INTRODUCTION
Chicken meat consumption has drastically increased in the past decades, and Australia has the fourth highest consumption of chicken meat in the world, eating 47.64 kg/person in 2020 (ACMF, 2021). The success of the poultry industry is highly related to the improved growth and performance capabilities of chicken meat birds with some strains of broilers capable of reaching market weight by 35 d of age (Singh et al., 2021). The industry now requires new approaches to further advance efficiency and production to keep up with consumer demand while still ensuring optimal health and welfare of chickens (Zuidhof et al., 2014).
The maternal environment, including maternal nutrition greatly impact the health, welfare and productivity of progeny (Angove and Forder, 2020). In chickens, embryonic development occurs in the egg and the egg must provide all the nutrients needed for the chick to grow and hatch (Tazawa and Whittow, 2000). Commercial chicken meat birds spend more than 33% of their life period in ovo, this highlights the importance of embryonic health and development (Givisiez et al., 2020). The use of in ovo technologies and in ovo supplementary feeding allows for additional nutrition to be provided to growing embryos with the aim of improving chick post hatch survivability and growth (Omede et al., 2017). Breeder hens reach sexual maturity and start producing eggs between 20 and 25 wk of age with mating for first fertile eggs occurring shortly after (ACMF, 2020). Prior to 30 wk of age, chicks produced by these young breeder hens have higher first week mortality and lower final BW compared to chicks hatched from older hens (Yassin et al., 2009). Reasons behind this may be attributed to younger flocks producing smaller eggs with smaller yolks (Yadgary et al., 2010), and thus less energy-rich lipids for embryonic development and hatch (Tazawa and Whittow, 2000). Towards the end of incubation, the increased energy demand has severely depleted glycogen reserves, this forces the embryo to utilize more muscle protein for gluconeogenesis resulting in inhibited early growth and development (Noy and Uni, 2010).
Creatine is a naturally occurring guanidino compound, synthesized by the body or absorbed from the diet; it stores and transports energy to meet cellular energy demand and is found in abundance the brain and muscle tissue where energy requirements are high (Rackayova et al., 2017). Previous studies have investigated the benefits of creatine both as broiler feeding trials (Khajali et al., 2020) and in ovo feeding trials (Peebles, 2018). Studies into in ovo supplementation of creatine monohydrate (CrM) by Zhang et al. (2016) found providing chicks with in ovo CrM and glucose increased BW in the 7 d post hatch. In ovo creatine pyruvate (CrPyr) was found to increase energy reserves (Zhao et al., 2017a), hatching weight and post hatch growth to d 42 (Zhao et al., 2017c). Improvements were also seen in post hatch fiber diameter and relative breast muscle weight (Zhao et al., 2017b). However, these studies only utilized male chicks from breeders in peak lay. The Australian chicken meat industry rears both male and female birds together; previous findings have demonstrated that males and females show different physiological responses to changes in both the maternal and in ovo environment (Angove et al., 2021). Thus, understanding in ovo nutrition in both males and females is fundamental when regarding management of flock uniformity in Australia. With this knowledge, the aim of the study was to improve the post-hatch performance of male and female chicks hatched from younger breeder flocks using in ovo CrM supplementation. It was hypothesized that in ovo injection of CrM would: 1) positively influence post-hatch growth and body composition, 2) increase fiber development and breast muscle yield in male and female birds hatched from young breeder flocks.
MATERIALS AND METHODS
All experiments were conducted with the approval of the Animal Ethics Committee (S-2021-023) of the University of Adelaide.
Incubation
Four hundred fertile broiler eggs (average 45 g) from young (27−29 wk) Ross 308 breeder hens were collected from a commercial breeder farm (Ingham’s Limited, Monarto South, SA, Australia) and transported to the University of Adelaide campus (Roseworthy, SA, Australia). Eggs were pre-incubated to room temperature (23°C) for 12 h and then incubated at 37.7°C and 55% RH from embryonic d 0 to 18, and 36.7°C and 60% RH from embryonic d 18 until hatch. Eggs were rotated 90° every hour until embryonic day 18 when they were transferred to hatching trays until hatch. During acclimatization, eggs were randomly separated into 3 treatment groups: non-injected control, saline injection and CrM injection.
In Ovo Injection
Injection solutions were prepared on embryonic day 14. CrM (Creapure, AlzChem, Trostberg, Germany) was dissolved in sterile avian saline (0.75%) solution to a concentration of 13.6 mg/mL (8.16 mg/egg). Concentration was based on previous findings where 12 mg of in ovo CrPyr resulted in the best performance improvements post-hatch (Zhao et al., 2017a), equivalent to 8.16 mg of CrM molecular weight.
