INTRODUCTION
As a nonpolar amino acid, Valine (Val) is one of the most hydrophobic amino acids (Brosnan and Brosnan, 2006). Val, leucine, and isoleucine are called branched chain amino acids due to the branched chain structures of their R groups. In animals, Val serves as a precursor for the synthesis of protein and other amino acids (Ferrando et al., 1995). As a glucogenic amino acid, Val is a substrate for glutamine synthesis which is involved in the Krebs cycle (Wu, 2009).
It has been reported that Val may potentially be the next limiting amino acids for laying hens, after Met, Lys, Trp, and Thr (Lelis et al., 2014). Nevertheless, research on the Val requirement of commercial white-egg laying hen has been limited. The NRC (1994) suggests 700 mg total Val per hen per day is required for white-egg laying hens during peak production. Harms and Russell (2001) estimated total Val requirement of 41 to 47 wk old Hy-Line W-36 laying hens as 619.0 mg/d based on 47.2 g of egg content production. Bregen-dahl et al. (2008) reported digestible Val requirement of 501.3 mg/d based on 49.4 g of egg mass in Hy-line W-36 laying hens from 26 to 34 wk of age. In brown laying hens, Peganova and Eder (2002) reported a total Val requirement of 585, 735, and 634 mg/d in Lohmann Brown hens from 25 to 32, 24 to 32, and 46 to 54 wk of age using linear broken line model in their consecutive experiments. Lelis et al. (2014) evaluated that digestible Val requirement in Dekalb Brown laying hens from 43 to 54 wk of age was 567.0 mg/d by averaging results from 100% response quadratic, 95% response quadratic, linear broken line, and quadratic broken line models. In this experiment, the total Val requirement in small-framed first-cycle laying hens (41 to 60 wk of age) was estimated based upon performance using linear broken line, quadratic polynomial, quadratic broken line, and exponential models.
MATERIALS AND METHODS
Diets
Corn and peanut meal were selected as major ingredients to generate a Val-deficient basal diet. Amino acid concentrations of corn and peanut meal were determined before dietary formulation (University of Missouri AESCL, Columbia, MO 65211—AOAC Official Method 982.30 E(a,b,c), chp. 45.3.05, 2006). After the basal diet was mixed, basal diet samples were pooled and analyzed for amino acid analysis (Table 1, Almeida et al., 2013). In the basal diet, Val concentration was 0.515%, and this result confirmed a Val-deficient diet in comparison with Val requirement of 0.740% as reported by NRC (1994). Dietary leucine and isoleucine concentrations were determined as 1.024% and 0.699%, respectively. The concentrations of leucine and isoleucine were not expected to generate an antagonism with Val or impact the requirement estimation (Bray, 1970; Allen and Baker, 1972). Synthetic L-Val (96.5% feed grade, Ajinomoto Heartland, Inc.) was supplemented to the basal diet in 0.070% increments, resulting in experimental diets containing 0.515, 0.585, 0.655, 0.725, 0.795, and 0.865% Val, respectively. During the experiment, experimental diets were mixed every 2 wk from the same group of experimental ingredients.
Table 1. Composition of Val-deficient basal diet.1
Animals and Housing
All animal work undertaken in this experiment was approved by the Institutional Animal Care and Use Committee at Virginia Tech (Blacksburg, VA). A laying hen flock of 600 Hy-line W-36 laying hens were reared from day of hatch to mature laying hens using multi-phase diets and management as recommended in the Hyline W-36 Commercial Layers Management Guide (2015). At 41 wk of age, 270 hens were evaluated for overall good health and high production (average egg production: 90.8%) before being selected for inclusion in the experiment. Hens were assigned to 6 treatments with 15 replicates of 3 birds/cage (464.5 cm2/cage) over A-frame battery cage units. Hens were provided with approximately 95 g/hen/d feed and ad libitum access to water. Birds were housed in dark-out housing conditions and provided a 16:8 lighting schedule for the duration of the experiment. House temperatures were controlled via a fan and air-inlet ventilation system and ranged from 70 to 80◦F over the experiment. The hen-housed egg production (HHEP) for the 2-wk period before initiation of the experiment (39 and 40 wk of age) ranged from 90.3 to 90.5% across the randomly allocated treatments (P = 0.99).
