Introduction
Environmental conditions influence metabolic and developmental processes during the perinatal period of mammals and avian species [1,2,3]. Changes in metabolism and development in this period can have long-term effects in later life [2,4]. Particularly glucose metabolism seems to play a crucial role in survival and early development of animals [1,5,6]. In chicken embryos, several studies have indicated that high environmental temperatures affect glucose metabolism and possibly explain decreases in survival and development in the peri- and posthatch period [3,5,7,8].
During incubation of chicken embryos, nutrient utilization and metabolic pathways depend on the developmental stage of the embryo. Carbohydrates are present in very low levels in the egg (<1%) at oviposition and the total amount decreases even further throughout incubation [9]. A major portion of the glucose is used in the first week of incubation for anaerobic glycolysis, as the chorioallantoic membrane (CAM) is not sufficiently developed to provide enough oxygen for complete fatty acid oxidation [10,11]. Fatty acids are the predominant energy source of chicken embryos during the major part of incubation, but glucose becomes an indispensable substrate for ATP production at the end of incubation when the hatching process occurs [12,13]. The hatching process is energy-demanding and, the oxygen supply is restricted by limited gas exchange across the eggshell and CAM at the start of the hatching process [14,15]. Because muscle activity is high and oxygen availability is relatively low, glucose is used for anaerobic glycolysis in muscles, resulting in increased plasma lactate concentrations [11,12,13,16,17]. Plasma glucose concentrations increase just before the hatching process starts, probably to ensure normal activity of the central nervous system [18] and to emerge successfully from the eggshell. This increase in glucose is a result of the mobilization of hepatic glycogen [12,18,19,20]. Because glucose is hardly available in the egg at the start of incubation (<1%) [9], glycogen stores are built up during the incubation period in the heart, liver, muscle, and yolk sac membrane [19,20,21,22].
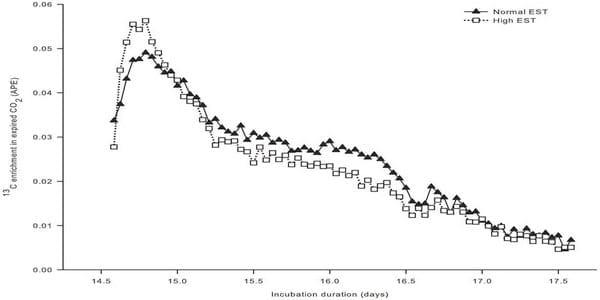
Figure 1. 13C enrichment in expired CO2 in eggs incubated at two different eggshell temperatures (study I). 13C enrichment in expired CO2 after injecting a single bolus of [U-13C]glucose (1.0 mg in 250 µL sterile water) in the chorio-allantoic fluid at day 14.5 of incubation of embryos incubated at a normal (37.8ºC) or high (38.9ºC) eggshell temperature (EST) from day 13 of incubation onward. doi:10.1371/journal.pone.0059637.g001.
We previously showed that hepatic glycogen stores at day 18 of incubation, just before the hatching process started, were reduced by 30% when increasing the eggshell temperature (EST) from 37.8 to 38.9ºC from day 7 of incubation onward [3]. These lower hepatic glycogen levels may indicate that hepatic glycogen synthesis was lower and/or that glucose oxidation was higher in embryos incubated at high compared with normal EST.
In the current study we investigated the hypothesis that glucose oxidation is increased when chicken embryos are incubated at a high compared to normal EST. The chicken embryo is a good model to study effects of environmental conditions on metabolic processes and development in the perinatal period, because it grows without direct maternal influences. In mammals, such studies are usually complicated because the fetus is connected to and influenced by the mother. In the current study, a novel approach was developed in a series of experiments to quantify glucose partitioning between anabolic and catabolic processes during late embryonic development in the avian embryo. Chicken embryos, incubated at a normal or high EST, were injected with [U-13C]glucose in the chorio-allantoic fluid at day 17.5 of incubation. The metabolic fate of labeled glucose was assessed by measuring 13C recovery in expired CO2, plasma glucose and lactate, and hepatic glycogen.
Methods
Design and Measurements Study I and II
Two studies were conducted to develop the technique to measure oxidation of [U-13C]glucose after injection into the chorio-allantoic fluid of chicken embryos. In addition, a contrast in EST was created in each of the two studies to provide general insight into the effects of environmental temperature on glucose oxidation in chicken embryos in the last week of incubation. For both studies, 150 fertile broiler eggs at day 13 of incubation were obtained from a Ross 308 flock of 59 weeks of age (Morren B.V., Lunteren, the Netherlands). Eggs were randomly divided between two identical open-circuit climate respiration chambers (CRC) [23] and incubated at a normal (37.8ºC; n= 75) or a high (38.9ºC; n =75) EST. The normal and high EST values were based on studies [24,25], because these studies showed that an EST of 38.9ºC negatively influenced hatchability and body development. Eggshell temperature was regulated in the CRC as described by [23,25] and relative humidity was maintained at 55%. Eggs were turned each hour over 90º. In study I, a solution containing [U-13C]glucose (99 atom% 13C, Sigma-Aldrich Chemie B.V., Zwijndrecht, the Netherlands; 1.0 mg in 250 µL of sterile water) was injected as a single bolus into the chorio-allantoic fluid in each of the 150 eggs at day 14.5 of incubation. Eggs at the normal EST treatment were injected on average 1.5 hours earlier than eggs at the high EST treatment. In study II, a solution containing [U-13C]glucose (Sigma-Aldrich Chemie B.V.; 0.73 mg in 250 mL of sterile water) was repeatedly injected as a bolus into the chorioallantoic fluid in each of the 150 eggs for 4 consecutive days from day 14.5 of incubation onward.