Eggs were candled and early dead and infertile eggs were removed. The embryo, surrounding amnion, and air cell were identified by candling and marked. Injection sites (air cell and amnion) were disinfected with 70% ethanol, and punctured with a 23-gauge needle. The air cell was punctured to allow for pressure to be released. Saline and CrM solutions were injected into the amnion with a 25-gauge needle. Eggs assigned to the saline treatment (n = 123) were injected with 0.6 mL of 0.75% avian saline only, eggs assigned to the CrM treatment (n = 123) were injected with 0.6 mL of CrM solution. Control eggs (n = 134) received no injection. After injection, the site was sealed with Selleys glass silicone sealant and eggs were returned to incubation trays and placed back in the incubator within 40 min of removal.
Animal Husbandry and Tissue Sampling
At hatch, 24 chicks (12 male and 12 female) per treatment were euthanized by cervical dislocation. BW and sex were recorded, the heart, liver and both breast muscles were removed and weighed. Heart, liver and breast muscle tissues were frozen in liquid nitrogen and stored at 80°C for biochemical analyses. An additional 5 mm cross-sectional slice of right breast muscle tissue was collected and stored in 10% neutral buffered formalin for histological analysis.
The remaining hatched chicks (n = 380) were transported to the South Australian Research and Development Institute poultry unit (University of Adelaide Roseworthy Campus, SA, Australia). Birds were individually weighed, and leg tagged and chicks within treatment groups were randomly assigned to 24 raisedfloor pens (1.2 m £ 0.9 m; n = 8 replicates per treatment, 10 birds/pen). Pens were lined with paper and covered in wood shavings and birds were provided with ad libitum access to both feed and water. A commercially available starter feed (Laucke Mills Meatline Starter, Daveyston, SA, Australia) was fed from hatch to 24 d of age and a commercially available finisher feed (Laucke Mills Meatline Finisher, Daveyston, SA, Australia) was fed from 24 to 42 d of age. Daily health assessments, including noting any mortalities or required culls were conducted, with bird BW and feed intake measured weekly. The lighting schedule was 23L:1D from d 0 to 5 and 16L:8D from d 5 to 42. Temperature and ventilation within the shed were maintained according to standard management practices throughout the trial.
On d 42, ninty-six birds (2 male and 2 female per pen) were humanely euthanized by cervical dislocation and the same sampling procedure conducted as per hatch sample collection.
Body Composition (d 42)
A further 2 birds per pen (n = 48, d 42) were humanely killed by cervical dislocation, weighed and frozen in dorsal orientation. Total lean mass, total fat mass and total bone mass were obtained with the use of a dual-energy X-ray absorptiometry (DXA). Scans were conducted at the South Australian Research and Development Institute (Gilles Plains, SA, Australia) and performed on a GE-Lunar Prodigy DXA scanner (Serial number DF+13727, GE Healthcare, Australia) with enCORE software version 13.60.033 using Small Animal acquisition in medium mode. Analysis was performed using enCORE software version 18 using batch processing mode. Remaining birds were also humanely euthanized by cervical dislocation and their final BW and sex were recorded.
Histological Analysis
Breast muscle samples obtained at d 0 and d 42 were dehydrated using a graded ethanol series and embedded in paraffin wax with samples orientated for transverse fiber cross-section. Samples were sectioned 5 mm using a microtome (Microm model HM340E, Microm International GmbH part of Thermo Fisher Scientific OttoHahn-Str. 1A 69190 Walldorf/Germany), mounted to slides and stained with Hematoxylin and Eosin (H&E). Slides were scanned with a NanoZoomer (Hamamatsu model 2.0-HT, Sydney, NSW, Australia) and the corresponding NanoZoomer Digital Pathology (NDP.view) software was used to zoom into the image and obtain images for analysis with ImageJ (Schneider et al., 2012). A H&E filter was applied to the image and the pink (eosin) image was kept, converted to black and white and a number of fibers were selected to determine mean muscle fiber area (mm 2 ) and number of fibers per mm 2 (d 0 samples only; Figure 1 and 2) For d 0 samples, 10 to 15 fascicles were analyzed which resulted in an average of 300 fibers being analyzed per sample. At day 42, 8 to 11 sections were analyzed resulting total of 150 fibers by selecting a sample of 10 to 20 fibers from different regions of the section. Additionally, sections from d 42 were also assessed for presence/absence of myopathies, specifically muscle atrophy. A scoring system similar to Gratta et al. (2019) was used with samples assigned a 1 if fibers were predominately normal and a 2 if 33% or more of the fibers were abnormal.
