Dietary Thr may be particularly important to the mucus layer because of the importance that Thr has as a structural component of mucin proteins. Threonine and serine (Ser) play crucial roles linking carbohydrate groups through ester linkages to the mucin backbone (Montagne et al. 2004). Lein et al. (1997) reported that Thr and Ser represented approximately 40% of the total endogenous AA losses in pigs fed protein-free diets, with mucin being the major source of AA excretion. Due to the high prevalence of Thr in the mucin molecule, mucin synthesis may be particularly sensitive to a Thr deficiency (Faure et al. 2005, 2006). Threonine represents approximately 40% of the mucus protein structure (Carlstedt et al. 1993). Horn et al. (2009) showed that chicks fed a Thr-deficient diet had reduced crude mucin excretion, although MUC2 mRNA abundance and goblet cell density were not affected, whereas ducklings fed Thr deficient diets had a reduction in crude mucin excretion and MUC2 mRNA abundance. Faure et al. (2005) reported that growing rats fed 30% of the recommended level of dietary Thr exhibited reduced mucin synthesis, although mucosal protein synthesis was not reduced. Changes in mucin thickness or carbohydrate structure may alter the viscosity of the mucus layer, leading to changes in nutrient transport to the enterocyte and furthermore altering nutrient digestibility. Corzo et al. (2007) showed that broilers reared with recycled litter responded to increased dietary Thr, suggesting that microbial-derived changes to mucin influence endogenous Thr losses. In addition to the importance of threonine on mucin dynamics, threonine also plays a critical role with regard to intestinal mucosa and pancreatic digestive enzymes. Approximately 62% of threonine is retained by the gut, with approximately 90% of the retained threonine being utilized by enterocytes (Stoll et al. 2008). Alterations in gut morphology leading to decreased villi surface area could decrease nutrient transport and absorption. A Thr deficiency may lead to a decrease in total protein or mucin synthesis, thereby limiting gut secretions, nutrient breakdown, and nutrient transport across the gut epithelium. Data in the current manuscript are part of a series of research. Growth performance data and diet analyses were presented by Horn et al. (2009). The objective of this study was to investigate the effects of dietary Thr on growth, villi length and width, nutrient digestibility, basal short-circuit current, and glutamine transport in broiler chicks and White Pekin ducklings.
MATERIALS AND METHODS
Experimental Design
One-day-old Ross 308 broiler cockerels were obtained from commercial hatcheries. Birds were reared in heated battery brooders at 35 and 32°C from day 1 to day 7 and from day 8 to day 14, respectively, with continuous lighting and un-restricted access to water. Bird husbandry and handling procedures were approved by the Purdue Animal Care and Use Committee.
For exps. 1 and 2, 72 birds of each species were fed a standard broiler chick starter diet from day 1 to day 14 of age and were weighed, wing-tagged, and assigned to six replicate cages of four birds per dietary treatment on day 14. The 18 groups of birds per species were blocked on the basis of weight; each block of similar weights was randomly assigned to each diet in a randomized complete block design. The dietary treatments consisted of semipurified, isonitrogenous corn-soybean mealbased diets with the addition of crystalline AA and graded concentrations of dietary Thr in exps. 1 and 2 (Table 1). For each experiment, a basal diet was initially mixed containing all constant dietary components. Variable ingredients (Thr, Gln, and corn starch) were subsequently added for each diet. Supplementation of Gln served to make the diets isonitrogenous and corn starch served as a filler. Dietary treatments contained approximately 40% of the Thr requirement (3.3 g of Thr kg-1), 70% of the Thr requirement (5.8 g of Thr kg-1) or 100% of the Thr requirement (8.2 g of Thr kg-1) based on National Research Council requirements for broiler chicks (NRC 1994). Chromic oxide served as an indigestible marker. Birds were killed by CO2 asphyxiation at the end of the feeding trial. Individual bird weight and cage feed intake were recorded at the end of the trial.
