1. Introduction
Salmonella is one of the leading causes of foodborne outbreaks in the United States, responsible for approximately 1 million cases each year [1]. Poultry and poultry products have been implicated as a major source of Salmonella infection in humans [2]. Due to public health concerns, the Food Safety and Inspection Service (FSIS) mandated that meat-processing plants implement Hazard Analysis Critical Control Point (HACCP) program and meet USDA approved limits for Salmonella contamination on poultry and other meat products [3]. Since the implementation of HACCP program, U.S. poultry plants have reduced Salmonella contamination of carcasses to 7.5%, setting the new Salmonella performance standard for HACCP [4]. For some poultry companies to stay in compliance with the new HACCP standard will require pre-harvest, on-farm intervention strategies for Salmonella. Vaccination of poultry breeders has been shown to be efficacious in reducing carcass and egg contamination with Salmonella in commercial poultry [5–7]. However, continued on-farm surveillance for Salmonella becomes important in maintaining a “Salmonella-free” status [8].
Over 2000 serotypes have been identified for Salmonella enterica, based on antigenic differences in O, H1 and H2 antigens [9]. Despite the diversity of Salmonella serotypes, 30 Salmonella serotypes are responsible for 73% cases of salmonellosis in the United States, and S. enterica serotypes S. Enteritidis, S. Hadar, S. Heidelberg, and S. Typhimurium alone account for 37% these cases [10]. These Salmonella serotypes are also the same ones that commonly colonize poultry [11], and due to their public health significance, USDA Food Safety Inspection Service has imposed new regulatory actions on companies producing ground chicken or turkey containing any one of these Salmonella serotypes [12].
During the 1980’s, S. enterica serotype Enteritidis displaced S. Typhimurium in its frequency of isolation from outbreaks in the eastern United States [13,14], primarily through its contamination of USDA grade A table eggs [15]. In response to this emerging problem, the poultry industry and USDA implemented a series of steps to monitor layer flocks for S. Enteritidis, implement a Salmonella eradication program, and divert table eggs contaminated with this Salmonella serotype for pasteurization [8]. Though pasteurization is an acceptable resolution to S. Enteritidis for food safety, the producers lose valuable income when this method is necessary due to costs. Therefore, identification of S. Enteritidis positive flocks can have an important economic impact for the table egg producer.
Different methods have been developed to detect Salmonella in an effort to identify positive flocks. Current techniques include culture and isolation [16–19], PCR [20,21], agglutination tests [22], and enzyme-linked immunosorbent assays (ELISA) [23,24]. Current culture-based methods for detecting Salmonella vary in their ability to isolate all Salmonella serotypes and identify salmonellae with atypicalcolony type on differential/selective agar [18,25–27]. It takes at least 5-to-7 days to identify Salmonellafrom culture and an additional 4-to-5 days if a secondary enrichment is included [28–30]. Certain sampling methods like drag swabs [31] are also likely to miss salmonellae, especially on farms with intermediate to low-level contamination [32]. Simpler and cost effective methods for monitoring birds involve serological screens that measure antibody responses to specific microbes.
Serological screens have proven both practical and useful in the eradication of important veterinary pathogens from poultry farms [8] and at least in one study, a serological screen was useful in the eventual elimination of S. Enteritidis from poultry breeders in the Netherlands [33]. The U.S. National Poultry Improvement Plan (NPIP) has instituted serological agglutination tests to insure that birds are free of microbes like Mycoplasma gallisepticum and S. enterica Pullorum that have economic impact on the poultry industry [8]. These tests are routinely used by the poultry microbiology lab in the serological screening of flocks. The slide-agglutination test has been adapted to the serological detection of different Salmonella serotypes [34], including S. Enteritidis [22] but these are not able to compete with thesensitivity of ELISA tests [35,36]. Previous ELISA immunoassays have focused on Salmonella lipopolysaccharide (LPS) as the detecting antigen [37,38]. LPS is a powerful B-cell mitogen that induces a strong humoral response, directed mostly to O-antigen component of this polysaccharide [39]. Serology using LPS, which can produce cross reactions with other gram negative organisms, is not specific enough to reveal exposure.