Figure 1. Processing of breast muscle micrographs from Ross 308 chicks at hatch generated from ImageJ: Original image x40 resolution (A), Eosin stain (pink) image (B), binary mask (C), analyzed particles (D). Analyses provided mean muscle fiber area (mm2 ) and number of fibers per mm2.
Figure 2. Processing of breast muscle micrographs from 42 d old Ross 308 broilers generated from ImageJ: Original image x10 resolution (A), Eosin stain (pink) image (B), binary mask (C), analyzed particles (D). Analyses provided mean muscle fiber area (mm2).
Creatine Concentration Analysis
A subsample of fertile eggs (n = 15) were selected prior to incubation, internal contents of each egg were separated and freeze dried so yolk and albumin could be analyzed separately for baseline creatine concentration analysis. Frozen liver, heart and breast muscle tissue samples collected at hatch and d 42 were also freeze dried (Christ Alpha 1-4 LDplus Freeze Dryer, Germany), and sent to Alzchem (Trostberg GmbH, Germany) for analysis.
Briefly, tissue samples were homogenized with water by mixing. After extraction by stirring and sonification, the suspension was centrifuged, and an aliquot of the supernatant was filtrated through membrane filter and a SPE cartridge. In protein rich tissues, the proteins were precipitated with methanol, the sample centrifuged, and the liquid filtered through a 0.45 mm membrane filter and a SPE cartridge. Creatine (mg/kg) was immediately determined in the filtrate using an ion chromatography.
Statistical Analysis
Hatchability, mortalities/culls and abnormal fibers at d 42 were analyzed using a Chi-squared test. Continuous data were checked for normality with a Shapiro−Wilk test. Histological data were found to not be normally distributed, for hatch samples a log transformation was performed in SPSS prior to analysis. Histological samples from d 42 were analyzed using a Kruskal-Wallis test. All remaining data were then analyzed with a generalized linear mixed model with bird number the random factor at hatch and pen number the random factor at d 42. Sex, treatment and sex by treatment factors were analyzed. IBM SPSS Statistics 27 (Armonk, NY) was used to conduct all statistical analyses and differences were deemed significant at P ≤ 0.05.
RESULTS
Hatchability, Growth, and Performance
An increased hatch rate was seen in chicks given in ovo CrM (93.5%), compared to chicks given in ovo saline (88.6%) and the control group (88.8%) (Figure 3). This increased hatch rate was not statistically significant (P = 0.188) but was a 5.5% increase over the commercial hatch rate for this age flock (88%).
Figure 3. Hatch rate (%) of Ross 308 broiler chicks given an in ovo injection at embryonic d 14; Control) non-injected control group; Saline) 0.75% saline solution-injected control group; CrM) 8.16 mg Creatine monohydrate (CrM)/0.75% saline solution-injected group. Commercial hatch rate, shown as red dashed line, (Ingham’s Limited, Monarto South, SA, Australia). No statistically significant association was found between treatment and hatch rate (P = 0.188).
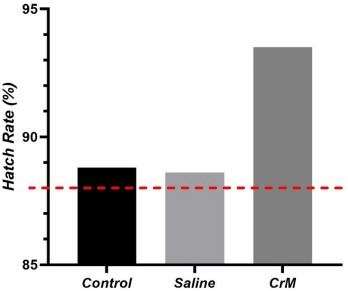
Figure 4. Average BW gain (kg) per week of Ross 308 broiler chickens given an in ovo injection at embryonic day 14; Control) non-injected control group; Saline) 0.75% saline solution-injected control group; CrM) 8.16 mg Creatine monohydrate (CrM)/saline solution-injected group. Industry represents the average BW expected for Ross 308 in commercial settings (Aviagen, 2019). Data presented as mean § SEM. # denotes statistical significance between control and saline treatments (P = 0.030) and control and CrM treatments (P = 0.037). * denotes highly statistical significance between male and female birds (P < 0.001).
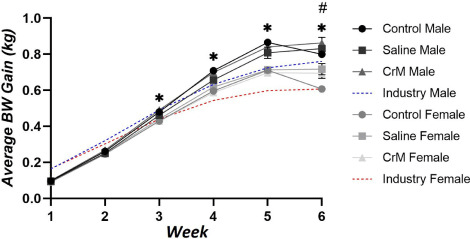
No statistically significant difference in average BW was found between treatment groups (P > 0.05; Additional file 1). From day 21 males weighed more than females (P < 0.001) and the average day 42 BW was 3.25 kg § 0.03 for males and 2.80 kg § 0.03 for females. In the first 5 wk of the trial, no significant difference between treatment groups in BW gain was found (Figure 4). The final week, birds in the CrM and saline treatment group gained more than birds in the control group (P = 0.037 and P = 0.030 respectively). From wk 3 onwards there was a highly statistical significance between male and female birds (P < 0.001) with males being heavier and gaining more than females. BW gain greatly exceeded expected industry gain for Ross 308 chickens (Aviagen, 2019).