For exps. 3and 4, 40 birds of each species were fed a standard broiler chick starter diet from day 1 to day 14 of age. On day 14, birds were weighed, wing-tagged, and divided into groups. Twenty replicate cages of birds per species were assigned to each dietary treatment with one bird per cage in a completely randomized design. Battery cage set-up allowed for visual and near physical contact with other birds of their specie. The dietary formulations were similar to that of exps. 1 and 2 (Table 1). Dietary treatments contained approximately 40% of the Thr requirement (3.3 g of Thr kg-1) or 100% of the Thr requirement (8.2 g of Thr kg-1) based on the NRC requirements for broiler chicks (NRC 1994). Birds were fed dietary treatments from 14 to 28 d of age. Ten replicates per species were killed by CO2 asphyxiation at the end of the experiment. Bird weight and feed intake were recorded weekly.
Table 1. Ingredient composition of the experimental basal diet on an as fed basisz
Analyses
Diets were analyzed for crude protein, P, Ca, and AA and met or exceeded formulated values. Variability of up to 15% between formulated and analyzed AA between diets was accepted. Total P was determined using ammonium molybdate according to Onyango et al. (2004). Dietary Ca was determined using flame atomic absorption spectroscopy (Varian FS240 AA, Varian Inc., San Palo, CA), whereas N was determined by the combustion method (LECO Model 2000 CHN, St. Joseph, MI). Amino acid analyses were conducted at the University of Missouri Experiment Station Chemical Laboratory (Columbia, MO 65211-71701). Amino acid samples were prepared using acid hydrolysis, 6 M-HCl at 110°C for 24 h under an N atmosphere. Amino acid hydrosylates were determined using HPLC after post-column derivatization [Association of Official Analytical Chemists (2000) method 994.12]. Ingredient composition and analyzed nutrients are presented in Tables 1 and 2, respectively.
Table 2. Analyzed concentration of nutrients fed to broiler chicks and White Pekin ducklings
Excreta were collected on the last 2 d of each experiment and immediately frozen. Subsequently, excreta were lyophilized (Unitop 600L, Virtis, Gardiner, NY), and ground through a 0.5-mm screen (Retsch ZM 100, GmbH & Co., K.C., Germany). Samples of excreta were dried (Precision Scientific Co., Chicago, IL) at 100°C for 24 h for determination of moisture content. Chromium concentration in feed and excreta was determined using methods described by Fenton and Fenton (1979). Excreta samples were digested in nitric and 70% perchloric acid for solubilization of minerals. Total tract retention was calculated using the formula:
ND = [1-(Ci.Co-1 x No.Ni-1)] x 100
where ND represents percent nutrient retention; Ci and Co are concentration of chromium in the diet or excreta, respectively; Ni and No are concentration of nutrient in the diet or excreta, respectively.
Intestinal Morphology
Samples of jejunum from six birds per dietary treatment per species from exps. 1 and 2 were collected after euthanasia. The bird with the median body weight per cage was selected (95% of average body weight). A 10-cm segment was cut from the jejunum using a razor blade and placed in 10% buffered formalin for 48 h, dehydrated with ethanol, cleared with Sub-X® (Polysciences, Inc., Warrington, PA) and placed in paraffin (Polyfin paraffin, Sigma Polysciences, St. Louis, MO). Sections were stained with Alcian Blue and Periodic acid-Schiff reagent (Luna 1968). Briefly, tissues were cleared and hydrated, and stained with Alcian Blue solution (1 g Alcian Blue, 3mL L-1 acetic acid, 97 mL distilled water, pH 2.5) for 30 min to stain for mucincontaining goblet cells. Tissues were then rinsed in tap water for 10 min and oxidized in periodic acid (5 g L-1) for 5 min, rinsed in lukewarm tap water for 10 min, and stained in Coleman's Schiff reagent as a counter stain (Sigma Polysciences, St. Louis, MO) for 10 min. Villi length and width were measured from five villi per bird and the means were analyzed for differences. Villi length is defined as the length from the villus tip to the valley between villi. Villi width is defined as the width at the midpoint between the villus crypt and the villus tip.
