INTRODUCTION
Salmonella and Campylobacter continue to be pathogens of concern associated with poultry and poultry products, which raise a public health issue globally (Antunes et al., 2016). As per the foodborne illness source estimation reports for 2016, Salmonella and Campylobacter are responsible for about 18 and 64% of poultry-related foodborne illnesses in the United States, respectively (IFSAC, 2018). With the increasing episodes of illnesses because of these pathogens, stringent regulations have been formulated by the US Department of Agricultures’ Food Safety and Inspection Service to reduce the risk of foodborne illness. This includes but not limited to the performance standards for Salmonella and Campylobacter as 7.5 and 10.4%, respectively, on postchill whole chicken carcasses and 15.4 and 7.7%, respectively, on raw chicken parts (USDA-FSIS, 2011; 2016). Therefore, the focus remains to reduce foodborne pathogen prevalence by applying antimicrobial interventions that aid in mitigating or limiting the chances of cross contamination during processing and handling.
Currently, the adoption of a multihurdle approach during poultry processing is the most effective strategy to control Salmonella and Campylobacter in poultry (Stopforth et al., 2007). Poultry processors have implemented several antimicrobial interventions at various locations throughout the processing line (e.g., inside– outside bird washer, carcass rinses, chilling, and so on). Chilling of carcasses in the poultry chillers with added antimicrobials is the primary processing step where antimicrobial interventions are incorporated during broiler processing and in some instances also serve as the critical control point of an overall Hazard Analysis and Critical Control Point pathogen reduction program. However, antimicrobial interventions such as postchill immersion tanks or spraying systems that use a higher concentration of antimicrobials with short contact times have been proven to be an added hurdle after primary chilling which further facilitates the reduction of pathogens on poultry carcasses (Nagel et al., 2013). In addition to the antimicrobial interventions for the chicken carcasses, cutup parts are also now being subjected to antimicrobial treatment in the postcutup decontamination tank to provide added antimicrobial efficacy against Salmonella and Campylobacter in the processing plants (Zhang et al., 2018).
There are several approved antimicrobials that have been evaluated and are used during primary and secondary broiler processing to reduce Salmonella and Campylobacter on poultry carcasses and parts (USDAFSIS, 2019). Organic and inorganic acids, chlorine, and cetylpyridinium chloride are few examples of antimicrobials that have been used for pathogen control in the past, however, currently, peracetic or peroxyacetic acid (PAA) is the most widely used antimicrobial/processing aid in chillers and postchill immersion tanks. Peracetic acid used for pathogen control during broiler processing is an equilibrium mixture of acetic acid and hydrogen peroxide and is an effective antimicrobial agent against bacteria and bacterial spores. The antimicrobial effects of PAA are due to its combined acidic and oxidizing properties (Fatemi and Frank, 1999). Peracetic acid is currently being used in the main poultry chiller up to a concentration of 220 ppm, whereas the maximum allowable concentration in the postchill immersion tank is up to 2,000 ppm (USDA-FSIS, 2019). Several studies have confirmed the antimicrobial efficacy of PAA against Salmonella and Campylobacter populations for poultry and poultry parts. Kumar et al. (2020) reported 1.76 and 1.78 log10 cfu/mL reduction in Salmonella and Campylobacter population, respectively, on inoculated chicken breast fillets when immersed in 500 ppm of PAA for 30 s. Smith et al. (2015) reported that PAA (200 ppm) was effective in reducing the Campylobacter population on broiler carcasses by 1.42 log10 cfu/mL of rinsate. Nagel et al. (2013) reported that postchill immersion application of broiler carcasses in 400 ppm of PAA for 20 s resulted in 2.02 and 1.93 log10 cfu/mL reduction of Salmonella and Campylobacter, respectively. Bauermeister et al. (2008) further reported that PAA concentrations as low as 25 ppm could effectively reduce Salmonella levels; however, higher concentration would be required to achieve greater reduction of Campylobacter. In addition to improving the safety of poultry meat, poultry processors tend to use antimicrobials at higher pH to improve the moisture retention of meat as higher pH has been associated with increased water binding, thereby increasing the yield of poultry meat (Alvarado and McKee, 2007). Validation of antimicrobials is extremely important because their efficacy is affected by several factors such as contact time, concentration, pH, and coverage. Although studies have been performed to indicate the efficacy of PAA at various concentrations against Salmonella and Campylobacter on poultry, research on the effect of higher pH of PAA solutions on the efficacy in reducing Salmonella and Campylobacter has not been reported. Thus, there is a need to evaluate the impact of pH on the efficacy of PAA against Salmonella and Campylobacter during processing, especially on broiler parts. The objective of this study was to evaluate the impact of PAA solution pH against Salmonella and Campylobacter on chicken wings.