Overall mortalities were quite low, with only 3 mortalities occurring during the trial. However, leg issues were observed in many of the birds therefore for ethical reasons a large number of culls occurred during the trial. Early culls were quite low (Figure 5) but during wk 4 and 5, the number of culls required greatly increased for the saline and control groups. However, during the final week of the trial, the CrM and saline groups saw a greater number of culls compared to the control group. The overall mortality/cull rate was 10.8% which is more than double the industry standard of 4% (ACMF, 2020), but there was no statistically significant association between mortalities/culls and treatment (P = 0.232).
Organ Weights
Treatment did not have a statistically significant impact on left (P = 0.613), right (P = 0.265), or overall (P = 0.680) breast muscle, or liver (P = 0.088) and heart (P = 0.300) proportions (Table 1). A sex effect was seen in the left breast muscle proportion, with females having more breast muscle in relation to BW than males (P = 0.014). This significance was not seen in the right (P = 0.267) or total (P = 0.310) breast muscle proportions. Birds hatched on embryonic d 21 were heavier (P = 0.008) and had larger percentage of left (P = 0.018), right (P = 0.003) but not overall breast muscle (P = 0.984) than birds hatched on embryonic d 22. Liver (P < 0.001) and heart (P = 0.038) as a percentage of BW were also greater in birds hatched on embryonic d 21.
Figure 5. Cumulative culls/mortalities per week of Ross 308 broiler chicks given an in ovo injection at embryonic d 14; Control) non-injected control group; Saline) 0.75% saline solution-injected control group; CrM) 8.16 mg Creatine monohydrate (CrM)/0.75% saline solution-injected group. No statistically significant association was found between treatment and overall number of mortalities/culls (P = 0.232).
Table 1. BW(g), breast muscle, liver and heart as a percentage of BW of Ross 308 broiler chicks at hatch given an in ovo injection at embryonic day 14.
At day 42, treatment also had no effect on left (P = 0.315), right (P = 0.325), or overall (P = 0.252) breast muscle, or liver (P = 0.380) and heart (P = 0.561) as a percentage of BW (Table 2). Despite having the smaller BW (P < 0.001), females had more breast muscle as a proportion of BW than males for left, right, and total muscle (P < 0.001). Females also had a higher proportion of liver (P = 0.011), but males had a higher proportion of heart muscle (P = 0.016).
Body Composition (d 42)
Bone (P = 0.646), fat (P = 0.693), and lean mass (P = 0.712) proportions were not affected by treatment (Table 3). There was a sex effect, males had higher proportions of lean (P = 0.012) and bone (P = 0.003) mass and females had a higher proportion of fat mass (P = 0.005). While not statistically significant, the sex effect of the average proportions of fat and lean mass were less pronounced in birds given the in ovo CrM treatment.
Breast Muscle Fiber Analysis
Mean fiber area (μm2) and number of fibers per μm2 at hatch were not significantly different among treatment groups (P > 0.05; Figure 6). The breast muscle of female chicks had larger mean fiber area, while the males had more fibers per μm2 (P < 0.001).
Mean fiber area (μm2) at d 42 was still not statistically significant between treatment groups (P > 0.05; Figure 7). At d 42 the female breast muscle tissue still had larger mean fiber area (μm2) compared to males (P < 0.0001).
Table 2. BW (kg), breast muscle, liver and heart as a percentage of BW of Ross 308 broiler chickens at day 42 given an in ovo injection at embryonic day 14.
Table 3. Bone, fat and lean mass as a percentage of BW of Ross 308 broiler chickens at day 42 given an in ovo injection at embryonic day 14.
Following the descriptions of abnormal fibers outlined by Gratta et al. (2019), Petracci et al. (2013), Mazzoni et al. (2015) and Radaelli et al. (2017) of: damaged overly round fibers, fibers with hyaline degeneration and damaged fibers undergoing phagocytosis samples were assigned a 1 if only a few of the fibers were abnormal and a 2 if a large number of the fibers were abnormal. Figure 8 shows classification of abnormal fibers and allocation of a 1 or 2 score.
There was no statistically significant association between treatment group and a high number of abnormal fibers (P = 0.745; Additional file 2). There was a statistically significant sex effect (P = 0.024) with more samples from males assigned the 2-score due to a high number of abnormal fibers within the sample. 27 female samples and 16 male samples were given a 1-score and 21 female, and 32 male samples were given a 2-score.