Tissue Preparation for Nutrient Transport
The distal duodenum was removed for analysis of basal current and glutamine response by modified Ussing Chambers (VCC MC8, Physiologic Instruments Inc., San Diego, CA) from one bird per cage on day 20 or 21 of exps. 1 and 2. The bird with a median body weight (95% of average body weight) per cage was selected. The tissue was placed directly into oxygenated Ringer's Buffer solution, which contained (mmol L-1) CaCl2, 1.2; MgCl2, 1.2; Na2HPO4, 2.4; NaH2PO4, 0.4; NaHCO3, 25; KCL, 5.0; and NaCl 115, at a pH of 7.4. Subsequently, two 3-cm sections were taken from each duodenum. Serosa was retained, excess fat was removed from the tissue and segments were cut open, exposing the lumen, and washed with cold Ringer's solution. Each segment was mounted on the Ussing Chambers maintained at 40°C, and continuously stirred and oxygenated by 95% O2 and 5% CO2.
Electrical and Permeability Measurements
The Ussing Chambers were connected to dual voltage clamps and were allowed to equilibrate for 15 min before measurement of a glucose response. After the tissues were allowed to stabilize, 40 mL of a 1 M solution of glucose and mannitol was added to measure the glucose response and ensure viability of the tissue segment. Subsequent to the addition of glucose, the mucosal reservoir was emptied and refilled with Ringer's solution. Basal transmucosal short-circuit current (Isc) and glutamine transport were measured. Basal Isc is the measure of the flow of ions per unit time when the tissue transepithelial voltage is 0 mV. Glutamine was added to the brush border membrane and active transport was measured by the change in Isc per square centimeter. Experiments were conducted within 20 min of bird euthanasia.
Statistical Analysis
Data were analyzed using GLM procedures of the SAS Institute, Inc. (2004) appropriate for randomized complete block design (exps. 1 and 2) or completely randomized design (exps. 3and 4). Linear and quadratic contrasts were used for comparing the concentration of dietary Thr on growth performance, nutrient retention, nutrient transport, and gut morphology for exps. 1 and 2. Bird cage served as the experimental unit. Outliers were determined by examination of the studentized residuals in regression procedures of SAS software. Data normality was assessed by univariate procedures of SAS software (SAS Institute, Inc. 2004). The level of significance was P<0.05 and a trend was defined as 0.05<PB<0.10.
RESULTS
Supplementation of dietary Thr increased growth in broiler chicks in exp. 3, but not in exp. 1, and in ducklings in exps. 2 and 4 (Tables 3and 4). Body weight gain was numerically, but not significantly. increased from 43to 70 g for chicks fed dietary treatments for 7 d, whereas gain increased (PB0.001) from 31 to 198 g for chicks fed dietary treatments for 14 d as dietary Thr increased from 3.3 to 8.2 g kg-1. Dietary Thr did not affect feed intake (FI) for the 7- or 14-d feeding trials for chicks. For ducklings, gain increased (PB0.02) from -18 to 158 g and from -3to 564 g for birds fed dietary treatments for 7 or 14 d, respectively, as dietary Thr increased from 3.3 to 8.2 g kg-1. Furthermore, FI was increased (PB0.01) from 282 to 487 and from 663to 1667 g in ducklings fed dietary treatments for 7 or 14 d, respectively, as dietary Thr increased from 3.3 to 8.2 g kg-1. There were no mortalities for these experiments.
Table 3. Growth performance in broiler chicks and White Pekin ducklings fed graded concentrations of dietary threonine for exps. 1 and 2z,y,x
Table 4. Growth performance in broiler chicks and White Pekin ducklings fed low or adequate dietary threonine for exps. 3 and 4 z,y,x
Villi length and width were not affected by increasing dietary Thr for chicks or ducklings. The average villi length was 722.9 and 545.5 mm for chicks and ducklings, respectively. The average villi width was 127.0 and 127.2 mm for chicks and ducklings, respectively.
Nutrient retention data for exps. 3and 4 are presented in Table 5. In the broiler chick study (exp. 3), DM and N retention increased (PB<0.05) as dietary Thr increased from 3.3 to 8.2 g kg-1. Nitrogen retention increased (P=0.05) from 46.6 to 60.9% as dietary Thr increased from 3.3 to 8.2 g kg-1 in ducklings for exp. 4.
Table 5. Dry matter, N, P, and Ca retention in broiler chicks and White Pekin ducklings fed approximately low or adequate dietary threonine for exps. 3 and 4 z,y,x
Basal short circuit current and glutamine transport data are presented in Table 6. There was no effect of dietary treatment on basal current or glutamine transport in chicks or ducklings. Average basal current was 3.41 or 1.77 mA cm-2, whereas glutamine transport was 0.65 or 2.10 mA cm-2 for chicks and ducklings, respectively.