Over the 19-wk experimental period, laying hens were visually inspected at least twice daily, at the morning check, eggs were collected and feed provided at approximately 10 am, and a follow-up check was provided after 4 pm each day. Egg numbers and mortality were recorded daily, but feed intake, HHEP, and FCR were calculated to correspond with bi-weekly feed manufacture periods. Each week, all eggs produced on Tuesday and Wednesday were collected for egg weight measurements, again calculated on a bi-weekly basis to correspond with feed manufacture periods. Meanwhile, 30 eggs from each treatment (2 eggs from 2 consecutive days in each experimental unit) were stored for Haugh unit, relative albumen weight, relative yolk weight, relative shell weight and egg shell thickness determination and egg quality indices. Body weight data were collected every 4 wk.
Statistical Analysis
SAS 9.4 (SAS Institute Inc, Cary, NC) was used to analyze, feed intake, egg weight, daily Val intake, Haugh unit, relative albumen weight, relative yolk weight, relative shell weight, and shell thickness using a 1-way ANOVA with means separated by repeated measures using a Tukey’s adjustment. Body weight losses at the end of 60 wk of age were analyzed using a 1-way ANOVA with Fisher’s LSD test. Significance was accepted at P ≤ 0.05. Laying hen Val requirements were calculated by fitting regression based on average HHEP, egg mass, and FCR over the entire duration of the experiment using nonlinear models (linear broken line, quadratic broken line, quadratic polynomial, and exponential model) in JMP (SAS Institute Inc, Cary, NC) described by Bregendahl et al. (2008). Daily Val intake was the independent variable in linear broken line, quadratic broken line, and quadratic polynomial models and Val concentration was the independent variable in exponential model to estimate requirement. The reason why percentage Val was applied in exponential model was due to the limitation of the exponential model which can only estimate Val requirement on a percentage basis. In both quadratic polynomial and exponential models, Val requirements were estimated when 95% of maximum response was achieved. As 90 to 100% of the maximum quadratic response was typically presented to quantify a response in published reports, we chose the median level, 95%, to estimate requirements (Peganova and Eder, 2002; Kidd and Tillman, 2016).
The equation of the linear broken line model was as follows:
y = a − b (x − c) for x ≤ c and
y = a for x > c,
where y = response criteria, a = ordinate of the break-point, b = slope of the line for x ≤ c, c = abscissa of the breakpoint (= requirement), and x = Val daily intake.
The equation of the quadratic broken line model was as follows:
y = a − b(x − c)2 for x ≤ c and y = a for x > c,
where y = response criteria, a = ordinate of the break-point, b = coefficient of quadratic term for x ≤ c, c = abscissa of the breakpoint (= requirement), and x = Val daily intake.
The equation of the quadratic polynomial model was as follows:
y = ax2 + bx + c,
where y = response criteria, a, b, c are the coefficients of quadratic equation, x = Val daily intake.
The equation of the exponential model was:
y = a + b 1 − e−c(x−d)
where y = response criteria, a = intercept (performance of the lowest Val concentration diet in the model), b = maximum response due to increased Val concentration, c = slope, d = Val concentration of the lowest Val con-centration diet, and x = Val concentration of the diet.