Current ELISA tests for Salmonella have moved towards the use of purified flagellin as the antigen for the serological detection of specific S. enterica serotypes [40]. The Salmonella flagellins are a more appropriate antigen for serology due to reduced cross-reactivity [41]. Since Salmonella flagellin is not difficult to clone or express [42,43], we were able to purify recombinant Salmonella flagellar H1 antigens g,m, i, r, z10 and H2 antigens 1,2 and e,n,x using current protein fusion technology, and develop an ELISA assay for monitoring poultry for exposure to S. enterica serotypes S. Enteritidis, and S. Typhimurium. A serological test for those Salmonella of public health significance will be animportant tool for the industry to assess the effectiveness of their Salmonella eradication programs as well as identify farms where Salmonella contamination is a significant problem.
2. Results and Discussion
2.1. Expression and Purification of Recombinant Salmonella H1 and H2 Flagellin Antigens
Flagellin genes fliC and fljB from Salmonella enterica serotypes Enteritidis, Hadar, Heidelberg, and Typhimurium were amplified by PCR, cloned into the Pinpoint Xa-1 T vector, and introduced into E. coli BL 21. The Pinpoint Xa-1 T vector is designed for PCR cloning of foreign genes in-frame with abiotin purification tag (BPT), which is used for the purpose of purifying recombinant proteins by affinity column chromatography with avidin-bound resin. The expression of biotin-tagged flagellin was observed upon induction with 0.1 mM IPTG for all cloned Salmonella flagellin genes, producing the expected, 70.8 kD proteins, as determined by SDS-PAGE and confirmed by western blot analysis using H1 specific antisera (Figure 1). Cross-reactivity was observed for recombinant flagellin antigens in western blots using the commercial rabbit antisera. For proteins less than 70.8 kD, this cross-reactivity appears to be attributed to the rabbits’ immune response to other antigens shared with E. coli K12 strains. This cross-reactivity was eliminated when the recombinant flagellin was purified from E. colicells (see Figure 2). There was also some cross-reactivity observed for the 70.8 kD recombinant antigens with the different H1 typing antisera. However, the intensity of antibody binding was greatest for the recombinant H1 antigen paired with commercial antisera specific for that phase 1 antigen. The cross-reactivity observed with these antigens may be due to strong amino acid sequence conservation in the NH2– and –COOH ends of the Salmonella flagellin [44].
Figure 1. Sodium dodecyl sulfate polyacrylamide gel electrophoresis (SDS-PAGE) and western analysis of isopropyl-β-D-thiogalactoside (IPTG) induced and non-induced recombinant Salmonella fliC clones for H1: i, g,m, r, and z10 antigens. E. coli B21 with the recombinant fliC alleles i (lanes 2, 3), g,m (lanes 4, 5), r (lanes 6, 7) and z10 (lanes 8, 9) were allowed to grow to an optical density (λ 600 nm) of 0.5 before inducing expression of the protein fusion with 0.1 mM IPTG for 10 h (lanes 3, 5, 7 and 9). Lanes 2, 4, 6, and 8: non-induced cells. Lane 1: molecular weight standards. (A) SDS-PAGE of whole cell lysates of induced and non-induced E. coli B21 with recombinant fliC alleles. Recombinant Salmonella flagellin proteins were identified by western analysis (B–D) using Spicer-Edwards typing sera for i, g,m (B), r (C) and z10 (D) H1 antigens.
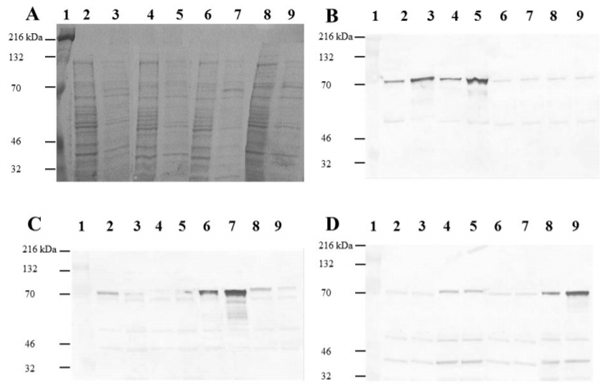
Purification of recombinant antigens from E. coli BL21 was achieved through sonication and Triton-X 100 solubilization procedures commonly used in purification of recombinant, protein fusions [45]. Flagellar protein tended to be insoluble and modifying the Triton X-100 concentration did not improve the solubility of the recombinant protein fusion (data not shown). Analysis of its amino acid sequence revealed extensive hydrophobic regions throughout the protein as determined using Kyte-Doolittle hydropathy plot that might explain the insoluble nature of the recombinant protein in Triton X-100. Substituting Triton X-100 with the chaotropic agent, guanidine HCl (6 M) did improve solubility and isolation of the protein from the insoluble fraction. The fractions were dialyzed against 50 mM Tris, pH 7.5 with 0.1% Triton X-100 to maintain solubility and compatibility with the avidin resin column. SDS-PAGE and western analysis demonstrated the protein was liberated from insoluble fractions following dialysis with Triton X-100 (Figure 2).