DISCUSSION
Threonine plays a critical role in the structure of mucus proteins and digestive enzymes. Because a Thr deficiency has been shown to alter mucin dynamics in poultry (Horn et al. 2009), there may be implications for nutrient absorption and overall gut health. Threonine retention (82%) tends to be higher when compared with other essential AA such as isoleucine (61%), methionine (68%) or valine (61%) in broiler chickens (Baker 1991; Edwards et al. 1996, 1999; Baker et al. 1997). In the current studies, a Thr deficiency was used to investigate the effects Thr may have on nutrient digestibility, absorption, and gut health in broiler chicks and White Pekin ducklings. Few studies exist in terms of Thr and nutrient digestibility; therefore, direct comparisons are severely limited.
Table 6. Basal short-circuit current and glutamine transport in broiler chicks and White Pekin ducklings fed graded concentrations of dietary threonine in exp. 1 and 2 z,y,x,w
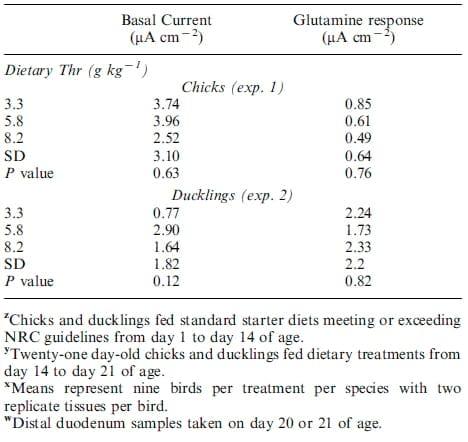
Dietary Thr clearly has implications for growth in poultry (Davis and Austic 1982; Smith and Waldroup 1988; Webel et al. 1996). For the current study, also discussed in Horn et al. (2009), increasing dietary Thr from approximately 40% (3.3 g kg-1) of the Thr requirement to adequate concentrations (8.2 g kg-1) increased gain in broiler chicks and ducklings over the 7- or 14-d feeding trials. Furthermore, feed intake was depressed when dietary Thr was decreased in broiler chicks and ducklings. A wide range of studies exists in the literature examining the effect of dietary Thr on growth, although to the authors' knowledge no studies exist examining the effects of Thr and growth in ducklings. Smith and Waldroup (1988) reported that increasing total dietary Thr from 5.9 to 8.7 g kg-1 in chicks fed semipurified sorghum-based diets increased gain by 28 g in 3-wk-old broilers fed the dietary treatments for 14 d. Furthermore, Webel et al. (1996) reported that increasing digestible Thr from 4 to 7 g kg-1 increased gain from 31.1 to 71.2 g d-1 in broilers fed diets from 3to 6 wk of age. Gain was more drastically affected in ducklings when compared with chicks, which is most likely due to differences that exist in rate of growth and digestive tract development between ducklings and chicks. During the first 3wk post-hatch, ducklings grow at a rate approximately double that of broiler chicks (NRC 1994). Plausibly, developmental differences exist in the digestive tract between ducklings and chicks; therefore, the Thr requirement of broiler chicks may not be applicable to ducklings (Jamroz et al. 2002, 2004; Kluth and Rodehutscord 2006). Jamroz et al. (2004) reported pancreatic enzyme activity in waterfowl to be lower during the first 2 wk of age when compared with broiler chicks. These results suggest slower pancreatic development in waterfowl when compared with chicks. Furthermore, Kluth and Rodehutscord (2006) reported lower CP and AA digestibilities in ducklings compared with broiler chicks and turkeys. In the current study, FI was depressed as dietary Thr decreased in ducklings, but not in broiler chicks. It is widely accepted that FI is decreased when an AA imbalanced diet is fed (Pond et al. 1995).
