Introduction
The concept of maintenance has been defined as the state of balance between the intake of nutrients and their inevitable losses (Siqueira et al., 2011; Silva et al., 2014; Soares et al., 2019). That contributes to an understanding of the differences in responses between individuals of a population and enhances the requirements concept. Different methodologies have been developed to estimate nitrogen and amino acid requirements for maintenance (Burnham and Gous, 1992; Siqueira et al., 2011; Samadi and Liebert, 2006; Dorigam et al., 2014; Dos Santos et al., 2014; Silva et al., 2014). Some of these studies employed procedures that use a nitrogen-free diet and assume a linear relationship between nitrogen balance (NB) and nitrogen intake (NI), but these procedures have been criticized in the literature (Samadi and Liebert, 2006).
Traditionally, the use of a nitrogen-free diet enables the quantification of inevitable nitrogen losses and results in a negative NB of the bird. A negative NB is essential to estimate the maintenance requirement using the linear relationship between NB and NI. However, this procedure has been questioned because the deprivation of nitrogen or protein intake enhances the catabolism of body protein (Mitchell, 1924), and this physiological state does not correspond to practical farm conditions (Silva et al., 2014). Most maintenance requirements used in factorial models are assumed to vary with body weight or metabolic body weight (Lima et al., 2016; Reis et al., 2018; Silva et al., 2019; Melaré et al., 2019). However, it has been suggested that amino acid and protein requirements for maintenance are better related to body protein content (Emmans and Fisher, 1986; Siqueira et al., 2011; Lima et al., 2016).
A procedure published by Samadi and Liebert (2006) enables the determination of the nitrogen maintenance requirement (NMR) in birds with a positive NB using an exponential relationship between nitrogen excretion (NEX) and NI. The estimated intercept (NMR when NI = 0) corresponds to the NEX of a bird that receives a nitrogen-free diet (Samadi and Liebert, 2006). In addition, the NMR can be interpreted as the inevitable losses of nitrogen or endogenous protein, which reflects the catabolism of the amino acids that contribute to the inefficient use of dietary amino acids for protein deposition in growing animals (Silva et al., 2014; Ekmay et al., 2016). Adapting this concept and using experimental conditions in which lysing (Lys) is the first-limiting amino acid for protein deposition, the LMR can be determined using an exponential relationship between NEX and Lys intake (LI).
The objective of this study was to determine the Lys maintenance requirements of male and female using broilers as animal models.
Material and Methods
This study was approved by the local Ethics Committee on Animal Use (no. 007125-08). The experiments were conducted in Jaboticabal, SP, Brazil (21°15'16" S; 48°19'19" W, altitude 607 m).
Nitrogen balance trials were conducted in periods from 6 to 21 (I), 22 to 37 (II), and 38 to 53 (III) days of age, and 84 broiler chickens (Cobb500® genotype; 42 males and 42 females) were used in each trial. The birds were housed individually in metabolic cages and were distributed in a complete randomised design with seven treatments and six replicates of males and six females per experimental unit.
The treatments consisted of six graded lysine levels of 2.76 (L1), 5.88 (L2), 8.99 (L3), 12.1 (L4), 15.2 (L5), and 18.3 g kg−1 (L6) of diet in which the Lys:CP remained constant (4.91 g 16 g N−1) and Lys was assumed to be the first-limiting amino acid for growth. A seventh treatment (L7) was obtained by the addition of 4 g of L-Lys HCl (78%) kg−1 of feed to a diet similar to L1 that contained 2.76 g Lys kg−1 of diet (as fed) as a control diet. This diet was used to verify whether Lys was actually the first limiting amino acid in the diets. The experimental diets were obtained via diluting a summit diet with a dilution diet (Tables 1 and 2). The proportions used in the summit diet (L6) and the dilution diet (L0) to prepare the experimental diets were L1 = 15:85, L2 = 32:68, L3 = 49:51, L4 = 66:34, L5 = 83:17, and L6 = 100:0.