Figure 2. Purification of recombinant, biotin-tagged, S. Enteritidis H1 g,m antigen. Molecular weight markers (lane 1), S. Enteritidis wild type, whole cells (lane 2), E. coli BL21 whole cells (lane 3), E. coli BL21 with pJMZ42 induced with 0.1 mM IPTG for 10 h: whole cells (lane 4); insoluble fraction following removal of guanidine HCl via dialysis (lane 5); soluble fraction following removal of guanidine HCl via dialysis (lane 6); protein remaining in soluble fraction following binding of proteins to affinity chromatography resin (lane 7); and purified g,m recombinant antigen following elution from avidin beads with 5 mM biotin (lane 8). The arrow indicates the correct size for the g,m protein fusion. (A) SDS-PAGE. (B) Western Blot using Spicer-Edwards anti- H1 i, g,m sera to detect recombinant H1 g,m antigen.
2.2. Development and Evaluation of S. enterica Enteritidis, Specific ELISA Using Recombinant FliCg,m Protein Fusion as the Detecting Antigen
An ELISA based on the S. Enteritidis H1 g,m antigen was developed for detection of S. Enteritidis-infected birds. A checkerboard titration revealed that 500 ng of purified, recombinant H1 g,m antigen per well was optimal antigen concentration for the ELISA (Figure 3). A 1:100 dilution of chicken sera was used for determining quality control ranges for negative and positive control sera. Pooled sera from S. Enteritidis, bacterin-vaccinated birds (n = 10) and un-vaccinated, Salmonella culture-negative birds (n = 10) was used as positive and negative controls, respectively, and the quality control criterion was set for the ELISA from seven repetitions, two samples per repetition. Range for the positive control sera at OD490nm (HRP) was 0.78–1.07 (mean = 0.93, 2 standard deviation = 0.15) and negative control sera 0.03–0.11 (mean = 0.07, 2 standard deviation = 0.04). The diagnostic predictive value for the H1 g,m ELISA was determined for sera collected from chickens with known Salmonella exposure. Sera were collected from broiler breeders (n = 8) on a poultry farm in which the environment was culture-positive for Salmonella enterica Typhimurium but negative for S. Enteritidis. These sera were used to determine the negative baseline background from a S. Enteritidis culture-negative flock. Criterion for determining the appropriate baseline titer was defined as the highest titer of serum that could discriminate between known positive and negative samples. Since background was most apparent at the lower dilutions, we chose the dilution of 1:64 as the minimal dilution and OD490nm > 0.4 for the detection of H1 g,m-specific antibody titers (Figure 4) [46]. Next, H1 g,m antibody titers were determined for birds (n = 5) experimentally challenged with S. Enteritidis. The average H1 g,m antibody titer for birds exposed to S.Enteritidis was 1:256 with a minimum titer of 1:128. Western blots were performed to ensure that test sera were reacting with the recombinant protein fusions. The positive control sera and sera from S. Enteritidis colonized birds reacted with a protein band at approximately 70.8 kD, the expected size for the recombinant protein antigen. There was no reactivity with the protein antigen with sera from S. Enteritidis, culture negative birds (Figure 5).
Figure 3. Checkerboard titration of recombinant H1 g,m and positive control sera for S. Enteritidis.
Figure 4. Establishing negative baseline for S. Enteritidis enzyme-linked immunosorbent assays (ELISA) using the recombinant H1 g,m antigen. A-J sera are individual animal sera collected from a poultry farm that cultured positive for S. Typhimurium and negative for S. Enteritidis. Positive (solid line) and negative (dashed line) threshold optical density (OD) values were determined using from S. Enteritidis/H1 g,m flagellin vaccinated and unvaccinated chickens. The cut-off dilution and OD value for the recombinant H1 g,m ELISA was 1:64 and 0.4, respectively (dotted lines).