In the current study, increasing dietary Thr clearly increased DM digestibility in broiler chicks and ducklings. For broiler chicks, increasing total dietary Thr from 40% of the recommended requirement to approximately adequate (NRC 1994) increased DM digestibility by 28 or 12% in chicks fed dietary treatments for 7 or 14 d, respectively. Similarly, increasing dietary Thr increased DM digestibility by 35 or 11% in ducklings fed dietary treatments for 7 or 14 d, respectively. For chicks, increasing dietary Thr from 40% to approximately adequate levels increased P digestibility by 14%, although there was no effect of dietary Thr on Ca or N digestibility. Furthermore, for ducklings, increasing dietary Thr from 40% to adequate increased N digestibility by 23%, although there was no effect of dietary Thr on P or Ca digestibility. Changes in nutrient digestibility may be caused by an overall decrease in gut protein synthesis, resulting in decreased villi surface area, ultimately decreasing nutrient transport and absorption. Dozier et al. (2001) reported an improvement in nitrogen retention and recovery of AMEn in male broiler chicks receiving adequate dietary Thr in comparison to broiler chicks that were fed Thr deficient diets suggesting a link between nutrient recovery and dietary Thr. Alterations in mucin viscosity could have implications for active transport of ions across the gut epithelium. Furthermore, a Thr deficiency may specifically inhibit mucin synthesis, altering mucin viscosity, and decreasing overall nutrient transport to the enterocyte (Montagne et al. 2004). Threonine is a major component of some digestive enzymes. The Thr content of pancreatic digestive enzymes is particularly high (Block et al. 1966) and a Thr deficiency could alter enzyme synthesis, reducing N and energy recovery.
A Thr deficiency did not alter jejunum morphology in chicks or ducks for the current experiment. The values for jejunum length and width were similar to those reported in the literature for chicks. For example, Awad et al. (2004) reported 1064 mm and 175 mm for average villi length and width, respectively, in the jejunum of 6-wk-old broiler chicks. Although there is a dearth of information about duckling gut morphology, Watkins et al. (2004) reported an average villus height of 850 mm in the duodenum of 3-wk-old Pekin ducklings fed standard wheat-based starter diets. Slightly lower values reported for the current study are likely due to differences in bird age, dietary ingredients, and experimental design and methodology. The mechanism in which Thr may alter the villus growth dynamics may be twofold. First, the reduction in FI and gut fill associated with an AA deficiency may have implications for villus surface area. Additionally, because Thr serves as a key component of enzyme and mucin structure, a deficiency may alter protein synthesis in the gut.
For the current study there were no differences in villi length in broiler chicks or ducklings. Enterocyte turnover takes approximately 4 to 6 d in poultry species. A 7-d experimental period may not have allowed sufficient time to elucidate difference in villus characteristics for the current trial. For the current experiment, increasing dietary Thr did not alter basal short-circuit current or glutamine transport in chicks or ducklings. Basal short-circuit current is an estimation of the summation of all active ion transport. A decrease in basal short-circuit current may be indicative of a less viable intestinal tissue. A change in glutamine absorption could be indicative of altered AA uptake through Na-dependant AA transporters in the brush border membrane of intestinal tissues. The average initial short-circuit current values from the current experiment (3.41 mA cm-2 for chicks; 1.77 mA cm-2 for ducklings) are lower, but comparable with Grub et al. (1987). Grub et al (1987) reported ileal short-circuit current was 33 mA cm-2 in 6- to 8-wk-old chickens. The values for glutamine transport from the current experiment are lower, but comparable with Rehman et al. (2007). Rehman (2007) found the glutamine active transport in 5-wk-old broilers to be 19 mA cm-2.