Three NB trials within period (6 to 21, 22 to 37, and 38 to 53 days of age) were conducted using six birds per diet and sex. The individual experimental period was divided into five days of adaptation and two consecutive excreta collection periods of five days each. Birds were fed ad libitum during the adaptation period and were restrictively fed during the collection period based on the feed intake measured in the adaptation period. At the end of the experimental period, the data from those two collection periods were combined to increase the number of replications. This procedure was used to compensate for the effects of age on feed intake as proposed by Samadi and Liebert (2006). The excreta were collected in trays and weighed at the end of each period and then frozen. At the end of each period, two birds per replication were killed to determine Lys content in the body.
The excreta samples obtained from each experimental unit during each period were thawed and homogenised. To determine body Lys content, the carcasses were processed in a meat mill. Representative quantities of the samples (excreta and carcass) were weighed in Petri dishes and frozen again (−20 o C) for freeze-drying. The samples were re-frozen and lyophilised for 72 h at −80 °C under 800 mbar of pressure (Edwards® 501 Modulyo freeze drier, West Sussex, United Kingdom). The samples were weighed to quantify the dry matter content and ground in a Micro Mill (IKA® A11 Basic Analytical Mill, Staufen, Germany). The total nitrogen content of the diets, excreta, and carcasses were analysed in a nitrogen distiller (Kjeltec™ 8400 Foss, Foss, Hillerod, Denmark) using the Kjeldahl method (Method No. 2001.11) according to AOAC (2004). The factor 6.25 was used to convert the nitrogen content to crude protein (CP). The total amino acid contents of the carcasses and the diets were analysed by Ajinomoto Ltd. using high-performance liquid chromatography (HPLC). The correction of the total amino acid content in the diets for digestible amino acids was performed using the tabulated digestibility coefficients (Rostagno et al., 2011).
Table 1 - Ingredients and nutritional composition of the basal diets used in the formulation of the experimental diets using the dilution technique (g kg-1)
Table 2 - Nutritional composition (as fed) of the experimental diets (g kg−1)
The data were analysed for one-way ANOVA using SAS software (Statistical Analysis System, version 9.1). The value of 0.67 data was used as the exponent for BW to eliminate the influence of BW on NB according to a basic model for growing animals (Gebhardt, 1980). The LI (mg BW0.67 kg−1 per day) was obtained considering the Lys concentration (c, g Lys 16 g N−1) in NI as suggested by Samadi and Liebert (2008):
LI = (NI × c)/16 (1)
A regression analysis between LI (mg BW0.67 kg−1 per day) and NEX (mg BW0.67 kg−1 per day) was used to fit an exponential function for males and females. The average Lys concentration analysed in body protein (BLys, as a % of body protein) was used as a constant value, so NEX at zero LI can be converted to the Lys maintenance requirement (LMR). The resulting equation from this relation can be described as:
NEX = (LMR × eb×LI)/(6.25 × BLys) (2)
From equation 2, the LMR (mg BW0.67 kg−1 per day) was estimated as the intercept of the model when LI = 0. The value 6.25 is used to convert nitrogen to protein.
The fitted models for males and females were compared using a statistical analysis to verify the similarity of model parameters. The following hypotheses were tested:
1 - H0 : LMRmale = LMRfemale = LMRgeneral vs. H1 : not all LMRs are equal;
2 - H0 : bmale = bfemale = bgeneral vs. H1 : not all b are equal;
3 - H0 : LMRmale = LMRfemale = LMRgeneral and bmale = bfemale = bgeneral vs. H1 : at least one parameter is not equal (LMR or b).
Based on these hypotheses, the following models were adjusted: Ω = unrestricted model, in which the two parameters (LMR and b) were adjusted to males and females; ω1 = restricted model, in which the LMR parameter is common for males and females (this model was verified by the hypothesis H0 (1)); ω2 = restricted model, in which the b parameter is common to males and females (this model was verified by the hypothesis H0 (2)); ω3 = restricted model, with all parameters common to males and females (general) (this model was verified by the hypothesis H0 – (3)).