Figure 6. Mean fiber area (μm2) and number of fibers/μm2 of breast muscle tissue of Ross 308 broiler chickens at hatch given an in ovo injection at embryonic d 14; Control) non-injected control group; Saline) 0.75% saline solution-injected control group; CrM) 8.16 mg Creatine monohydrate (CrM)/0.75% saline solution-injected group. Error bars are § SEM. ** denotes highly statistical significance between male and female birds (P < 0.001).
Creatine Concentration Analysis
Greater concentrations of creatine were found in the yolk samples compared to the albumin (13.61 § 0.79 vs. 8.26 § 0.4 mg/kg; P < 0.001). Two of the egg yolk samples were removed from the analyses due to poor sample quality.
Significant differences in creatine were observed in both liver and heart tissue at hatch, where chicks that received the in ovo injection with CrM had increased creatine concentration compared to saline and noninjected controls (P < 0.05; Figure 9). An age by treatment effect was observed for liver tissue only, which was driven predominantly by the day 0 CrM treatment group having significantly greater levels of creatine at hatch (493.03 § 27.21 mg/kg), which had significantly decreased by d 42 (349.86 § 26.85 mg/kg; P < 0.001; Figure 9)
Figure 7. Mean fiber area (μm2) of breast muscle tissue of Ross 308 broiler chickens at d 42 given an in ovo injection at embryonic d 14; Control) non-injected control group; Saline) 0.75% saline solutioninjected control group; CrM) 8.16 mg Creatine monohydrate (CrM)/ 0.75% saline solution-injected group. Error bars are § SEM. ** denotes highly statistical significance between male and female birds (P < 0.001).
Figure 8. Micrographs showing damaged fibers in breast muscle tissue from 42 d old Ross 308 broilers. Samples with predominately normal fibers at 10x resolution (B) were assigned a 1 score for analysis. Samples with 33% or more abnormal fibers at 10x resolution (C) were assigned a 2 score for analysis. Analysis of damaged fibers found within the samples was used to determine if in ovo creatine prevented the development of abnormal muscle fibers.
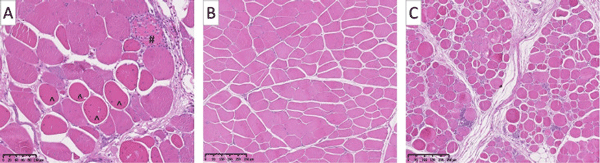
Figure 9. Mean creatine concentration (mg/kg) of liver (A), heart (B) and breast muscle (C) tissue of hatch (day 0) and d 42 Ross 308 broiler chickens. Birds received an in ovo injection at embryonic d 14; Control, non-injected control group; Saline, 0.75% saline solution-injected control group; CrM, 8.16 mg Creatine monohydrate (CrM)/ 0.75% saline solution-injected group. Error bars are § SEM. ** denotes highly statistical significance between treatment (A) and age (B and C; P < 0.001). Values without common letters are different between treatments, P < 0.05).
No differences in creatine concentration were observed in breast muscle between treatments at hatch or at d 42. Similarly, there were no differences in creatine concentrations between treatments for either liver or heart tissue at d 42 (Figure 9).
An age effect was observed for both heart and breast muscle. Creatine concentration decreased in heart tissue from 4882.48 § 82.52 mg/kg at hatch to 3816.81 § 69.91 at d 42 (P < 0.001). Interestingly, the opposite was observed for breast muscle, with a significant increase in creatine concentration from 5734.63 § 206.49 mg/kg at hatch to 18029.01 § 176.89 mg/kg at d 42 (P < 0.001; Figure 9).
There were no statistically significant differences in creatine concentrations between males and females at hatch or at d 42 for all tissues measured (liver, heart and breast muscle).
DISCUSSION
This study aimed to improve the post-hatch performance of male and female chicks hatched from younger breeder flocks using in ovo CrM supplementation. In this study we hypothesized that in ovo injection of CrM would positively influence post-hatch growth and body composition, and increase fiber development and breast muscle yield in male and female birds hatched from young breeder flocks.
The primary finding of this study was an increased hatch rate in chicks from young breeders given in ovo CrM. Although this finding was not statistically significant, the hatch rate of 93.5% was a 5.5% increase over the commercially expected 88% hatch rate seen in Ross 308 chicks from young breeder flocks. This study also found that hatch weight, post-hatch growth, breast muscle yield, and fiber number and size were not affected by in ovo CrM in the same chicks.