RESULTS AND DISCUSSION
Laying Hen Performance
In the current experiment, quadratic responses were observed on HHEP, body weight loss, feed intake, egg mass, FCR, and Val daily intake by feeding different concentration Val diets to laying hens (Table 2). Average feed intake increased from 66.5 to 92.9 g/hen/d as Val concentration increased from 0.515 to 0.725%, and a plateau was reached after 0.725% Val concentration. After an initial drop from 41 to 50 wk of age, feed intake in 0.515 and 0.585% treatments recovered after 51 wk of age but could not fully overcome the Val deficiency (Figure 1A). Laying hens fed 0.725, 0.795, and 0.865% Val diets maintained a relatively stable feed consumption at approximate 92 g/hen/d throughout the duration of experiment. The dose response of feed intake in the current experiment was consistent with those reported previously (Harms and Russell, 2001; Peganova and Eder, 2002; Lelis et al., 2014). Feed intake reduction is a typical response of laying hens to Val-deficient diets. Harms and Russell (2001) fed deficient to adequate Val diets (0.525 to 0.765%) to Hy-line W-36 laying hens and feed intake ranged from 81.2 to 96.2 g/hen/d. In Peganova and Eder’s 3 brown layer trials (2002), the lowest Val diets, 0.44% Val, induced a low feed intake at 68 g/hen/d in hens from 24 to 32 wk of age during their first experiment. In the second and third experiment, the lowest Val concentration of 0.51% led to feed intakes between 109 to 110 g/h/d which was 4 to 7 g lower than the maximum feed intake. Although the lowest Val concentrations were only slightly reduced between their first trial and subsequent experiments (0.44 vs 0.51%), feed intake was more adversely affected in the first experiment. In current experiment, the low-est Val concentration diet (0.515%) led to a feed in-take of 66.5 g/hen/d. This Value was lower than that in Harms and Russell’s experiment in 2001 and Peganova and Eder’s experiments in 2002 when similar Val con-centration diets were fed to laying hens. Differences in macro ingredients (peanut meal vs. soybean meal) and crude protein of the basal diets may explain these feed intake differences.
Average HHEP increased significantly as dietary Val increased from 0.515% to 0.865%, with a plateau occurring after the 0.725% treatment over the 19-wk period. After the onset of experiment, HHEP of 0.515% Val treatment dropped dramatically during the first 10 wk to lower than 40% (Figure 1B). An adaptation on deficient Val was observed in 0.515% treatment as HHEP rebounded after 51 wk of age; however, no such adaptation occurred in 0.585% or 0.665% Val treatments. Decreased egg production caused by a Val-deficient diet has been observed in previous experiments. Harms and Russell (2001) supplemented dietary Val from 0.525 to 0.765% in Hy-line W-36 laying hens rations from 39 to 46 wk of age and egg production was lowest in 0.525% Val treatment at 77.9%. Bregendahl et al. (2008) reported egg production of around 50% (exact egg production was not reported) in Hy-line W-36 laying hens fed 0.47% digestible Val diet from 26 to 34 wk of age. Since Val is an important component for protein synthesis in egg, insufficient dietary Val reducing egg production is not surprising (Lewis, et al., 1950).
Table 2. Hen-housed egg production (HHEP), body weight (BW) loss, feed intake, egg weight, egg mass, feed conversion ratio (FCR), and Val daily intake from 41 to 60 wk of birds fed diets containing 0.515, 0.585, 0.655, 0.725, 0.795, and 0.865% Val, respectively.
Figure 1. (A) Feed intake, (B) hen-housed egg production (HHEP), and (C) body weight change from 41–60 wk of birds fed diets containing 0.515, 0.585, 0.655, 0.725, 0.795, and 0.865% Val, respectively.
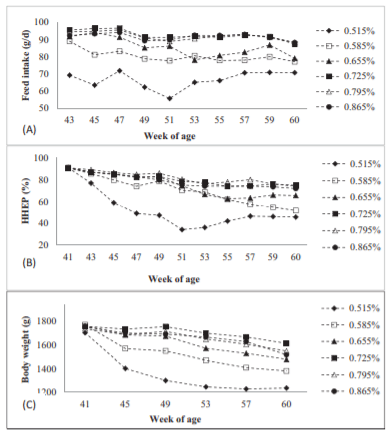
Body weight loss was observed in all treatments as compared to initial body weight. The 0.515% dietary Val concentration induced a highest body weight loss of 516.7 g/hen by the end of the experiment and this was decreased as dietary Val concentration increased from 0.515 to 0.725%. Birds were fed diets containing 0.795 and 0.865% Val, body weight loss showed numeric increases but no significant differences in comparison to the hens fed the 0.725% Val treatment. Body weight loss was found in all treatments, most likely due to the change to experimental diets and controlled feeding strategy applied over the experimental period. This controlled feeding was used to ensure feed intake similar to commercial hens (in the Hyline W-36 Commercial Layers Management Guide, 2015). Body weight of laying hens fed 0.515% Val diet dropped dramatically after receiving experimental diet and no further decrease was observed after 53 wk of age (Figure 1C). Meanwhile, an increase of both feed intake and HHEP were observed after 53 wk of age indicating the possibility of adaptation to the low Val diets may be occurring. The improvement in HHEP before an increase in body weight gain may indicate that egg production is conserved before body weight at least under the body weights observed in this experiment.