The likelihood ratio test was used to test these hypotheses. Considering the samples of n observations, the distribution of the likelihood function (– 2 ln (L)) was approximately a chi-square distribution (χ2 ) with ν degrees of freedom (Regazzi, 2003) calculated as:
χ2calculated = -n × ln(RSSΩ/RSSω) (3)
in which n is the number of observations; RSSΩ is the residual sum of squares for Ω; and RSSω is the residual sum of squares for ω1 , ω2 , or ω3 . The probability value was subsequently subjected to a chi-square distribution with ν degrees of freedom.
To analyse the intercept of the exponential function, the models were chosen based on the significance degree of the statistical analysis (P-value). The first step was to analyse if one model could describe the response for males and females (ω3 , P> 0.05). If a general model was not possible (ω3 , P< 0.05), the next step was to analyse a model that described the same intersection point (in this case LMR) for both males and females (ω1 , P> 0.05). Thus, although the model for males and females describes different slope rates, an adequate model has LMR parameters that do not differ between males and females. If these cases were not possible, the models must be considered separately for males and females.
A Gompertz function (Gompertz, 1825) was fitted to the NB data to describe the body protein weight (BPt) as a function of time (t) for males and females:
BPt = BPm × e(-e (-B×(t- t*))) (4)
in which BPm is the mature body protein weight (kg), t* is the is the age when the growth rate is maximum (days), t is the time after hatching (days), and B is the rate of maturing of body protein (day−1). The LMR was recalculated from metabolic body weight (BW0.67) to metabolic protein weight at maturity (BPm0.73 × u), as suggested by Emmans and Fisher (1986). The BPm0.73 value is the metabolic protein weight at maturity (kg) and u is the degree of maturity of the protein at time t (u = BPt/BPm,), which corrects the requirements for age. An important distinction in the scaling here is that 0.73 is used only for mature weight and should not be used as BW0.73 in which BW is an immature body weight (Taylor, 1980).
Results
The N deposition was greater for birds receiving the control treatment (L7) compared with those receiving L1 diet, which confirms that Lys was the first limiting amino acid in the diet.
Both males (Table 3) and females (Table 4) responded significantly (P< 0.001) in N deposition because of the graded dietary Lys supply in L1-L6 diets. In addition, N excretion significantly increased (P< 0.001) with increasing N intake, exceeding the observed N deposition data for L6 diet for males in period III (Table 3) and females fed L6 diet in periods II and fed L5-L6 diets in period III. Although a response was observed in N deposition data, both males and females did not respond significantly (P> 0.05) in body weight and feed intake, except for feed intake in period III. However, it can be observed that body weight and feed intake were lower in birds fed the lowest Lys level than in those fed diets L2-L6.
Table 3 - Effects of the experimental diets (limiting in lysine) on the nitrogen (N) balance data (±SD)1 in male birds in each period
The average body lysine content (BLys) was determined as 6.4% of the body protein, and this value was used in equation 2 for modelling lysine maintenance requirements.
The exponential regression between LI and NEX were fitted according to equation 2, and the daily values for LMR were 9.29, 33.4, and 40.2 mg BW0.67 kg−1 per day for males and 9.36, 30.0, and 39.4 mg BW0.67 kg−1 per day for females. The estimated values of the intercept for LI = 0 were similar in each period studied for both sexes. Therefore, the similarity test of the models was used for the hypothesis of similarity of the model parameters b and LMR in each phase for both sexes (Table 5).
The similarity test of the models did not indicate differences between the LMR (P> 0.05) for male and female birds in period I. However, the obtained models in periods II and III are different for males and females (P< 0.05). The LMR was not different for both sexes in periods II and III (P> 0.05) and were estimated at 10.1, 31.5, and 39.8 mg BW0.67 kg−1 per day for periods I, II, and III, respectively. There was a good fit of the exponential model for the LI and NEX data, with R2 values higher than 0.89 (Figure 1).