A Thr deficiency could decrease nutrient digestibility and absorption by overall suppression of protein synthesis. An overall reduction of protein synthesis at the level of the gut would result in a decrease in digestive enzymes, mucus proteins, nutrient transporters and villus surface area. Dietary Thr and AA may be especially important for gut growth and development in young growing animals. Stoll et al. (1998) found that approximately 60% of dietary Thr is utilized for protein synthesis in the gut in milk-fed piglets. Glutamine, arginine, and proline also play important roles in GIT growth and development and exemplify the importance of sufficient dietary AA. It is well established that glutamine increases cell proliferation, gene transcription, and protein synthesis in the GIT of growing animals (Burrin and Stoll, 2002). Furthermore, arginine serves a crucial role in growing animals by maintaining mucosal blood flow and enhancing immune function (Blachier et al. 1995; Wallace and Miller 2000). A Thr deficiency may suppress overall mucosal growth and maintenance, reducing villus surface area. A reduction in villus surface area could lead to a decrease in nutrient absorption. Czernichow et al. (1992) reported a decrease in jejunal mucosal weight in rats enterally fed an AA mixture when compared with rats enterally fed lipids and carbohydrates, or parenterally fed. Furthermore, jejunal villi length was greater in rats supplemented enterally with AA when compared with rats parenterally fed. In addition to affecting gut morphology, AA may be involved in secretion of intestinal hydrolytic enzymes (Kimura et al. 1976; McCarthy et al. 1980; Czernichow et al. 1992). Czernichow et al. (1992) reported that enteral supplementation of AA increased sucrase and aminopeptidase activity in the rat jejunum when compared with rats enterally supplemented with lipids and carbohydrates and rats fed parenterally. The increase in specific brush border enzymes due to the supplementation of AA could be due to a signaling pathway in which brush border enzymes are synthesized due to the presence of AA, or to an increase in uptake of AA in the enterocyte used to synthesize brush border enzymes.
In addition to a suppression of overall protein synthesis in the gut, it may be possible that mucin viscosity is altered in birds during periods of Thr deficiency. Intestinal mucins are especially rich in dietary Thr, and may be sensitive to Thr deficiency. Threonine makes up approximately 11% of the mucin molecule in humans and serves to link mucin carbohydrates to the mucin AA backbone (Gum et al. 1992). In addition to protective functions, mucin serves as a selective diffusion barrier, and has implications on nutrient absorption. Faure et al. (2005) reported that a 30% Thr deficiency significantly decreased mucin synthesis, although mucosal protein synthesis was not altered in rats. Changes in mucin dynamics could alter nutrient absorption, and have broad applications in terms of overall gut health. Although, traditionally increasing mucin thickness is attributed to decreasing nutrient transport the gut wall, it may be possible that a decrease in mucin production leads to a more viscous mucin layer. Previous studies have suggested that as mucin increases in viscosity, the carbohydrate structure is altered (Mortazavi and Smart 1994). Furthermore, it has been suggested that as mucin carbohydrate structure increases in density, the mucus layer becomes increasingly adhesive and gel-like (Forstner et al. 1984; Girod et al. 1992; Mortazavi and Smart 1994). A decrease in nutrient digestibility in Thr-deficient animals could be the result of a more viscous mucus layer, altering nutrient transport from the lumen to the enterocyte.
Threonine may have implications on gut health and nutrient digestibility in broiler chicks and White Pekin ducklings. Results from the present studies show that a Thr deficiency over a 7- or 14-d feeding trial decreases growth and DM digestibility. In chicks, P digestibility is decreased as dietary Thr decreases, whereas in ducklings, N digestibility decreases as dietary Thr decreases. Dietary Thr may have implications on nutrient absorption due to changes in gut protein synthesis, although morphological changes were not observed in the present experiment. Further research is needed to understand how AA, in particular Thr, affect gut morphology, digestive enzymes, transporters, and mucin viscosity in the GIT of poultry.
ACKNOWLEDGEMENTS
The authors express their appreciation to Midwest Poultry Consortium, 4630 Churchill Street, St. Paul, MN, and Maple Leaf Farms, Syracuse, IN, for their support of this research, Jason Fields for set-up and maintenance of animal holding facilities, Pat Jaynes for technical assistance with histological measurements, and Blaire Aldridge for technical assistance with gut permeability measurements.
Association of Official Analytical Chemists. 2000. Post-column ninhydrin derivation. Method 994.12. AOAC, Arlington, VA.
Awad, W. A., Bohm, J., Razzazi-Fazeli, E., Hulan, H. W. and Zentek, J. 2004. Effects of deoxynivalenol on general performance and electrophysiological properties of intestinal mucosa of broiler chickens. Poult. Sci. 83: 19641972.
Baker, D. H. 1991. Partitioning of nutrients for growth and other metabolic functions: efficiency and priority considerations. Poult. Sci. 70: 17971805.
Baker, D. H., Fernandez, S. R., Parsons, C. M., Edwards H. M. III, Emmert, J. L. and Webel, D. M. 1997. Maintenance requirement for valine and efficiency of its use above maintenance for accretion of whole body valine and protein in young chicks. J. Nutr. 126: 18441851.