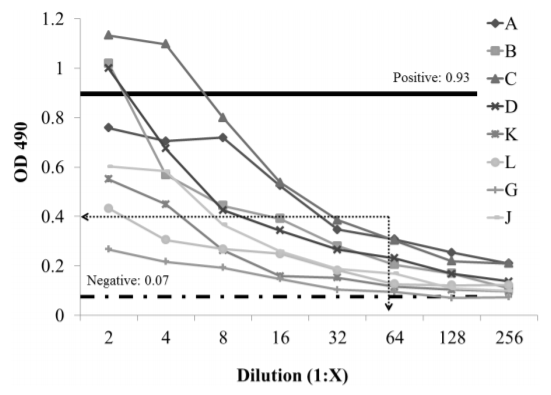
Figure 5. Western analysis demonstrates specificity of recombinant H1 g,m antigen. Positive control sera (lane 1), negative control sera (lane 2), S. Enteritidis challenged specific pathogen free leghorn birds (n = 5) (Samples: 37 9-5, 39 4-5, 40 9-5, 40 8-29, and pooled sera) (lanes 3–7). Sera samples from birds prior to S. Enteritidis challenge (1638, 1636, 1637, 37 8-8, 39 8-8, 1659, 1660, and 1670) (Lanes 8–15), Sera samples collected from S. Enteritidis-culture negative chickens (A, B, C, D, K, L, G, and J) (Lanes 16–23).
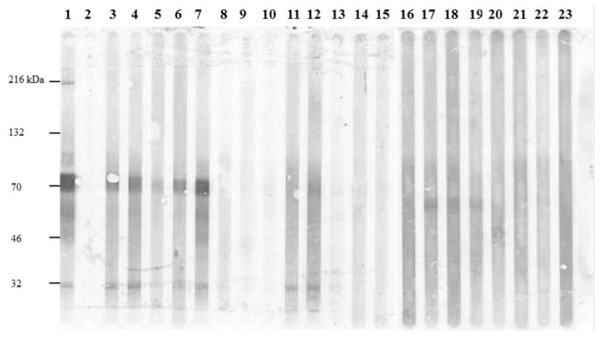
In this study, biotin-flagellin protein fusions were produced for developing ELISAs that detect specific Salmonella serotypes in poultry by monitoring serological responses to specific H1 and H2 flagellar antigens. A specific H1 ELISA was further developed by identifying optimal antigen concentration; positive and negative threshold for known vaccinated and unvaccinated birds; and negative baseline was established from chickens exposed to Salmonella serotype with flagellins other than the one included in the ELISA. This approach is important in establishing cut-off values (titers and OD) for determining positives for each recombinant flagellar antigen and later validation of vaccinates or birds exposed to a known Salmonella serotype. These parameters can be used to establish verity when determining titers of serum samples of unknown status. Any values at or near the baseline criteria will be considered “borderline” and additional tests may need to be administered to either confirm or deny whether the sample is indeed positive or negative. Positive identification of targeted Salmonella serotypes can be detected by addressing the combination of phase 1 (H1) and 2 (H2) antigens present in bi-phasic salmonellae. Therefore, this approach can be effective because it can discern which Salmonella serotypes are present in poultry flocks by monitoring antibody titers to specific H1 and H2 antigens.
Serology is used to detect the presence of Salmonella Enteritidis in layers due to the speed and accuracy of these tests [47]. Serological monitoring of poultry for Salmonella has many advantages. Poultry producers use it in breeders to screen for the presence of pathogens that may affect broiler and layer performance. Because serum is commonly collected from breeder at specified intervals, serotype-specific Salmonella screening can be applied with normal pathogen monitoring of the flock for exposure to important Salmonella serotypes S. Enteritidis and S. Typhimurium as well as immunological status of vaccinated flocks. A serological test is especially useful for identifying Salmonellapositive flocks where Salmonella is shed intermittently or at low levels in the poultry flocks. Application of a fast and accurate test will be useful to producers since the National Poultry Improvement Plan has strict guidelines for the monitoring of specific Salmonella serotypes, like S. Enteritidis [47]. Targeting parent lines of poultry is beneficial to producers because there are far fewer numbers of chickens to screen, and vertical transmission of Salmonella can be intercepted. This tool will allow producers to reduce the transmission to progeny flocks, thus ultimately improve the overall safety of their consumable product. Applications of serological tests may also have use as confirmatory measure because of serotype-specific nature of the tests. Using serological screens can assist the producer in decisions concerning fate of flocks or their poultry product. By using theses screens, producers can prevent infected poultry and poultry products from entering the food chain or accidentally contaminating the processing facilities.