Table 4 - Effects of the experimental diets (limiting in lysine) on nitrogen (N) balance data (±SD)1 in female birds in each period
Table 5 - Estimate of the parameters (±SD) of the unrestricted (Ω) and restricted (ω1 to ω3) exponential models from nitrogen balance data for male and female birds in each period
Figure 1 - Estimation of lysine maintenance requirement (LMR) via the exponential fit between lysine intake (LI) and nitrogen excretion (NEX) for male and female broilers.
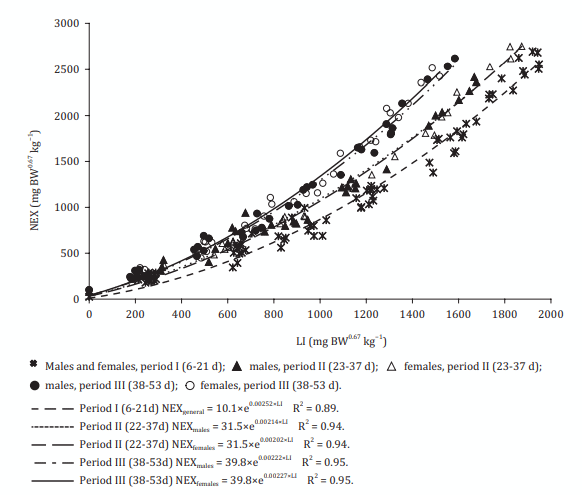
The BPm values used in the calculations were obtained from the Gompertz function (equation 4) and were 1.07 kg for males and 0.70 kg for females. The estimated t* values were 43 days for males and 37 days for females, and the B values were 0.04 day−1 for males and 0.05 day−1 for females. Using these coefficients for males and females in equation 4, the BPt were estimated as 30.2 and 29.6 g at 14 days, 188 and 169 g at 30 days, and 458 and 369 g at 46 days of age for males and females, respectively. Dividing BPt by BPm resulted in values of u: 0.03 and 0.04 (14 days), 0.18 and 0.24 (30 days), and 0.43 and 0.53 (46 days) for males and females, respectively. Recalculating the LMR from metabolic body weight (10.1, 31.5, and 39.8 mg BW0.67 kg−1 per day) to a metabolic protein weight at maturity (BPm0.73 × u) resulted in the following Lys maintenance requirements: 172 and 148 mg BPm0.73 kg−1 (period I); 216 and 207 mg BPm0.73 kg−1 (period II); 189 and 180 mg BPm0.73 kg−1 (period III) for males and females, respectively.
Discussion
The concept of maintenance has been widely accepted in animal nutrition. However, it is difficult to make reliable comparisons because of divergences in the mathematical procedures and response criteria used by authors to assess maintenance requirements. For example, Edwards et al. (1999) determined LMR by considering Lys and protein accretion equal to zero in the linear equations. However, considering zero daily Lys accretion (32.3 mg d−1 or 89.1 mg BW0.67 kg−1 per day) resulted in higher maintenance requirement estimates than zero daily protein accretion (2.50 mg d−1 or 6.90 mg BW0.67 kg−1 per day) for birds from 10 to 20 days of age. On the other hand, the estimates obtained from the exponential function in the present study indicated that a LMR of 9.29 and 9.36 mg BW0.67 kg−1 per day is equivalent to 4.69 and 4.48 mg BW0.67 kg−1 per day for males and females, respectively, for the period of 6 to 21 days of age, and thus similar to those obtained for zero daily protein accretion reported by Edwards et al. (1999). This implies that LMR for birds is not as high as suggested by Edwards et al. (1999) and is in line with those suggested by others (Emmert and Baker, 1997).