Egg weight increased from 56.5 to 62.1 g/egg as dietary Val increased from 0.515 to 0.725%. It is interesting to note that a reduced egg weight was observed as birds were fed diets containing 0.795 and 0.865% Val. The quadratic response on egg weight as increased dietary Val concentration was consistent with the response reported by Peganova and Eder (2002). In their experiment, egg weight kept increasing as dietary digestible Val concentration increased to 0.74%. When additional Val was supplemented in these diets (digestible Val of 0.80 and 0.86%, respectively), a numeric decrease in egg weight was observed. Harms and Rus-sell (2001) reported a reduced egg weight by feeding diets containing 0.525, 0.560, 0.595, 0.630% Val to lay-ing hens and egg weight was improved as dietary Val increased to 0.665, 0.700, and 0.765%. Unlike current and other reported data, egg weights were not reduced with excess Val in this experiment. Val requirements based on egg weight were estimated in previous studies (Harms and Russell, 2001; Bregendahl, et al., 2008). In the current experiment, although egg weight fitted a quadratic response, Val requirement was not reported due to a poor fit (R2 = 0.37).
Egg mass and FCR responded quadratically as dietary Val increased. Laying hens fed 0.515% Val produced 27.9 g egg mass per day. As hens were fed diets with additional Val, egg mass significantly increased until 0.725% Val was reached. A slight decrease in egg mass was observed as dietary Val concentrations climbed to 0.795 and 0.865%, most likely due to the numerically reduced egg weights associated with these treatments. Laying hens fed diet containing 0.515% Val showed a FCR of 2.62. FCR was improved from 2.62 to 1.89 as dietary Val concentration increased from 0.515 to 0.725%. A plateau was achieved as more Val was added to diets after 0.725% Val on FCR. Similar responses were reported previously for both egg mass and FCR (Harms and Russell, 2001; Peganova and Eder, 2002; Bregendahl, et al., 2008; Lelis et al., 2014).
Table 3. Egg quality and egg composition from 41 to 60 wk of birds fed various concentrations of dietary Val.
Egg Quality and Composition
Egg Haugh unit responded quadratically as dietary Val increased. Eggs from hens fed 0.515% Val showed the highest Haugh unit across all treatments (Table 3). As Val concentration increased, Haugh unit decreased from 86.6 to 82.6. In contrast, Peganova and Eder (2002) found no difference on Haugh unit by various dietary Val concentrations. Haugh unit, an albumen quality indicator, can be affected by factors such as bird age, molting, temperature, lighting and housing system (Travel et al., 2011); however, various dietary Val concentrations have not been reported to affect Haugh unit. Since all Haugh unit Values were above 82, the differences are noted, but might be questionable from a practical standpoint as these were all high-protein quality eggs. Harms and Douglas (1960) reported a higher Haugh unit when laying hens were fed diet containing 14.7% crude protein than those fed 16.7% crude protein. There were small differences in dietary crude protein in this experiment, but the relative differences were small enough to discount dietary protein as a major factor in Haugh unit response. Relative yolk weight result of eggs generated by hens fed various Val concentrations was mixed. Eggs from 0.515 and 0.795% Val treatments showed a significant higher relative yolk weight than those in 0.725% with that of other eggs being intermediate. Relative albumen weight in 0.515% Val treatment was significantly lower than those in other 5 treatments and a quadratic response was observed as dietary Val concentration increased. Egg relative shell weight in treatment 0.515% was significantly higher than those in other treatments. Shell thickness in the eggs from hens fed 0.585% Val was lower than those in other treatments (P ≤ 0.01). Lelis et al. (2014) reported no significant difference on relative yolk, relative shell weight, and relative albumen weight when digestible Val concentration varied from 0.555 to 0.666% in laying hen diets. In the current experiment, egg weight in the lowest Val concentration treatment was significantly lower than that in the other treatments, while there were no differences in egg weight with different dietary concentration in Lelis et al. (2014)’s study. A dramatic impact on egg weight by the most deficient Val treatment in the current experiment may explain the increased relative shell weight and reduced relative albumen weight. As the quantity of shell deposited on the shell membrane is generally not related to overall egg weight/size (Roland, 1980), the lower egg weight probably resulted in a relatively higher shell weight. These data may also indicate that albumen weight is a more sensitive indicator of Val status than relative yolk weight.