MATERIALS AND METHODS
Bacterial Strains and Preparation of Inoculum
A cocktail of nalidixic acid–resistant Salmonella Typhimurium and a gentamicin-resistant strain of Campylobacter coli was used for the study (Kumar et al., 2020). The strains were obtained from the US National Poultry Research Center, US Department of Agricultures’ Agricultural Research Station, Athens, GA. Frozen culture of a nalidixic acid–resistant strain of Salmonella Typhimurium was streaked onto Brilliant Green Sulfa agar (Difco, Sparks, MD) supplemented with 200 ppm of nalidixic acid (Sigma-Aldrich, St. Louis, MO) and incubated for 24 h at 35°C ± 1°C. Fresh colonies (2–3) from Brilliant Green Sulfa agar were inoculated into 9 mL of tryptic soy broth (Remel, Lenexa, KS) + 200 ppm nalidixic acid and were incubated for 24 h at 35°C ± 1°C. After incubation, the cultures were centrifuged at 5,500 x g for 10 min, and the pellet was resuspended with 5 mL 1% PBS (Fisher Scientific, Fair Lawn, NJ) and centrifuged again. The supernatant was then removed, and the pellet was resuspended in 0.5 mL of PBS and added into 250 mL PBS.
A loopful of frozen culture of C. coli was streaked onto Campy-Cefex Agar (HiMedia Laboratories Pvt. Limited, Mumbai, India) supplemented with 200 ppm of gentamicin (Thermo Fisher Scientific, Ward Hill, MA) and incubated for 48 h at 42°C under microaerophilic conditions containing 85% N2, 10% CO2, and 5% O2. Campylobacter colonies were restreaked onto fresh Campy-Cefex plates twice to allow adequate formation of a lawn of bacteria. A lawn of Campylobacter was swabbed from the agar plates and added to 1% PBS. The final inoculum consisted of a cocktail of Salmonella Typhimurium (ca. 7 log10 cfu/mL) and C. coli (ca. 6–7 log10 cfu/mL) in 250 mL of PBS and was confirmed by direct plating. For each replication, a fresh inoculum was prepared on the day of the experiment.
Preparation of Antimicrobial Treatments
The antimicrobial treatments evaluated in the study included 50 ppm (0.005%) and 500 ppm (0.05%) PAA (Zee Company, Chattanooga, TN) at 3 pH levels (8.2, 10, and 11), along with water adjusted to pH 11 using sodium hydroxide (NaOH) (Mallinckrodt Baker Inc., Phillipsburg, NJ). As NaOH was used to adjust the pH of PAA solutions, it was included as an individual treatment to evaluate the antimicrobial activity of NaOH alone (without PAA). Because the commercially available PAA solution is an equilibrium solution with hydrogen peroxide and acetic acid, preparation of higher concentrations of PAA solutions result in a lower pH as the concentration of acetic acid increases simultaneously. The PAA concentrations used in this study were based on the levels permitted by US Department of Agricultures’ Food Safety and Inspection Service for use during poultry processing and preliminary work (data not shown). The solutions were prepared in individual plastic buckets (18.9 L; Encore Plastics, Forsyth, GA) using chilled water (≤4°C) and mixed thoroughly. The concentration of PAA for each treatment was confirmed using the PeroxyChem Titration kit (LaMotte Company, Ocean City, MD). The PAA treatments were adjusted to different pH levels by adding NaOH (1 or 10 N), and the pH of each treatment was confirmed using a benchtop pH meter (Orion Star A111; Thermo Scientific, Ward Hill, MA). The treatment solutions were maintained at 4°C to 6°C throughout the study.