Blachier, F., Selamnia, M., Robert, V., Touil, H. and Duee P. H. 1995. Metabolism of L-arginine through polyamine and nitric oxide synthase pathways in proliferative or differentiated human colon carcinoma cells. Biochem. Biophys. Acta. 1268: 255262.
Block, R. J., Wiess, R. W. and Cornett, D. B. 1966. The amino acid composition of proteins. Pages 149295 in R. J. Block and D. Bolling, eds. The amino acid composition of proteins and feeds. Charlets C. Thomas Publisher, Springfield, IL.
Burrin, D. G. and Stoll, B. 2002. Key nutrients and growth factors for the neonatal gastrointestinal tract. Clin. Perinatol. 29: 6596.
Carlstedt, I., Herrmann, A., Karlsson, H., Sheehan, J., Fransson, L. A. and Hansson, G. C. 1993. Characterization of two different glycosylated domains from the insoluble mucin complex of rat small intestine. J. Biol. Chem. 268: 1877118781.
Corzo, A., Kidd, M. T., Dozier, W. A. III, Pharr, W. T. and Koutsos, E. A. 2007. Dietary threonine needs for growth and immunity of broilers raised under different litter conditions. J. Appl. Poult. Res. 16: 574582.
Czernichow, B., Galluser, M., Hasselmann, M., Doffoel, M. and Raul, F. 1992. Effects of amino acids in mixtures given by enteral or parenteral route on intestinal morphology and hydrolases in rats. J. Parenter. Enteral. Nutr. 16: 259263.
Davis, A. T. and Austic, R. E. 1982. Threonine metabolism of chicks fed threonine-imbalanced diets. J. Nutr. 112: 21772186.
Dozier, W. A. III, Moran, Jr., E. T. and Kidd, M. T. 2001. Male and female broiler responses to low and adequate dietary threonine on nitrogen and energy balance. Poult. Sci. 80: 926930.
Edwards, H. M. III and Baker, D. H. 1999. Maintenance sulfur amino acid requirements of young chicks and efficiency of their use for accretion of whole body sulfur amino acids and protein. Poult. Sci. 78: 14181423.
Edwards, H. M. III, Baker, D. H., Fernandez, S. R. and Parsons, C. M. 1996. Maintenance threonine requirement and efficiency of its use for accretion of whole body threonine and protein in young chicks. Br. J. Nutr. 78: 111119.
Edwards, H. M. III, Fernandez, S. R. and Baker, D. H. 1999. Maintenance lysine requirement and efficiency of using lysine for accretion of whole-body lysine and protein in young chicks. Poult. Sci. 78: 14121417.
Faure, M., Mettraux, C., Moennoz, D., Godin, J. P., Vulchoud, J., Rochat, F., Breuille, D., Obled, C. and Corthesy-Theulaz, I. 2006. Specific amino acids increase mucin synthesis and microbiota in dextran sulfate sodium-treated rats. J. Nutr. 136: 15581564.
Faure, M., Moennoz, D., Montigon, F., Mettraux, C., Breuille, D. and Ballevre, D. 2005. Dietary threonine restriction specifically reduces intestinal mucin synthesis in rats. J. Nutr. 135: 486491.
Fenton, T. W. and Fenton, M. 1979. An improved procedure for the determination of dietary chromic oxide in feed and feces. Can. J. Anim. Sci. 59: 63 1634.
Forstner, J., Wesley, A., Mantle, M., Kopelman, H., Man, D. and Forstner, G. 1984. Abnormal mucus: Nominated but not yet elected. J. Pediatr. Gastroenterol. Nutr. 3. Suppl. 1: S6773.
Girod, S., Zahm, J. M., Plotkowski, C., Beck, G. and Puchelle, E. 1992. Role of the physiochemical properties of mucus in the protection of the respiratory epithelium. Eur. Resp. J. 5: 477474.
Girod, S., Zahm, J. M., Plotkowski, C., Beck, G. and Puchelle, E. 1992. Role of the physiochemical properties of mucus in the protection of the respiratory epithelium. Eur. Resp. J. 5: 477474.
Grub, B. R., Driscoll, S. M. and Bentley, P. J. 1987. Electrical pd, short-circuit current and fluxes of Na and Cl across avian intestine. J. Comp. Physiol. 157: 181186.
