 100 mg/mL nalidixic acid and counting the presumptive colonies of Salmonella after the incubation period (24 h at 37°C). The actual log10 CFU/mL levels of prepared inocula were (7.98, 8.68, 8.40, 8.70, 8.88) and (9.04, 9.04, 8.93, 8.40, 8.62) for the 5 replications of Experiments 1 and 2, respectively. The prepared inocula were serially diluted or not to obtain planned different levels of Salmonella respective to the replication and experiment.
Moisture Analysis of Litter Samples
To determine moisture percentages, 10 g of litter from each flask was placed into an aluminum dish and dried in a drying oven for 48 h at 90°C. After drying, the litter was weighed, and the following equation was used to calculate moisture percentage:
[(Initial weight of litter before drying − Final weight of litter after drying) / Initial weight of litter before drying] x 100
Microbial Analysis of Litter and Dust Samples
Litter sample. Litter samples were analyzed for aerobic plate counts (APC), Salmonella, E. coli, and coliforms. Each litter sample weighing 10 g was mixed with 90 mL of sterile saline solution and stomached for 1 min. Following serial dilution, in a duplicate manner, 1 mL from an appropriate dilution was plated onto 3M Petrifilm aerobic count plates (3M Health Care, Convey Ave, MN) and 3M Petrifilm rapid E. coli/coliform count plates (3M Health care, Convey Ave, MN) and 0.1 mL was spread onto XLT4 agar plates containing 100 μg/mL nalidixic acid. E. coli/coliform count petrifilm plates and XLT4 agar plates were incubated for 24 h at 37°C and aerobic count petrifilm plates for 48 h at 37°C. The counts were recorded for respective microbes after the incubation period. In the case of getting no detectable Salmonella or E. coli from litter samples, the original saline diluted samples were further incubated for 24 h at 37°C and followed by streaking onto XLT4 and MacConkey (BBL, Becton Dickinson and Company, Sparks, MD) agar plates for Experiment 1 and onto CHROMagar Salmonella plus base (CHROMagar, Paris, France) agar plates for Experiment 2. The streaked plates were incubated for 24 h at 37°C before recording of final results.
Generated dust sample. Dust samples were analyzed for APC, Salmonella, E. coli, and coliforms as described for litter sampling. First, 5 mL of BPW containing collected dust was serially diluted and used for direct microbial analysis, and the remaining BPW (5 mL) was incubated for 24 h at 37°C and used for determination of microbial prevalence. The microbial counting of the sample was done by following the same procedure as described for the litter sampling.
Statistical Analyses
The microbial counts from litter and generated dust samples were log transformed to log10 CFU/g and log10 CFU/L, respectively. For Experiment 1, one-way ANOVA was used to analyze treatment effect (Salmonella inoculum levels) on litter and dust bacterial levels. Means were separated using Tukey’s HSD test, and level of significance set at P ≤ 0.05. Simple logistic regression was used to analyze the relationship between litter Salmonella and Salmonella in dust. In Experiment 2, data from litter and dust samples were analyzed using one-way ANOVA with the different litter moisture ranges as the treatment. Means were separated using Tukey’s HSD test, and the level of significance was set at P ≤ 0.05. Simple logistic regression was used to analyze the relationship between litter moisture levels and Salmonella in dust samples. Prevalence data were statistically analyzed using Fisher’s Exact test with significance at P ≤ 0.05. All data were analyzed using SAS Studio, release 3.8 Enterprise Edition.