Previous research investigating in ovo CrPyr saw improved hatch weights, and BW gain at 7 (Zhao et al., 2018a,b), 21 (Zhao et al., 2017a) and 42 (Zhao et al., 2017c) days post-hatch when injected with 12mg CrPyr on embryonic day 17.5. However, the in ovo CrPyr treatment did not impact hatch rate in these studies (Zhao et al., 2017a,c). Creatine provides energy in muscle tissue via the enzyme creatine kinase, through the phosphorylation of creatine to phosphocreatine resulting in stored ADP being converted into ATP; a metabolically active energy source available to the tissue (Turner and Gant, 2014). Hatching requires extreme utilization of the energy stores within the chick for the chick to be able to successfully hatch (Noy and Uni, 2010). Metabolic rate drastically increases during the last 10% of incubation just prior to hatch; metabolic rate at this stage of incubation is 100% of the overall metabolic rate that occurred throughout incubation just prior to pipping (Tazawa and Whittow, 2000). This metabolic rate then rapidly spikes to over 140% of the overall rate just prior to hatch. This reinforces the increased energy demand required for a chick to hatch, as metabolic rate increases due to increase energy demand in late incubation. Muscle fibers used for hatch are exclusively anaerobic and muscle glycogen stores produced by the chick from the nutrition within the egg, are essential for the chick to have the energy to hatch; the yolk sac also provides lipoproteins and calcium and phosphorus providing additional energy and important skeletal development just prior to hatch (Moran, 2007). Muscle energy stores and yolk sac size therefore influence if the chick will have the energy required to hatch; and mortalities can occur in the egg or early post hatch if these energy stores are depleted too quickly (Maatjens et al., 2017). In ovo CrM likely provided chicks additional ATP by increasing the amount converted from ADP stores, which was then used during the hatching process preventing energy depletion. Resulting in more chicks being able to hatch and improving early post hatch survival. Previous in ovo creatine research did not see an improved hatch rate however, these studies used fertile eggs from breeder hens in peak lay older than 30 wk of age and with an average egg weight of 69 g (Zhao et al., 2017a,c). The eggs used in the current study from younger flocks were an average of 45 g. A study comparing eggs from 30 wk old and 50 wk old breeder hens found that the yolk comprised of 29.1% of the egg in eggs from the younger hens compared to 33.9% of the egg in the eggs from the older hens (Yadgary et al., 2010). The eggs from the 30-wkold hens were an average of 59.48 g, the eggs used in our study were from even younger breeder hens and were also much smaller, with an even smaller proportion of egg yolk. The smaller yolk would therefore result in reduced energy reserves available for embryonic growth and hatch (Tazawa and Whittow, 2000).
Creatine tissue concentrations were increased in the liver and heart at hatch but no differences in organ weights were observed. Creatine was not increased in the breast muscle at hatch. Interestingly, the previous in ovo creatine studies by Zhang et al. (2016) and Zhao et al. (2018b) did not see an increase in creatine concentrations in the liver at hatch. Furthermore, Zhao et al. (2017a) saw increased creatine concentrations in the breast muscle tissue at hatch. None of these studies investigated the creatine concentration in heart tissue. Creatine synthesis requires the amino acids arginine and glycine to be condensed to guanidinoacetic acid (GAA) in the kidney; and from this GAA, creatine is synthesized in the liver (Joncquel-Chevalier Curt et al., 2015). The addition of in ovo creatine should spare arginine reserves which in theory would result in an increase in the amount of creatine in the liver tissue which would explain the increase seen in our study. Evidence has been found to suggest that creatine supplementation can support heart health and metabolism (Kreider and Stout, 2021). Internal pipping at embryonic d 19 to 20 results in the start of pulmonary respiration increasing the demand on the heart to ensure oxygen demand at these tissues is met to begin the switch from predominately anaerobic to aerobic energy metabolism that occurs at hatch (Tong et al., 2013). Indicating that maintaining cardiac strength and output during this time is essential to survival; more research investigating the possible benefits of increased creatine on the integrity of the embryonic cardiac muscle should therefore be conducted. The energy provided by in ovo CrM could have been used in its entirety during embryonic growth and hatching, meaning these chicks now had the same creatine energy stores as the saline and control chicks for post hatch growth. Thus, explaining why no post hatch improvements were observed in this study.
Early studies by Fisher (1958) found the size of the “hatching muscle” (Musculus complexus) located at the base of the neck in birds and believed to provide strength to the neck for pipping, seemed to be associated with the ability to successfully hatch; with chicks that pipped but didn’t hatch having a smaller hatching muscle than those that hatched. This muscle has been mostly overlooked in more current research, but it would be interesting for future studies to investigate if creatine influenced the size of this muscle.