Val Requirement
Antagonism may exist among branch chain amino acids due to their similar molecule structure. Excess dietary leucine induces growth-depressing effect in several species (Harper et al., 1984). High leucine intake increases the activity of branched chain α-keto acid dehydrogenase, the key enzyme involved in degradation of leucine, isoleucine, and Val (Harris et al., 2001). Increased activity of branched chain α-keto acid de-hydrogenase leads to an increased oxidation of Val and isoleucine resulting in a reduced efficacy of these amino acids (Smith and Austic, 1978). In the current experiment, the leucine concentration in experimental diets was 1.024%, which was higher than the NRC leucine requirement at 0.86% (adjusted feed intake as 95 g/d). Allen and Baker (1972) reported increased dietary leucine resulted in decreased Val efficiency of utilization in chicks and that Val efficacy reached a minimum Value of 74% of normal with a 5.57% increase in dietary leucine in broilers (basal diet contained 1.20% leucine). The leucine concentration in current experiment was lower than the leucine concentrations that generate antagonism and an impact on Val requirement by dietary leucine should not be expected. There-fore, we conclude that no performance depression was observed by dietary leucine concentration on HHEP, feed intake, or egg weight in Val sufficient treatments in current experiment.
Table 4. Val requirement estimated from linear broken line, quadratic broken line, quadratic polynomial, and exponential model based on hen-housed egg production (HHEP), egg mass, and FCR.
Figure 2. Val requirement estimation using linear broken line re-gression based on (A) hen-housed egg production (HHEP) [y = 80.24 + 0.1222(x – 591.9), R2 = 0.83], (B) egg mass [y = 48.90 + 0.07885(x – 597.3), R2 = 0.87], and (C) feed conversion ratio (FCR) [y = 1.930 + 0.004247(500.3 – x), R2 = 0.62].
![Figure 2. Val requirement estimation using linear broken line re-gression based on (A) hen-housed egg production (HHEP) [y = 80.24 + 0.1222(x – 591.9), R2 = 0.83], (B) egg mass [y = 48.90 + 0.07885(x – 597.3), R2 = 0.87], and (C) feed conversion ratio (FCR) [y = 1.930 + 0.004247(500.3 – x), R2 = 0.62].](/_next/image/?url=https%3A%2F%2Fimages.engormix.com%2FE_articles%2F48594_679.gif&w=828&q=75)
The Val requirement was estimated based on HHEP, egg mass, and FCR using linear broken line, quadratic broken line, quadratic polynomial, and exponential model. In the linear broken line model, optimum Val requirement was 591.9 mg per day as the maximum HHEP was 80.24% (Table 4, Figure 2). Based on egg mass and FCR, the optimum requirement was 597.3 and 500.5 mg per day. Across all models, the Val requirement estimated by linear broken line was lowest. This agrees with the demonstration that linear broken line model typically resulted in a lower amino acid requirement in comparison with other nonlinear curve models (Fisher, et al., 1973). Daily egg mass has been of particular interest in the determination of the Val requirement of laying hens due to its economic importance (Peganova and Eder, 2002). In the current experiment, after conversion to Val requirement per gram egg mass production, the requirement was 12.2 mg/g egg mass. In similar research reports, linear broken line regression in Hy-line W-36 laying hens (Harms and Russell, 2001) resulted in Val intake of 11.0 mg/g egg mass (originally reported as 13.1 mg/g egg content). Bregendahl et al. (2008) estimated Val requirement as 11.7 mg/g egg mass in Hy-line W-36 laying hens from 26 to 34 wk of age (digestible Value was originally reported as 10.1 mg/g egg mass and 86% digestibility was applied to calculate total requirement). The estimated requirement from our study was close but slightly higher than requirements from other studies evaluating Hy-line W-36 Val requirement. The slight differences on Val requirement may be explained by different laying hen performance and hen age. In brown layers, Peganova and Eder (2002) reported that Val requirement was 13.3, 22.7, and 11.1 mg/g egg mass in 3 different trials at different ages (experiment 1: 24 to 32 wk of age; experiment 2: 25 to 32 wk of age; experiment 3: 46 to 52 wk of age). With the exception of the second experiment, the 13.3 and 11.1 mg/g egg mass requirement was similar to the estimation in the current experiment.