One of the biggest disadvantages of current detection methods is the time needed to screen and identify an infecting Salmonella serotype. Culture and enrichment processes can take over a week for identification, especially if delayed enrichment is used [48]. Initial confirmation can be completed in 3 days. The ability to detect salmonellae responsible for foodborne outbreaks is essential for improving the safety of food [4,12,49]. Guidelines set by the NPIP are based on the producers’ ability to be able to determine not only whether Salmonella is present in their breeder flocks, but also have the ability to label the farm as “U.S. S. enterica Enteritidis Monitored” so their products can be shipped interstate [47]. Standards to determine quality of poultry and poultry products are based on rules of the NPIP. Since producers aim for the highest grade of poultry and poultry products, they need to have an effective detection system that can be accurately used in a manner set by the NPIP’s guidelines. An effective screen followed by an accurate and fast serological confirmation test can keep poultry farmers producing high quality and best-value products. This means highest profit while keeping harmful Salmonella from the consuming public.
3. Experimental Section
3.1. PCR amplification and Cloning of S. enterica Flagellin Genes, fliC and fljB from Salmonella Serotypes Enteritidis, Hadar, Heidelberg, and Typhimurium
Whole cell template for PCR was made for S. enterica serotypes S. Enteritidis, S. Hadar, S. Heidelberg, and S. Typhimurium as described by Hilton et al. [50]. Template was diluted 1:10 and1 µL of each dilution was used in PCR reaction. PCR primers were designed, using Gene Runner 3.04 (Hastings Software, Hastings, NY, USA), to amplify the complete H1 and H2 flagellin genes fliC and fljB, respectively, and allows for its insertion in-frame with the biotin purification tag on Promega’s Pinpoint Xa-T protein purification system (Promega, Madison, WI, USA). A Bam HI, restriction enzyme recognition site was engineered into the reverse fliC and fljB PCR primers to determine the DNA inserts orientation with respect to the vector’s biotin gene tag. Oligonucleotides, used for PCR and DNA sequencing, are listed in Table 1. PCR was performed using the Idaho Technologies Rapidcycler, hot-air thermocycler (Idaho Falls, ID, USA) [51]. The PCR reaction consisted of 3 mM MgCl2, 50 mM Tris (pH 7.4), bovine serum albumin at 0.25 mg/mL, 0.1 mM primer, 0.2 mM deoxynucleoside triphosphates (Roche Diagnostics, Indianapolis, IN, USA), 0.5 U of Taq polymerase (Roche Diagnostics), and 1 µL of whole cell template. Program parameters for the fliCPCR consisted of a hold at 94 °C for 15 s; 30 cycles of 94 °C for 15 s (denaturing), 60 °C for 15 s (annealing), and 72 °C for 35 s (extension) with a slope of 2.0; and a final extension at 72 °C for 4 min, while the program parameters for the fljB PCR was a hold at 94 °C for 15 s; 30 cycles of 94 °C for 15 s (denaturing), 55 °C for 15 s (annealing), and 72 °C for 35 s (extension) with a slope of 2.0; and a final extension at 72 °C for 4 min. PCR amplicons were visualized by gel electrophoresis using a 0.8% agarose gel containing 5 µg/mL ethidium bromide and run at 100 V for 45 min in TAE buffer [52]. The PCR products were purified using a Qiagen PCR purification kit (Qiagen Inc., Valencia, CA, USA).
Table 1. PCR and DNA Sequencing Primers.