RESULTS AND DISCUSSION
Experiment 1
Using the developed in vitro system, bacteria were transferred from poultry litter to generated dust. Litter and dust APC are presented in Table 1. Overall average APC of litter and dust samples were 10.55 ± 0.03 log10 CFU/g and 4.92 ± 0.07 log10 CFU/L, respectively. The mean APC contribution by air that was collected from the flask with no litter was 2.28 ± 0.07 log10 CFU/L. APC of litter did not differ between replications (P = 0.0699). Homogeneity in APC in litter samples between replications was likely due to collecting litter from one pen to run all replications of Experiment 1, therefore, minimizing litter source or house location as a potential confounding factor. APC from generated dust did not differ except for the first replication which had significantly lower APC (4.42 ± 0.15 log10 CFU/L, P = 0.0036). A possible explanation for the lower APC of dust samples during the first replication could be the increased restriction of dust entering the impingement system due to 4 layers of cheesecloth covering the dust intake tube of designed setup. In replications 2 through 5 only 2 layers of cheesecloth were used. APC of litter in this study are comparable with literature where wood shaving litter and straw litter had APC of 9.89 log10 CFU/g and 9.76 log10 CFU/g, respectively, during flock growout (Fries et al., 2005).
In the present study, litter was collected from one pen of a research broiler house to assess microorganism transfer from litter to dust in a simulation of maximum dust production. Litter moisture content did not vary between replications (P = 0.7854) and averaged 25.04% ± 0.09. Within the scope of this study, dust generated from the litter was not weighed or distinguished into settled dust versus airborne dust. The dust sampled in this study contained APC (4.92 log10CFU/L) that could potentially settle or remain airborne. Additionally, particle sizes were not measured. Covering the pipet tip with 2 layers of cheesecloth allowed for particles of a small enough size to avoid clogging the impingement system, which allows for passage of particles up to 1 mm. Therefore, this study data cannot be directly compared with literature studies where bacteria were generally assessed separately from air and settled dust (Skora et al., 2016; Yang et al., 2018). Even the differences in sampling procedures and instruments for air sampling can translate a significant variation in the measurement of microorganisms (Adell et al., 2014). More specifically, studies have shown that airborne aerobic bacteria can range from 0.385 to 4.484 x 104 CFU/ m3 in broiler houses, and culturable bacteria can be high as 2.9 x 106 CFU/m3 in the air of poultry farms (Lawniczek-Walczyk et al., 2013; Yang et al., 2018). Settled dust, collected by locating 3 metal plates (2 at the ends and 1 at the middle for each sampled house) at 1.6 m height, from 10 broiler houses had an average level of 3.2 x 109 CFU/g of total number of bacteria (Skora et al., 2016). These results provide support to the conclusion of this study that poultry dust can carry a significant APC if the poultry houses offer the conditions for high levels of dust production.
Table 1. Bacteria recovery from litter and dust samples from an in vitro dust production system, Experiment 1.
Table 2. Salmonella recovery from litter and dust samples from an in vitro dust production system, Experiment 1.
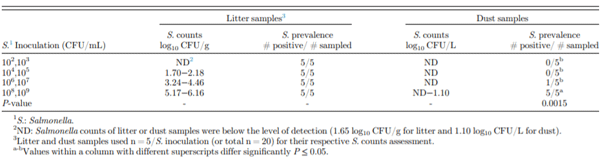
Results of E. coli and coliforms of litter and generated dust samples are presented in Table 1. The overall average counts (log10 CFU/g) of E. coli and coliforms of litter samples in this experiment were 3.74 ± 0.22 and 4.48 ± 0.27, respectively. Only one dust sample was confirmed positive for E. coli with counts equivalent to 0.57 log10 CFU/L. This dust sample was generated from litter having 6.14 log10 CFU/g of E. coli. Four of the dust samples were confirmed positive for coliforms (0.57, 0.10, 0.10. 0.40 log10 CFU/L). The respective litter samples used to generate these 4 coliform positive dust samples had 6.52, 6.19, 5.86, 5.78 log10 CFU/g counts of coliforms, respectively. No E. coli or coliforms were detected from the air that was collected from the flask with no litter. E. coli has previously been recovered in the air of broiler sheds with levels ranging from 102 to 104 CFU/m3, when the typical counts of E. coli in the litter were around 8 log10 CFU/g (Chinivasagam et al., 2009). With weekly analysis (total sampling for a 7 wk growout period) of the environmental condition of broiler houses it was reported that E. coli and coliforms in air ranged from 0 to 0.89 and 0.77 to 2.96 log10 CFU/m3, respectively. The same study found E. coli and coliforms in litter ranged between 0 to 2.85 and 1.39 to 4.73 log10 CFU/g, respectively (Hassan and Gherbawy, 2009). The discrepancy of present study results of E. coli and coliforms with literature might be explained by the relatively small volume of air sampled, the small volume of litter used in this study, the differences in litter types and age, the intentional generation of dust, or attachment of E. coli and coliforms with large particles which could not be transferred to the impinger collection media.