Inoculation of Chicken Wings
Fresh skin-on broiler chicken wing flats (before any antimicrobial treatment) were obtained from a local commercial poultry processing facility and transported to the Food Safety Research Laboratory in the Department of Poultry Science, University of Georgia on the day of the experiment. Chicken wings were used in this study to present a worst-case scenario as wings are completely covered with skin and can potentially provide protection to microorganisms and prevent exposure to antimicrobial. For each replication, approximately 0.45 kg (1 lb.) of chicken wings per treatment was spray inoculated with 10 mL of inoculum containing a cocktail of resistant strains of Salmonella Typhimurium (ca. 7 log10 cfu/mL) and C. coli (ca. 6–7 log10 cfu/mL) in a laminar flow biological safety cabinet (Labconco Corporation, Kansas City, MO). The inoculated wings were placed in a biological safety cabinet for 15 min to allow bacterial attachment at room temperature.
Application of Antimicrobial Treatments
The objective of this study was to compare the efficacy of PAA against Salmonella and Campylobacter on chicken wings at different pH levels and contact times. The inoculated chicken wings (˜0.45 kg each) were treated with PAA (50 or 500 ppm) at pH 8.2, 10, and 11 or NaOH solution at pH 11 for 10 s and 60 min. The exposure times of 10 s and 60 min were used to represent typical exposure times for postchill dips and main chiller, respectively. Inoculated chicken wings were placed on a sanitized stainless-steel mesh basket (Model DND-095RND120-C04S; AnySizeBasket, York, PA) and immersed in antimicrobial solutions. Air agitation of the antimicrobial solution in each bucket was achieved by pumping air (103.42 kPa) from a compressor (Model D55146 Air Compressor; Dewalt, Towson, MD) to the bottom by means of 2 concentric circular plastic tubes (ID 0.64 cm; 11.5 and 15.4 cm, with 1.6 mm holes drilled at 2.5 cm intervals; McMaster-Carr, Elmhurst, IL) secured to a ceramic plate placed at the bottom of the bucket such that the compressed air (bubbling) is uniformly distributed at the bottom of each container. All antimicrobial solutions were prepared on the day of the experiment, and the inoculated wings that were not subjected to antimicrobial treatment served as a positive control.
Microbiological Analysis
After treatment, the wings were aseptically placed in sterile bags and rinsed with 100 mL buffered peptone water (Difco, Sparks, MD) containing 0.1% sodium thiosulfate (Acros Organics, NJ) for 1 min. Sodium thiosulfate was added to buffered peptone water to neutralize the antimicrobial effect of residual PAA on the chicken wings (Gamble et al., 2017). Rinsing was performed by vigorous shaking of the samples for 1 min to recover bacterial cells from the wing flats. Rinsates from each sample were collected and serially diluted in 9 mL PBS for Campylobacter or 9 mL PBS containing 200 ppm nalidixic acid for Salmonella. For Campylobacter enumeration, appropriate dilutions prepared using PBS were plated on Campy-Cefex agar supplemented with 200 ppm gentamicin, and the plates were incubated at 42°C for 48 h under a microaerophilic environment as described previously. Likewise, for Salmonella enumeration, appropriate serial dilutions were prepared with PBS + nalidixic acid and plated on the Aerobic Plate Count Petrifilm (3M Food Safety, St. Paul, MN) and incubated at 37°C for 24 h. Typical Campylobacter and Salmonella colonies were counted and reported as log10 cfu/mL.
Experimental Design and Statistical Analysis
A 2 (PAA concentrations; 50 and 500 ppm) x 3 (pH 8.2, 10, and 11) x 2 (exposure times, 10 s or 60 min) experimental design was used. Three independent replications of the experiment were performed. For each replication, fresh inoculum, PAA solutions, and NaOH were prepared, and chicken wings were obtained from different day of production. The data were analyzed using ANOVA in the GLM of SAS (SAS 9.4 Institute, Inc., Cary, NC). Statistical differences between the treatments were reported as least square means, and significance was reported at a level of P ≤ 0.05 (SAS Institute, 2004).