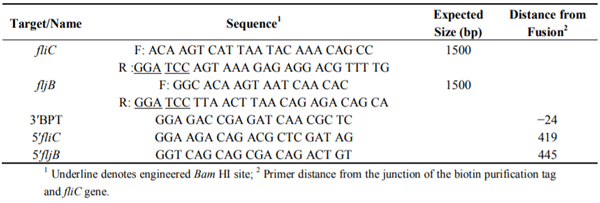
Amplified PCR products were cloned into Promega’s Pinpoint Xa1-T protein expression vector. PCR amplicons were ligated to vector at a 4.5:1 insert to vector DNA molar ratio with T4 DNA ligase according to the manufacturer’s instructions (Promega). Ligated plasmid DNA was transformed into Escherichia coli BL21 Gold (DE3) chemically competent cells according to the manufacturer’s instructions (Stratagene, La Jolla, CA, USA), and transformants were selected on LB agar with 100 µg/mL ampicillin (Bristol-Myers Squibb Company, Princeton, NJ, USA) (See Table 2 for description of bacterial strains and plasmids). E. coli transformants were screened for fliC or fljB DNA inserts by DNA: DNA hybridizations of colony blots. DNA: DNA hybridization was performed according to procedures described by Ausubel [53]. DNA was covalently bound to the membranes using an optimal setting of 1200 × 200 µJ/cm2 for 15–17 seconds on a Fisher Biotech UV cross-linker (Fisher Scientific, Pittsburgh, PA, USA). Probes specific for either fliC or fljB Salmonella serovar, were produced using the same primers for cloning except the amplicon was labeled with digoxigenin-tagged nucleotides present in the PCR reaction (Roche Diagnostics). Nylon membranes hybridized with probe at 68 °C overnight in pre-hybridization buffer containing 0.1% sodium chloride/sodium citrate buffer and 0.1% sodium dodecyl sulfate. Probe bound to membranes were detected with an anti-DIG antibody-alkaline phosphatase conjugate with color substrate solution of 4-nitro blue tetrazolium chloride (NBT) and X-phosphate/5-bromo-4-chloro-3-indolyl-phosphate (BCIP) (Roche Diagnostics). Positive colonies for fliC or fljB were traced back to the master plates for further screening by restriction analysis following plasmid DNA extraction.
Plasmid DNA was extracted using an alkaline lysis method [52] and digested with restriction enzyme Bam HI (New England Biolabs, Beverly, MA, USA) at 1 U per µg plasmid DNA. DNA was quantifiedby spectrophotometric measurement at OD λ 260 nm. Plasmid DNA was visualized by gel electrophoresis on a 0.8% agarose gel containing 5 µg/mL ethidium bromide. Plasmid DNA representative of phase 1 (H1) and phase 2 (H2) of flagellar antigens of S. Enteritidis, S. Hadar, S. Heidelberg, and S. Typhimurium are described in Table 2. At position 404 of the Pinpoint Xa1-T vector is a Bam HIrestriction site. The engineered Bam HI site on the PCR amplicon is 1.5 kb opposite the Bam HI restriction site in the cloning vector only when the inserted PCR product is in this correct orientation. Only when the PCR product is in the correct orientation, will the Bam HI liberate the DNA insert from the Pinpoint Xa1-T vector. DNA sequencing primers were designed, using Gene Runner 3.04, to confirm in-frame fusion of Salmonella flagellin gene with the vector’s biotin protein tag (Table 1).
Table 2. Bacterial Strains and Plasmids.
3.2. Expression S. enterica Flagellin, Protein Fusions in E. coli Cloning Host BL21.
E. coli with protein fusion constructs were grown in BHI media (Difco, Detroit, MI, USA) with ampicillin 100 µg/mL, with aeration, to an optical density (OD) (λ 600 nm) of 0.5 before inducing expression of the recombinant protein fusion with 0.1 mM isopropyl-β-D-thiogalactoside (IPTG) (Fisher Scientific) for 10 h at 37 °C. Cells were then centrifuged 5000× g for 10 min at room temperature, supernatant was decanted and cell pellets stored at −80 °C.