The previously mentioned in ovo CrPyr studies provided the injection to chicks on embryonic d 17.5 whereas this study injected chicks at embryonic d 14. Subramaniyan et al. (2020) investigated 3 different in ovo injection times during incubation and found that in ovo injection on embryonic d 14 saw improved hatch rate and hatchling weight over chicks given the same in ovo treatment on embryonic d 8 or 18. The treatment given in this study was a combination of synthesized silver nanoparticles and arginine, which as previously mentioned is one of the amino acids required for creatine synthesis. Despite previous studies injecting on embryonic d 17.5, this finding of the greatest improvements in hatch rate and weight being related to embryonic d 14 are why this time was selected. This earlier injection time could also explain the reason an improved hatch rate was seen here but not in other in ovo creatine studies and emphasizes the importance of providing in ovo supplementation at the optimal embryonic developmental age. In ovo vaccinations are usually given between embryonic d 17.5 and 19.2 and many in ovo feeding studies provide injections at embryonic d 17.5 (Peebles, 2018). The amnion is entirely consumed by embryonic d 19 (PoultryHubAustralia, 2021), in ovo feeding at embryonic d 17.5 would result in the amnion being rapidly consumed by the chick whereas injecting at embryonic d 14 would result in a slower feeding to the chick. By providing in ovo CrM earlier during incubation more of the energy could be utilized during early embryonic development potentially leaving less for post hatch growth.
Early post-hatch mortalities/culls were very low across all treatment groups but by wk 3, leg abnormalities were becoming apparent, and culls had to occur to maintain good bird welfare. Poor leg health is a common concern in broiler chickens, unfortunately genetic selection for growth has resulted in birds that are growing too heavy too fast for their bone strength to hold their weight (Bradshaw et al., 2002). Leg health issues can be exacerbated by incorrect incubation settings and poor quality feed (Muir and Groves, 2019; PoultryHubAustralia, 2021). Some strains of broilers have a higher predisposition especially those that see the most rapid early growth and poor nutrition and health of breeder hens can also result in an increase in musculoskeletal issues (Knowles et al., 2008). While not statistically significant, between weeks 3 and 5 the saline and control groups saw a larger number of culls compared to birds in the CrM group. Possibly the addition of in ovo CrM improved leg muscle strength so that leg abnormalities were less pronounced until the final week of the trial. This would need to be investigated further for full conclusions to be drawn. In regards to the final week plateau, d 42 is often used as the final grow out age during in ovo trials (Peebles, 2018). Due to the rapid growth of birds seen in this experimental situation it may be more appropriate for future studies to only grow birds to d 35. The BW gain of these birds greatly exceed that expected of them in a commercial situation, they likely hit a commercial weight before d 42. Completing a study at d 35 would mean the birds are younger but their final BW would likely still be a good representative of that seen by the industry.
In ovo treatment had no statistically significant effect on bone, fat or lean mass as a percentage of BW. Males had a statistically significant higher percentage of bone and lean mass while females had a higher fat mass percentage. The difference in fat percentage and lean percentage between the sexes was less pronounced in birds given in ovo CrM with females having more lean mass and males having more fat mass compared to controls and birds given in ovo saline, this finding was not significant but is interesting. A study by Chodova et al. (2021) also found that females had a higher percentage of abdominal fat than males supporting our body composition results. Flock uniformity is an important indicator of the overall health of a flock and in mixed flocks, uniformity is decreased compared to single sex flocks (Da Costa et al., 2017) Improving flock uniformity could result in improved bird welfare and even better economic gains (Gous, 2018). If in ovo CrM is responsible for male and female birds having a more similar fat and lean mass body composition in it could improve flock uniformity in a commercial setting that rears both sexes together. Despite not being statistically significant this interesting finding in relation to the impacts of in ovo CrM on body composition and potentially flock uniformity in mixed flocks warrants further investigation.
In ovo CrM did not improve the breast muscle weights at hatch or at d 42 in chicks from young breeder hens. These findings are in contrast to improved breast muscle weights at hatch day 7 d post hatch (Zhao et al., 2017b), and d 21 and 42 post-hatch (Zhao et al., 2017c) with in ovo injection of CrPyr.
In the current study, females had a higher proportion of breast meat, which is interesting as previous studies were inconsistent in their findings in regards to sex differences in breast muscle proportion. Danisman and Gous (2013) found no difference in breast muscle as a proportion of lean meat between the sexes in Ross 308s. However, Chodova et al. (2021) found that when on a low protein diet females had a higher proportion of breast meat but this finding was not seen in Ross 308s on a regular feed. A difference in breast muscle proportions was observed when comparing male and female Ross 308s grown to d 42 and 56 by CyganSzczegielniak et al. (2019). This difference was not statistically significant but the difference in carcass yield percentage was statistically significant between sexes and in both cases, females had higher proportions compared to males. These findings support the complexity of maintaining flock uniformity when males and females are reared together but also when hatched from different aged breeders, when considering treatments targeting improved muscle yield.