Salmonella recovery from litter and generated dust samples are shown in Table 2. Salmonella levels in litter ranged from 1.70 to 6.16 log10 CFU/g. Litter Salmonella levels were below the detection level (1.65 log10 CFU/g) when litter was inoculated with 10 mL of 102 to 103 log10 CFU/mL of Salmonella and allowed to equilibrate for 24 h. However, Salmonella prevalence was 100% from all inoculated litter samples (20/20). Salmonella enumeration results of 19 dust samples were below the level of detection (1.10 log10 CFU/L). One dust sample, generated from a litter sample having 5.54 log10 CFU/g of Salmonella, had 1.10 log10 CFU/L of Salmonella. Overall, Salmonella prevalence in dust samples obtained from the inoculated litter was 30% (6/20). No Salmonella were detected from the air that was collected from the flask with no litter. Significantly more Salmonella positive dust samples were obtained from litter samples with the highest levels of Salmonella (5.17 to 6.16 log10 CFU/ g, P = 0.0015) than the lower inoculum levels. A scatter-plot of the relationship between Salmonella levels of litter and generated dust samples is shown in Figure 2. Using logistic regression, increasing Salmonella levels found in litter were significantly related to Salmonella positive results in subsequent dust samples (P = <0.0001, R2 = 0.549, n = 20).
Figure 2. Scatterplot of predicted probability from logistic regression model of the presence of Salmonella in dust samples in relation Salmonella counts in litter samples. The graph equation is [ln (y/1-y) = - 8.4434+1.9505 (Salmonella counts of litter)]. R2 = 0.549.
![Figure 2. Scatterplot of predicted probability from logistic regres-sion model of the presence of Salmonella in dust samples in relation Sal-monella counts in litter samples. The graph equation is [ln (y/1-y) = - 8.4434+1.9505 (Salmonella counts of litter)]. R2 = 0.549.](/_next/image/?url=https%3A%2F%2Fimages.engormix.com%2FE_articles%2F47857_653.gif&w=1080&q=75)
Multiple studies have detected diverse bacteria from the air and settled dust in poultry houses, including Salmonella (Chinivasagam et al., 2009; Skora et al., 2016). Salmonella has been found to remain viable at least for 2 h in laboratory generated aerosols (McDermid and Lever, 1996). However, investigating aerosolized Salmonella viability over time in commercial poultry houses may better elucidate its hazards for poultry. It has been observed that airborne Salmonella can attach to dust with particle size ranges from 0.65 to > 7 μm in diameter (Adell et al., 2014). Moreover, airborne transmission of Salmonella spp. between poultry can occur (Holt et al., 1998; Harbaugh et al., 2006). Previously, Chinivasagam et al. (2009) explained the interrelationship between litter and aerosolized microorganisms in broiler sheds. They reported that the distribution of E. coli in broiler sheds demonstrates the litter-aerosol relationship. The microorganism transfer process occurred via the litter-dust-air interface. They consistently found that higher levels of E. coli in litter (̴108 CFU/g) led to higher levels in the air (102 to 104 CFU/m3). However, the same study did not find this relationship with Salmonella and Campylobacter due to their intermittent presence in litter and air. However, in this study, we observed that litter Salmonella levels play a significant role in dust being a carrier of Salmonella. Previously, the levels of Salmonella in settled dust and air have been reported as being varied between 1.1 x 105 to 6.3 x 105 CFU/g and 4.4 MPN/m3 to 3.3 x 102 CFU/m3, respectively, in poultry houses (Chinivasagam et al., 2009; Fallschissel et al., 2009; Skora et al., 2016). However, generated dust samples (19/20) in this study did not contain a countable number of Salmonella even though some were Salmonella positive after enrichment. This might be due to a potentially lower quantity of dust collected in 20 L of air, collection of only smaller particle sizes, or competition with other bacteria inside the collection medium (Adell et al., 2014).