RESULTS AND DISCUSSION
The initial population of Salmonella Typhimurium on inoculated chicken wings (control) was 5.98 log10 cfu/ mL of the rinsate (Figure 1). Immersion of inoculated chicken wings in 50 ppm PAA at pH 8.2, 10, and 11 for 10 s resulted in Salmonella reductions by 0.64, 0.55, and 0.57 log10 cfu/mL, respectively. No differences (P > 0.05) were observed between PAA treatments (50 ppm), irrespective of pH levels and NaOH treatment at pH 11. Immersion of chicken wing flats in 500 ppm PAA solution for 10 s resulted in greater reduction (P ≤ 0.05) in Salmonella populations by approximately 1.0 log10 cfu/mL of the rinsate; however, pH of the PAA solutions did not impact (P > 0.05) the antimicrobial efficacy (Figure 1). Furthermore, no differences (P > 0.05) in Salmonella reductions were observed between the 50 ppm, 500 ppm, and NaOH treatments when immersed for 60 min. Irrespective of the concentration and pH of PAA, greater reductions (P ≤ 0.05) of Salmonella were observed for 60 min of immersion in PAA solution than those observed for 10 s of immersion (Figure 1). Thus, for 10-s immersion treatment, a higher concentration of PAA resulted in greater Salmonella reductions (irrespective of different pH levels). For 60-min immersion treatments, higher (P ≤ 0.05) reductions were observed with a 500 ppm concentration than those observed with the 50 ppm concentration at pH 10. Furthermore, higher (P ≤ 0.05) reductions were observed with a 500 ppm PAA treatment at pH 10 than those observed with NaOH at pH 11 for 60 min immersion.
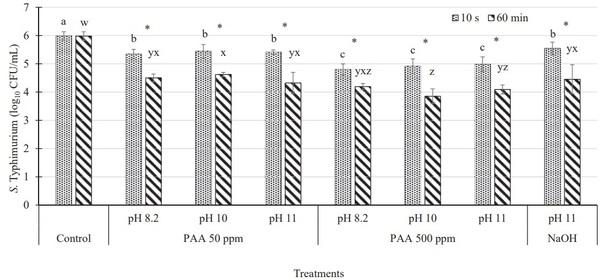
Figure 1. Salmonella Typhimurium population (log10 cfu/mL ± SD) recovered on inoculated chicken wings immersed in various concentrations of peracetic acid (PAA) adjusted to different pH levels (8.2, 10, and 11) and sodium hydroxide at pH 11 for 10 s and 60 min. a–cMeans with the different letter indicate significant differences (P ≤ 0.05) between treatments when treated for 10 s. w–zMeans with the different letter indicate significant differences (P ≤ 0.05) between treatments when treated for 60 min *Indicates significant differences (P ≤ 0.05) within treatment at different immersion time periods (10 s and 60 min) and same pH level.
The initial C. coli population on inoculated chicken wings (control) was 5.15 log10 cfu/mL of the rinsate (Figure 2). The PAA treatment (50 and 500 ppm) was effective (P ≤ 0.05) in reducing Campylobacter populations by 2.00 to 2.50 log10 cfu/mL on inoculated chicken wings when treated for 10 s or 60 min, whereas NaOH treatment (pH 11) resulted in Campylobacter reductions of 1.06 and 2.13 log10 cfu/mL when immersed for 10 s and 60 min, respectively (P ≤ 0.05; Figure 2). However, immersion time and the higher pH (8.2, 10, and 11) of antimicrobial solutions did not affect (P > 0.05) the antimicrobial efficacy of PAA (50 or 500 ppm) against Campylobacter (Figure 2).
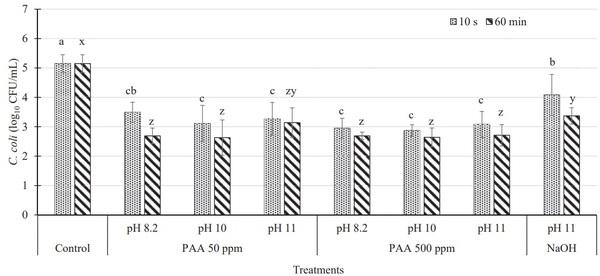
Figure 2. Campylobacter coli population (log10 cfu/mL ± SD) recovered on inoculated chicken wings immersed in various concentrations of peracetic acid (PAA) adjusted to different pH levels (8.2, 10, and 11) and sodium hydroxide at pH 11 for 10 s and 60 min. a–cMeans with the different letter indicate significant differences (P ≤ 0.05) between treatments when treated for 10 s. x–zMeans with the different letter indicate significant differences (P ≤ 0.05) between treatments when treated for 60 min *Indicates significant differences (P ≤ 0.05) within treatment at different immersion time periods (10 s and 60 min) and same pH level.