Sodium dodecyl sulfate polyacrylamide gel electrophoresis (SDS-PAGE) was preformed according to Lammeli et al.[58]. Polyacrylamide gels (3% stacking and 8% resolving) were cast and run using Bio-Rad Mini-PROTEAN II electrophoresis system with Kaleidoscope Pre-stained Standards (Bio-Rad Laboratories, Hercules, CA, USA) serving as molecular weight standards. Prior to induction with IPTG, one milliliter of cells was removed and served as a pre-induction control. Following 10 h of induction with IPTG, another one milliliter aliquot was taken as a post-induction sample. Total protein concentrations were determined using a BCA protein quantification kit (Pierce Chemical Company, Rockford IL, USA). Equal amounts of protein (approximately 400 µg) was added to SDS-PAGE solubilization buffer (25% Tris base, 2% sodium dodecyl sulfate, 5% 2-mercaptoethanol, 20% glycerol, and 0.0025% bromophenyl blue) and boiled (100 °C) for fifteen min. Samples were loaded (15 µL per sample) into the SDS-PAGE lanes and run at 26 mA, constant amperage for 2 h using Bio-Rad Mini-PROTEAN II electrophoresis system (Bio-Rad). Gels were run in duplicate for Coomassie brilliant blue staining and electrophoretic transfer to nitrocellulose membrane (Osmonics Inc., Westborough, MA, USA) for Western analysis (Bio-Rad). SDS-PAGE gels were transferred to nitrocellulose using constant voltage of 75 volts for 45 min and prepared according to the manufacturer’s instructions (Bio-Rad). Western analysis was performed as described by Towbin et al. [59]. Westerns blots were performed for each recombinant protein fusion construct to verify expression of fusion protein identified on SDS-PAGE gel stained with Coomassie blue, using either anti-biotin antibody-alkaline phosphatase conjugate (Roche Diagnostics) or Spicer-Edwards Salmonella typing, antisera (Difco) (1:5000 dilution). Anti-rabbit antibody-alkaline phosphataseconjugate (Sigma, St. Louis, MO, USA) was used as the secondary antibody for detecting Salmonella typing-antibody (1:5000 dilution). Western blots were examined for protein bands similar in size to the theoretical size of the recombinant protein fusion, 70.8 kDa.
3.3. Purification of Recombinant Salmonella Flagellin Proteins with Biotin Tag
IPTG induced cells, containing the recombinant protein fusion constructs, were resuspended in lysis solution (15 mL per gram cell paste) consisting of 6 M guanidine hydrochloride (Fisher Scientific) and 4 mg/mL lysozyme (Roche Diagnostics) and incubated at 37 °C overnight with shaking (200 rpm). Cellular debris was removed by centrifugation (5000× g for 10 min at 4 °C) and supernatant was transferred to 22 mm dialysis tubing with a 3.5 kDa molecular weight exclusion limit (Snakeskin®, Pierce Chemical Company). Guanidine hydrochloride was removed from the supernatant via dialysis against two liters of dialysis buffer containing 50 mM Tris-HCl pH 7.5, 50 mM NaCl, and 0.1% Triton X-100. Dialysis tubing (approximately 4 inches) was cut for dialysis of volumes no more than 15 mL. No more than two dialysis bags were present per two liters of dialysis buffer. Dialysis was performed at 4 °C with constant stirring with a magnetic stir bar. Dialysis buffer was changed once at 4 h and bags were removed the following day. The dialysate was frozen at −20 °C if purification was not performed immediately following dialysis. Softlink Soft Release Avidin Resin (Promega) was prepared and batch capture purification was performed as per the manufacturer’s instructions. Purified recombinant proteins were dispensed as 1 mL aliquots into 1.5 mL capacity microfuge tubes and frozen at −20 °C.
3.4. Enzyme-Linked Immunosorbent Assay
Sera were collected from adult chickens (n = 8) on a broiler breeder farm to establish a negative baseline cutoff for the ELISA. Eight milliliters of blood was collected per bird using 1 inch 20 gauge needles (PrecisionGlide®, Becton Dikinson and Company, Franklin Lakes, NJ, USA). Five environmental drag swabs were collected for poultry houses (8). Drag swabs were incubated overnight at 42 °C in Tetrathionate broth (Difco), then plated on XLT-4 plates (Difco) and incubated overnight at 37 °C. Salmonella suspect colonies were tested with O serogroup typing antisera (Difco) to identify O serogroup. Multiplex PCR was used to identify H1 and H2 allele types needed to assign serotype [60].