The LMR reflects the physical losses via endogenous protein in the intestine and integuments (Silva et al., 2011; Silva et al., 2014; Ekmay et al., 2016). The inefficient utilisation of dietary Lys for protein deposition and the inevitable endogenous losses resulted in daily LMR, which were estimated by the exponential relationship between LI and NEX as 9.29, 33.4, and 40.2 mg BW0.67 kg−1 per day for males and 9.36, 30.0, and 39.4 mg BW0.67 kg−1 per day for females during periods I, II, and III, respectively. This was expected because the proportion of the total daily requirement of amino acids necessary for maintenance is minimal during the initial period, but this requirement increases as the bird grows (Emmert and Baker, 1997; Silva et al., 2014; Araujo et al., 2014). The efficiency of Lys utilisation for protein deposition decreases with increasing weight of the bird combined with an increase in the maintenance (Eits et al., 2002; Araujo et al., 2014; Dorigam et al., 2014; Donato et al., 2016).
The efficiency with which absorbed Lys is used for protein deposition in growing young chicks is between 70 and 80% (Edwards et al., 1999; Fatufe et al., 2004; Araujo et al., 2014). According to this information, between 20 and 30% of Lys could be used for maintenance, which could explain the lower Lys maintenance requirement found in period I in relation to the requirement of Lys observed for growth (Baker, 1997). In this study, the tested models for period I are similar and can be used as a single model to describe the response in both sexes (NEX = 10.1 × e0.00252×LI, P> 0.05). The model similarity (P> 0.05) to describe the response for both males and females indicates that these birds exhibit the same pattern of initial growth regardless of sex (Table 5). According to the results of Marcato et al. (2008), protein deposition is similar for male and female birds up to 21 days (11.3 vs. 12.3 g/day) and become different thereafter. However, a single model was not able to represent the metabolic pattern of these birds during the other periods, as noted in the similarity test of the models (Table 5). The females in this study had lower LMR in period II (30.0 mg BW0.67 kg−1 per day) compared with the males (33.4 mg BW0.67 kg−1 per day).
The LMR estimated in period III were very similar for both sexes (40.2 and 39.4 mg BW0.67 kg−1 per day for males and females, respectively). In this period, the birds are close to the maximum protein deposition rate, which corresponds to 43 days for males and 37 days for females, calculated by equation 4. These values were similar because the ratios between the amino acid deposition in the carcass and catabolism remain fairly constant throughout the growth period after reaching the maximum protein deposition at this age (Sklan and Noy, 2004). Although these values are similar, one model is not sufficient to describe the responses of both sexes at this age, as demonstrated by the likelihood test (Table 5). One point on the axis of ordinates is capable of representing the LMR parameters for both sexes (P> 0.05), according to the test proposed by Regazzi (2003). Therefore, we adopted the daily values of 31.5 (period II) and 39.8 mg BW0.67 kg−1 per day (period III).
According to the coefficients estimated from Gompertz function, the maximum protein deposition rate corresponds to 43 days for males and 37 days for females (equation 4). In relation to these estimations, these birds presented a delay of six days to reach maturity in comparison to the estimates of 37 days for males and 31 days for females of Marcato et al. (2008). This delay could be explained by the limiting effect in the response of birds feeding the experimental diets, which were limiting in Lys. Therefore, the birds took longer to express their maximum potential for protein deposition, different from that observed by Marcato et al. (2008), wherein birds were fed diets not limiting in nutrients. However, the BPm values of 1.07 kg for males and 0.70 kg for females were close to the values estimated by Siqueira et al. (2010) of 1.09 kg for males and the values estimated by Marcato et al. (2008) of 1.04 kg for males and 0.67 kg for females of the Cobb500® genotype.
The energy from dietary protein that cannot be retained as body protein can be used for fat deposition. Providing a diet deficient in amino acids will thus increase fat deposition (Gous et al., 1990). Moreover, the fat deposition ratio of males and females are different (Sakomura et al., 2005). The change in body fat content represents a difficulty in the estimation of the maintenance requirement using traditional methods because there is little or no demand for amino acids for the maintenance of lipid reserves (Siqueira et al., 2011; Lima et al., 2016; Soares et al., 2019). As the amino acid requirement is more related to body protein deposition and given that at same body weight the birds could have different chemical body composition, Lys maintenance requirements are expressed more appropriately relative to body protein than to body weight (Lima et al., 2016).