Breast muscle yield is important to keep up with consumer demand but muscle quality is also an extremely important factor to consider (Petracci et al., 2019). At hatch, breast muscle fiber area (μm2) and number of fibers per μm2 were not affected by in ovo CrM. This is consistent with the finding by Zhao et al. (2017b) who also saw no improvements to muscle fibers at hatch in chicks given in ovo CrPyr. However, improvements in fiber size were seen 3 and 7 d post hatch by Zhao et al. (2017b), and Zhao et al. (2017c) also saw increased muscle fiber size at 21 and 42 d post hatch in chicks given in ovo CrPyr. In a review into the effects of creatine on muscle hypertrophy, Gualano et al. (2010) postulates that not only does creatine promote muscle hypertrophy but it also prevents muscle atrophy and these mechanisms explain why creatine increases muscle fiber size. This is likely why increased muscle fiber size was seen up to 42 d post hatch in chicks given in ovo CrPyr. In our study, in ovo CrM had no effect on muscle fiber size at d 42 which contradicts the in ovo CrPyr research findings. Previous studies using CrPyr also used Arbor Acres broilers compared to Ross 308s used in our study. Breed does have an impact on muscle fiber size and development (Koomkrong et al., 2015) and even amongst different breeds of commercial broilers breast meat quality differed, which could be related to muscle fiber size (Jawasreh et al., 2019). The difference in breeds used could therefore also account for the different results seen.
At hatch and at d 42 female birds had larger muscle fibers compared to males, with males having more fibers per μm2. The number of muscle fibers is determined before hatch with fiber hypertrophy being the cause of post-hatch growth (Scheuermann et al., 2004). Breast muscle fiber hypertrophy is highly correlated to breast muscle yield (Koomkrong et al., 2015), further supporting the importance of breast muscle fiber growth to overall animal growth. Berri et al. (2007) also found that female broilers had larger fibers which correlated with increased breast yield as a percentage of BW compared to males. However this difference in fiber size between the sexes was not observed by CyganSzczegielniak et al. (2019) in Ross 308s grown to d 42 or 56. This seems to indicate more research needs to be undertaken to determine if fiber size is sex dependent. Regarding the effect of sex on meat eating quality, Abou-Kassem et al. (2019) found that male quails had higher quality meat while females had more tender meat, but this is yet to be investigated in chickens.
Improving the size of breast muscle fibers is a desirable trait in relation to increasing the amount of meat produced. Chodova et al. (2021) also found that an increase in breast muscle fiber size correlated with a decrease in shear force resulting in more tender meat. Indicating there may be a link between tenderness and muscle fiber size.
The selection for increased meat yield has resulted in increased occurrence of breast muscle fiber abnormalities in meat chicken birds (Mazzoni et al., 2015); and a correlation between poorer meat quality and a high number of abnormal fibers was discovered by Petracci et al. (2013). Despite creatine not improving the number of abnormal fibers in this study, studies have found that creatine supplementation attenuated corticosteroid induced muscle atrophy in the diaphragm, calf (Menezes et al., 2007) and hindlimb (Roy et al., 2002) muscle of rats. Studies into the effects of in ovo creatine on the number and severity of muscle fiber abnormalities have not been previously investigated. This finding in rats indicates the potential benefits of creatine to preventing the development of abnormal fibers warrants more investigation in broilers.
The effects of in ovo creatine on broiler post hatch growth and performance is still a relatively new area of research. In this study, in ovo creatine supplementation likely improved the energy status of the growing embryo resulting in the improved hatch rate and early posthatch survival but leaving little reserves for post-hatch growth. These findings have immense application to industry, with the potential to place more robust chicks on the ground and improve flock uniformity when hatched from breeder flocks of different ages. Future research should focus on finding the optimal time for embryonic injection to produce the best post-hatch improvements.
ACKNOWLEDGMENTS
Acknowledgement and thanks to Inghams Enterprises Limited for providing the eggs, The University of Adelaide Microscopy and Histology Services for use of their laboratories and equipment. Michelle Hebert (University of Adelaide, Roseworthy, South Australia) for her assistance with statistical analysis. Chris Schultz and South Australian Health and Medical Research Institute for use of their facilities and assistance with dual-energy X-ray absorptiometry scanning of chickens. South Australian Research and Development Institute for use of their poultry shed and facilities. This research was supported by Poultry Hub Australia; Project Number 20- 224.
DISCLOSURES
The authors declare that they have no known competing financial interests or personal relationships that could have appeared to influence the work reported in this paper.
SUPPLEMENTARY MATERIALS
Supplementary material associated with this article can be found in the online version at doi:10.1016/j. psj.2022.102447.