Similar to the results from this study, Chen et al. (2014) reported no differences in the reductions of Salmonella and Campylobacter irrespective of the PAA concentration (700 and 1,000 ppm) when whole carcasses were immersed in PAA solutions for 23 s. Nagel et al. (2013) reported no difference in Salmonella and Campylobacter reductions when treated with 400 and 1,000 ppm PAA for 20 s in a postchill immersion tank. Smith et al. (2015) reported a 1.45 log10 cfu/mL reduction in Campylobacter population when the broiler carcasses were subjected to immersion in 200 ppm PAA for 60 s. The lower Campylobacter reductions observed in this study compared with those reported by Smith et al. (2015) could be because of the higher concentration of PAA (500 ppm) and a lower PAA solution temperature (4°C) during immersion. In addition, Bauermeister et al. (2008) reported Salmonella reductions of 0.76, 1.05, and 1.29 log10 cfu/mL after a 1-h immersion treatment with PAA at 25, 100, and 200 ppm, respectively, whereas a concentration of 200 ppm PAA was required to achieve greater (˜1.5 log10 cfu/mL) Campylobacter reductions. Kumar et al. (2020) reported reductions in Salmonella and Campylobacter populations by 2.16 and 1.25 log10 cfu/mL, respectively when chicken breast fillets were immersed in 500 ppm PAA for 10 s.
In all the previous studies, the pH of PAA solutions under evaluation was much lower (2.6–3.4) than that of the present study where the antimicrobial efficacy was evaluated at higher pH values (8.2, 10, and 11) of the PAA solutions. The antimicrobial activity of PAA is due to its ability to alter the cell membrane permeability and interfere with the protein synthesis by oxidizing sulfhydryl and sulfur bonds in the bacterial cell, thus causing cell death (Oyarzabal, 2005). Furthermore, it has been reported that the lower pH of PAA further improves the oxidizing effect of PAA solution, thereby improving its bactericidal effect (Bell et al., 1997). The pKa of PAA is 8.2 at 25°C (Koubek et al., 1963), and it exists in dissociated form at higher pH values. Thus, adjusting the pH of the PAA solutions to levels higher than its pKa (8.2) can prevent dissociation of the PAA, rendering the weak acid mode of action irrelevant. In the present study, the temperature of all the antimicrobial solutions was maintained at 4°C to 6°C throughout the contact time (10 s or 60 min), which may have contributed to the lower pathogen reductions after immersion treatment. This is in agreement with reports from Dickson and Anderson (1992), where it was suggested that increase in temperature and contact time can increase efficacy of antimicrobials.
Published literature on the antimicrobial activity of NaOH against Salmonella and Campylobacter in poultry chillers is lacking. However, higher pH in scald water (addition of NaOH) resulted in a bactericidal effect (Humphrey et al., 1981). McKee et al. (2008) reported that higher pH (˜11.7) due to NaOH in scald water resulted in greater Salmonella reductions (ca. ˜ 1.8 log10 cfu/mL) than the scald water without an additive (pH 7.8). This is contradictory to the results from our study; however, this can be because the temperature of scald water (˜50°C for soft scald and 56.6°C for hard scald) as well as the surface temperature of the bird are much higher. In our study, the temperature of NaOH solution was maintained at 4°C to replicate chiller temperatures. Similarly, Dickson (1988) reported reduction in Salmonella Typhimurium inoculated on beef muscle and fat tissue by about 2 and 2.50 log10 cfu/cm2, respectively, when washed with 5% NaOH solution. Jay (2000) documented that the addition of NaOH leads to an alkaline environment, causing disruption in the enzyme activity of bacterial cells and nutrient transport into the cells. In the present study, PAA was effective even at higher pH levels, which can be owing to the combined antibacterial action of PAA and an alkaline environment owing to NaOH.
Immersion of inoculated chicken wings in PAA solutions was effective in reducing Salmonella and Campylobacter populations. Longer immersion time (60 min) was more effective against the pathogens, irrespective of the PAA concentration and pH. The present study also demonstrated that higher pH of PAA did not have an additional antimicrobial effect against Salmonella and Campylobacter. Although the US Department of Agricultures’ Food Safety and Inspection Service–approved use of PAA in primary chillers is 220 ppm, we used a higher concentration (500 ppm) in the present study to evaluate whether longer exposure would result in greater efficacy on exposure for a longer contact time. Further research in a commercial processing plant should be conducted to validate the efficacy of PAA at varying pH levels against Salmonella and Campylobacter for extended dwell times.