According to Taylor (1980) and Emmans and Fisher (1986), the maintenance requirement should be related to protein weight and maturity of body protein (u), given by the relation between protein weight at time and protein weight at maturity. The scale used by these authors does not consider the fat content, which may eliminate the imprecision in determining the amino acid requirements due to variation of the fat content that vary widely in birds of the same genotype and similar body weight (Lima et al., 2016).
Therefore, the daily estimates in this study are more appropriately expressed as 172, 216, and 189 mg BPm0.73 kg−1 × u for males and 148, 207, and 180 mg BPm0.73 kg−1 × u for females in periods I, II, and III, respectively. The requirements expressed in this manner enable more refined comparisons between birds of different body weight across different studies; they also enable the recommendations to be extrapolated to birds of different ages and degrees of maturity of body protein.
In this research, we showed a way to correct the maintenance values determined with growing animals. When we use the system of protein weight at maturity and metabolic protein weight at maturity (BPm0.73 kg−1 × u), it is possible to extrapolate the recommendation even determined with young animals.
Siqueira et al. (2011) determined values between 141 to 174 and an average of 158 kg of BPm0.73 × u for adult roosters. In this research, the average value was 185 (148-216) mg per kg of BPm0.73 × u. The use of metabolic protein weight (Emmans and Fisher, 1986) and scale (Taylor, 1980) can be used to decrease the influence of growth on the estimate of maintenance. The values found were close to those determined with adult animals, especially when considering the variability. However, there is still an overall average effect when comparing young animal values of 185 versus 158 for adult animals, showing the influence of growth. However, this difference may have the influence of the studied amino acid lysine that has important effect on broiler growth (Dorigam et al., 2016), and future studies can be developed with other amino acids to confirm our hypothesis.
Conclusions
The lysine maintenance requirements for both sexes are 10.1 (period I: 6-21 days), 31.5 (period II: 22-37 days), and 39.8 mg BW0.67 kg−1 (period III: 38-53 days). The results provided ranges of lysine maintenance requirements values recommended in previous studies, validating this procedure. The procedure to estimate the requirements presented here provides new insights into the model of amino acid requirement estimations.
Conflict of Interest
The authors declare no conflict of interest.
Author Contributions
Conceptualization: J.C.P. Dorigam, E.P. Silva, N.K. Sakomura and J.B.K. Fernandes. Data curation: J.C.P. Dorigam, N.J. Peruzzi and M.B. Lima. Formal analysis: J.C.P. Dorigam, E.P. Silva, N.K. Sakomura and N.J. Peruzzi. Funding acquisition: N.K. Sakomura and J.B.K. Fernandes. Investigation: J.C.P. Dorigam, E.P. Silva, N.K. Sakomura and M.B. Lima. Methodology: J.C.P. Dorigam, E.P. Silva and M.B. Lima. Project administration: N.K. Sakomura and J.B.K. Fernandes. Resources: N.K. Sakomura. Supervision: N.K. Sakomura and J.B.K. Fernandes. Validation: N.K. Sakomura. Visualization: N.K. Sakomura. Writing-original draft: J.C.P. Dorigam, E.P. Silva, N.K. Sakomura, N.J. Peruzzi, M.B. Lima and J.B.K. Fernandes. Writing-review & editing: J.C.P. Dorigam, E.P. Silva and M.B. Lima.
Acknowledgments
The authors gratefully acknowledge Fundação de Amparo à Pesquisa do Estado de São Paulo (FAPESP) for financial support (grant number no. 2011/04313-8) and Ajinomoto Ltd., for crystalline amino acid donation and amino acid analysis.
This article was originally published in Revista Brasileira de Zootecnia, 49:e20180183, 2020 https://doi.org/10.37496/rbz4920180183. This is an Open Access article distributed under the terms of the Creative Commons Attribution License (http://creativecommons.org/licenses/by/4.0/).