Using a quadratic broken line model, optimum Val requirement was estimated as 691.4, 719.7, and 650.0 mg per day based on HHEP, egg mass, and FCR, respectively. After conversion, Val requirement was 14.5 mg/g egg mass. As expected, this Value was higher than that estimated from linear broken line model. The quadratic feature of the model tended to overestimate the requirement. R2 was 0.92 indicating the best fit across different models. Lelis et al. (2014) estimated Val to Lys ratio as 0.9028 in Dekalb brown layers from 43 to 54 wk of age using the quadratic broken line model. The Val requirement was 648 mg per day based on egg production (86% digestibility was applied from Bregendahl et al., 2008) which was slightly lower than the current experiment. Using a quadratic polynomial model, optimum Val requirement was 697.5, 675.2, and 648.3 mg per day based on HHEP, egg mass, and FCR, respectively. Lelis et al. (2014) estimated Val requirement as 651 mg based on egg production (86% digestibility was applied from Bregendahl et al. 2008).
An exponential model was fitted using the HHEP, egg mass, and FCR data sets resulting in Val requirement estimates of 0.746, 0.744, and 0.646%, respectively. The Val requirement was 693.0, 691.2, and 600.1 mg per hen per day (using a feed intake of 92.9 g per day in this calculation). The Val requirement estimated from egg mass was close to 0.72% in Peganova and Eder’s (2002) second experiment but higher than 0.65 and 0.60% in their first and third experiments. Requirements estimated from exponential models were higher than those from using a linear broken line approach. The higher requirements generated by fitting exponential models were in agreement with that from Peganova and Eder (2002). As reported previously, the Val requirement for optimum egg mass using the exponential model was 8, 13, and 5% higher than the same estimate using broken line models. In current experiment, the Val requirement from an exponential model was 15.7% higher than a linear broken line model when based on egg mass. Val requirement estimated from FCR was lower than those from HHEP and egg mass in all models. Peganova and Eder (2002) also reported a reduced Val requirement based on FCR data in comparison to other performance parameters.
In conclusion, different dietary Val concentrations induce a dose response on HHEP, body weight loss, feed intake, egg weight, egg mass, and FCR. Hy-line W-36 laying hens from 41 to 60 wk of age need 12.2, 14.5, 13.6, and 14.0 mg Val per day to produce 1 g of egg mass using linear broken line, quadratic broken line, quadratic polynomial, and exponential models, respectively.
ACKNOWLEDGMENTS
This experiment was financially supported by Evonik Corporation. Mention of a trade name, proprietary product, or specific equipment does not constitute a guarantee or warranty by Virginia Tech, and does not imply approval to the exclusion of other products that may be suitable. The authors gratefully acknowledge the assistance H. Yakout, D. Shumate, R. Pound, A. Gusterer, B. Singh, C. Mou, N. Barrett, M. White, I. Omara (Virginia Tech, Blacksburg) for animal care assistance and feed mixing.
This article was originally published in 2019 Poultry Science 98:1272–1279. http://dx.doi.org/10.3382/ps/pey448. This is an Open Access article under a Creative Commons Attribution License.