It is important to note that the levels and prevalence of bacteria of dust in this study are results of an in vitro system where dust production was maximized, to represent “worst case” scenario of dust levels in poultry facilities, by blowing air onto litter to form visible dust. It is anticipated that this degree of dust production would occur during an event of significant litter disruption, such as during harvest for processing, at the commercial poultry farms. Quantities of dust in the air during normal production would not be as high, therefore, expected to pose a lower risk of Salmonella transmission. It would be valuable to extend this work to evaluate the transmission of Salmonella and other bacteria both over the course of time as well as during the times of elevated dust production, such as harvest.
Experiment 2
The objective of Experiment 2 was to assess the effect of different levels of litter moisture content on transfer of bacteria to dust from litter, and the results of this study are given in Table 3. The range of 5 different litter moisture percentages (%) achieved in this study was: 12.1 to 13.5, 17.0 to 19.0, 22.2 to 23.7, 27.2 to 29.0, and 32.3 to 34.8. Generally, the litter moisture content in broiler houses can vary from 15 to 57% based on several factors including litter type, ventilation, excreta, water leakage from drinkers, bird age, and growout season (Avc{lar et al., 2018). The counts of aerobic bacteria, Salmonella, E. coli, and coliforms in the litter did not differ (P > 0.05) with moisture adjustment of litter. The counts ranged from 10.16 to 10.33, 5.92 to 5.95, 5.93 to 6.00, and 5.94 to 6.02 log10 CFU/g, for APC, Salmonella, E. coli, and coliforms, respectively. The mean APC contribution by air used to generate dust from litter, as determined with the flask without litter, was 1.90 ± 0.07 log10 CFU/L. No E. coli, coliforms, or Salmonella was detected from the air that was collected from the flask with no litter. For the dust samples, the increase in litter moisture content resulted in a significant decrease of enumerated counts of APC, Salmonella, E. coli, and coliforms for each attained moisture range. This may be because an increase in litter moisture content offered more closely binding of dust particles with litter that reduced dust generation from litter and, consequently, decreased bacterial counts in dust (Ogink et al., 2012). However, the quantity of dust generated at different litter moisture content was not measured within the scope of this study. APC of generated dust samples differed by 2.01 log10 CFU/L at the lowest and highest litter moisture range. It is interesting to note that Salmonella, E. coli, and coliforms in dust samples were below the detection level at the 2 highest ranges of litter moisture (27.2 to 34.8%). Moreover, dust samples obtained from the highest moisture litter samples were negative for Salmonella, E. coli, and coliforms. This might be due to a decrease in dust generation with higher moisture levels. APC may have been more likely to be detected in dust due to the much higher levels present in the litter. Overall, litter moisture ranging from 12 to 24% offered an opportunity for a higher contamination of dust with litter microorganisms. The relationship between moisture levels of litter and Salmonella presence in generated dust is shown in Figure 3. Using logistic regression, increasing moisture levels found in litter led to Salmonella negative results in subsequent dust samples (P = <0.0001, R2 = 0.651, n = 20).
Table 3. Effect of litter moisture contents on transfer of aerobic bacteria, Salmonella, E. coli, and coliforms from litter to dust samples, Experiment 2.