Horn, N. L., Donkin, S. S., Applegate, T. J. and Adeola, O. 2009. Intestinal mucin dynamics: response of broiler chicks and White Pekin ducklings to dietary threonine. Poult. Sci. 88: 19061914.
Jamroz, D., Wertelecki, T., Wiliczkiewicz, A., Orda, J. and Skirupinska, J. 2004. Dynamics of yolk sac resorption and post-hatching development of the gastrointestinal tract in chickens, ducks and geese. J. Anim. Phys. Anim. Nutr. 88: 239250.
Jamroz, D., Wiliczkiewicz, A., Orda, J., Wertelecki, T. and Skorupinska, J. 2002. Aspects of development of digestive activity of intestine in young chickens, ducks and geese. J. Anim. Phys. Anim. Nutr. 86: 3 53366.
Kimura, T., Shiosaka, S. and Sakaibara, A. 1976. Effect of dietary amino acids and food intake on intestinal sucrase and leucine aminopeptidase activities in rats. Nutr. Rep. Int. 14: 657670.
Kluth, H. and Rodehutscord, M. 2006. Comparison of amino acid digestibility in broiler chickens, turkeys, and Pekin ducks. Poult. Sci. 85: 19531960.
Lein, K. A., Sauer, W. C. and Fenton, M. 1997. Mucin output in ileal digesta of pigs fed a protein-free diet. Z. Ernahrungswiss. 36: 182190.
Luna, L. G. 1968. Manual of histologic staining methods of the Armed Forces Institute of Pathology. Armed Forces Institute of Pathology, New York, NY.
McCarthy, D. M., Nicholson, J. A. and Kim, Y. S. 1980. Intestinal enzyme adaptation to normal diets of different composition. Am. J. Physiol. 239: G445451.
Montagne, L., Piel, C. and Lalles, J. P. 2004. Effect of diet on mucin kinetics and composition: nutrition and health implications. Nutr. Rev. 62: 105114.
Mortazavi, S. A. and Smart, J. D. 1994. Factors influencing gel-strengthening at the mucoadhesive-mucus interface. J. Pharm. Pharmacol. 46: 8690.
National Research Council. 1994. Nutrient requirements of poultry. 9th rev. ed. National Academy Press, Washington, DC.
Onyango, E. M., Bedford, M. R. and Adeola, O. 2004. The yeast production system in which Escherichia coli phytase is expressed may affect growth performance, bone ash, and nutrient use in broiler chicks. Poult. Sci. 83: 421427.
Pond, W. G., Church, D. C. and Pond, K. R. 1995. Proteins and amino acids. Pages 136147 in Basic animal nutrition and feeding. John Wiley and Sons, New York, NY.
Rehman, H., Rosenkranz, C., Bohm, J. and Zentek, J. 2007. Dietary inulin affects the morphology but not the sodiumdependent glucose and glutamine transport in the jejunum of broilers. Poultry Sci. 86: 11822.
SAS Institute, Inc. 2004. SAS/STAT user's guide. Release 9.1.
SAS Institute, Inc, Cary, NC. Smith, Jr., N. K. and Waldroup, P. 1988. Investigations of threonine requirements of broiler chicks fed diets based on grain sorghum and soybean meal. Poult. Sci. 67: 108112.
Stoll, B., Burrin, D. G., Henry, J., Yu, H., Jahoor, F. and Reeds, P. J. 1998. Dietary amino acids are the preferential source of hepatic protein synthesis in piglets. J. Nutr. 128: 15171524.
Wallace, J. L. and Miller, M. J. 2000. Nitric oxide in mucosal defense: a little goes a long way. Gastroenterology 119: 512520.
Watkins, E. J., Butler, P. J. and Kenyon, B. P. 2004. Posthatch growth of the digestive system in wild and domesticated ducks. Br. Poult. Sci. 45: 3 3 1 341.
Webel, D. M., Fernandez, S. R., Parsons, C. M. and Baker D. H. 1996. Digestible threonine requirement of broiler chickens during the period three to six and six to eight weeks posthatching. Poult. Sci. 75: 12531257.
This article was originally published in the Canadian Journal of Animal Science, 2010, 90(4): 513-520, 10.4141/cjas10024