A checkerboard titration was performed to determine the optimal antisera dilution and antigen concentration to use in the ELISA. Ten, 10 week old specific pathogen free (SPF) white leghorn chickens were immunized using 1 inch 20 gauge needles (PrecisionGlide®, Becton Dikinson and Company) with 0.5 mL of S. enterica Enteritidis bacterin (Maine Biological Laboratories, Waterville MN, USA) subcutaneously in the cervical region, while another 10 birds were left unvaccinated, serving as negative controls. Two weeks later, vaccinated birds were boosted using 1 inch 20 gauge needles subcutaneously (PrecisionGlide®, Becton Dickinson and Company) with 0.5 mL of pre-purified S. Enteritidis recombinant H1 g,m flagellin preparation (1.68 mg/mL) in 5 mM biotin elution buffer inthe cervical region. Birds were then bled the following week using 3 inch 20 gauge needles (PrecisionGlide®, Becton Dikinson and Company) via cardiac puncture through the thoracic inlet. Birds were euthanized by cervical dislocation following the procedure.
Antibody titers resulting from S. Enteritidis exposure was determined as follows. One week old, specific pathogen free, white leghorn chickens (n = 5) were orally challenged with 106 CFU S. Enteritidis. Sera were collected 17 and 24 days post challenge. Aliquots were taken from each serumsample, pooled, and determined S. Enteritidis-specific antibody titers by ELISA for the pooled sera.
Fifty microliters of purified recombinant H1 g,m flagellin protein at 250 ng, 500 ng, 750 ng, and 1 µg in carbonate/bicarbonate coating buffer (pH 9.6) (25 mM sodium carbonate, 35 mM sodium bicarbionate) was placed in Costar flat bottom E.I.A./R.I.A 8 well strips (Costar Corporation, Cambridge, MA, USA). Plates were wrapped in Saran-Wrap® and incubated overnight at 4 °C. Plates were then washed once with PBS with 0.05% Tween 20. Fifty microliters of conjugate buffer (PBS, 0.05% Tween 20, 5% powered skim milk) was added to each well and serum was added in a serial dilution to create a 10-fold range from 1:10 to 1:100,000 in triplicate. Plates were incubated at 37 °C for 45 min then washed three times with PBS containing 0.05% Tween 20. Fifty microliters of anti-chicken horseradish peroxidase conjugate (Sigma) diluted to 1:10,000 was then added to each well and incubated at room temperature for 45 min. Plates were washed four times with PBS with 0.05% Tween 20. HRP color substrate, O-phenyl-diamine (5 mg per mL) (Sigma), solution was added and wells incubated for 10 min at room temperature to develop color. The reaction was terminated with 12.5 µL, 40% HCl and the OD was read at 490 nm.
4. Conclusions
This study provides a quick and easy serological method for screening breeder flocks of poultry for common Salmonella serotypes responsible for human foodborne outbreaks in the United States. Current serological methods lack the specificity to determine the serotype(s) of Salmonella due to common cross reactivity of antigens prepared for the tests. These cross reactivity issues can be reduced by focusing on the combination of phase 1 (H1) and phase 2 (H2) of the flagellar antigens of Salmonella serotypes responsible for the majority of foodborne outbreaks attributed to poultry. One can determine whether breeders are likely to be transmitting Salmonella serotypes of public health concern to their progeny which are consumed by humans. Poultry companies will be able to use this test to help monitor their flocks and determine immunological status if vaccinated [6].
The prevalence of Salmonella in poultry has already been decreased due to the regulatory actions and implementations of interventions shown to be effective at reducing Salmonella in poultry [5–7].
Accurate and quick tests are essential to maintain the high quality of food safety standards set by government regulations. The serotype-specific serological ELISA described in this study will help improve current Salmonella screens by implementing a quick and accurate procedure while possibly expanding its application to monitoring broiler chickens and other poultry species.
One advantage of using protein-fusion technology to produce antigens for ELISA is its amenability to large scale fermentation. One liter of an induced E. coli clone, produced 16 mg of recombinant antigen following extraction, purification, and dialysis. Using 500 ng of recombinant flagellin per well, a one liter culture can produce enough antigen for ~300, 96-well ELISA plates. With the ease in cloning and expressing the various Salmonella flagellin alleles, a broad-Salmonella serotype-specific ELISA screen can be quickly developed, commercialized, and applied towards the detection of important, pathogenic Salmonella serotypes.
Acknowledgments
This work was supported by a grant from the U.S. Poultry and Egg Association.
This article was originally published in Agriculture2013, 3, 381-397; doi:10.3390/agriculture3030381. This is an Open Access article distributed under the terms and conditions of the Creative Commons Attribution license (http://creativecommons.org/licenses/by/3.0/).