Generally, airborne bacteria can be reduced by limiting dust production (Mitchell et al., 2004). This may be because of the attachment of airborne bacteria with fine dust particles (Zhao et al., 2014). Different approaches can be used to reduce dust levels in animal houses. Spraying techniques that prevent suspension or resuspension of dust particles in the air reduce dust levels (Zhao et al., 2014). Several studies assessed the effect of spraying agents, i.e., water, slightly acidic electrolyzed water, acidic electrolyzed water, to reduce airborne dust, and/or bacteria in poultry houses (Ogink et al., 2012; Zheng et al., 2014; Chai et al., 2018). Typically, humidification of bedding material with spraying agents can result in adhering of dust particles with litter, thereby decreasing dust generation (Ogink et al., 2012).
Previously, Ogink et al. (2012) reported that spraying of water 2 times per day at different rates (No water, 75 mL m−2, 150 mL m−2, 300 mL m−2) on top of bedding material in laying hen houses resulted in reduction of dust particle emission (18 to 64% and 44 to 64% for particles of less than 10 μm and 2.5 mm in size, respectively). However, they observed an increase in ammonia emission (21 to 64%) linearly with an increase in dosing rates of spraying water. Although, the high litter moisture content may reduce particulate matter emission from litter, it has also been observed in poultry facilities that high litter moisture levels are correlated to severe footpad dermatitis, reduce bird’s performance, negatively affect other animal welfare aspects (breast cleanliness, breast irritation, hock burn, and gait), and decrease carcass yield (Mayne et al., 2007; De Jong et al., 2014). High litter moisture content also can cause indirect adverse effects in poultry production houses by increasing ammonia production and emission, which is reported to negatively affect poultry health, by affecting the bird’s respiratory system and decreasing the bird’s ability to fight against infections, and impacting bird performance (Al Homidan et al., 2003; David et al., 2015). Similarly, Kim et al., (2006), while comparing 7 kinds of spraying additives to reduce dust levels and airborne microorganisms in slatted-floor swine houses, reported that the average reduction of all treatments after spraying was 30%, 53%, and 51% for dust, airborne bacteria, and airborne fungi, respectively, compared to their initial levels before spraying. The same study observed that the fluctuation of airborne bacteria and fungi with time after the spray treatments was “somewhat identical” to dust, because of the conjoint movement of airborne microorganisms and dust in the air. However, it has also been noted that airborne bacteria reduction can depend on properties of spraying agent (e.g., bactericidal effects) rather than on reduction of dust levels (Zheng et al. 2014).
Overall, the results of this in vitro work confirm that litter can be a source for aerobic bacteria, Salmonella, E. coli, and coliform transfer to dust that can be settled or aerosolized. Therefore, the assessment of litter Salmonella counts can be an indicator of potential dust Salmonella contamination. Litter moisture content plays a significant role in this transmission. Increasing levels of moisture tended toward lower dust contamination with bacteria. However, the drawbacks of increasing litter moisture content need to be considered. Based on this study, the development of mitigation methods to control dust contamination is recommended due its potential role in Salmonella transmission.
Figure 3. Scatterplot of predicted probability from logistic regression model of the presence of Salmonella in dust samples in relation litter moisture percentage. The graph equation is [ln (y/1-y) = 40.3163 -1.4492 (litter moisture %)]. R2 = 0.651.
ACKNOWLEDGMENTS
This work was supported by the United States Department of Agriculture Agricultural Research Service, Athens, GA Project Number: 6040-32000-069-01-S.
DISCLOSURES
All authors have participated in (a) conception and design, or analysis and interpretation of the data; (b) drafting the article or revising it critically for important intellectual content; and (c) approval of the final version. This manuscript has not been submitted to, nor is under review at, another journal or other publishing venue. The authors have no affiliation with any organization with a direct or indirect financial interest in the subject matter discussed in the manuscript.