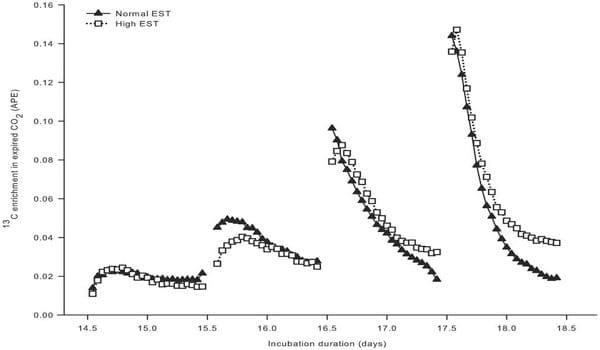
Figure 2. 13C enrichment in expired CO2 of embryos incubated at two different eggshell temperatures (Study II). 13C enrichment in expired CO2, after injecting a single bolus of [U-13C]glucose (0.73 mg in 250 µL sterile water) for four consecutive days from day 14.5 of incubation in the chorio-allantoic fluid of embryos incubated at a normal (37.8ºC) or high (38.9ºC) eggshell temperature (EST) from day 13 of incubation onward. doi:10.1371/journal.pone.0059637.g0020
For in ovo [U-13C]glucose injection, the air cell was located by candling the egg. The blunt end of each egg was sterilized with 70% ethanol. A 20-gauge needle was punctured through the eggshell at 2 to 3 mm above the air cell. A 25-gauge needle was inserted for ±7 mm through the hole to enter the chorioallantoic fluid and to inject the [U-13C]glucose solution. The injection hole was sealed with liquid paraffin. In both studies, total CO2 and 13CO2 production were measured every 6 minutes as described by [26] from day 13 until 18 of incubation. 13C enrichment in expired CO2 was expressed as a percentage of total CO2 production (atom% 13C). The natural background of 13C in expired CO2 was measured during 1.5 days prior to the [U-13C]glucose injection. Background 13C enrichment was subtracted from 13C enrichment and expressed as atom percentage excess (APE).
Design Study III
The main study was designed to investigate effects of environmental temperature on glucose metabolism in chicken embryos during the perinatal period. A normal (37.8ºC) or high (38.9ºC) EST treatment was applied from day 10.5 until 21.6 of incubation in 4 subsequent batches of 160 eggs each. Fertile broiler eggs at day 10.5 of incubation were obtained from a commercial Ross 308 breeder flock aged between 42 and 44 weeks (Lagerwey B.V., Lunteren, the Netherlands). On day 10.5 of incubation, eggs weighing between 60 and 64 g were selected. Per batch, eggs were randomly distributed between the two opencircuit CRC and incubated at a normal (37.8ºC; n = 80) or a high (38.9ºC; n = 80) EST treatment as described for the first 2 studies.
At day 17.5 of incubation, all eggs were injected with a single bolus of [U-13C]glucose (1.0 mg in 250 µL of sterile water) into the chorio-allantoic fluid using the same method as described for the first two studies. After injection, eggs were transferred from an egg tray to a hatching basket (560x360 mm) in the first three batches and to individual hatching baskets (120x135 mm) in the fourth batch. The hatching baskets were placed in the same CRC and eggs were exposed to the same EST treatment as before; this EST was maintained in the CRC until day 18.5 of incubation. At day 18.5 of incubation, the environmental temperature in the CRC was fixed at the normal or high EST, and the EST was allowed to increase during the remainder of the hatching process. Eggs in the first three batches were recorded by a video camera to determine individual hatching times. The study was approved by the Institutional Animal Care and Use Committee of Wageningen University, the Netherlands.
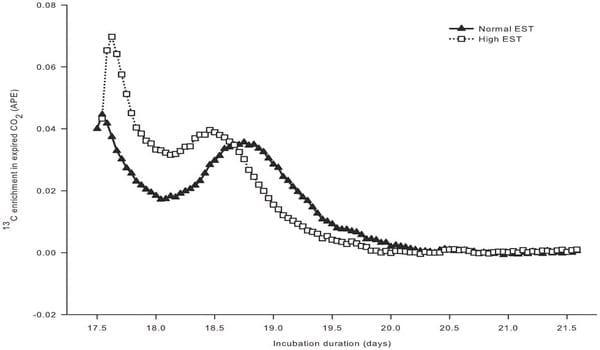
Figure 3. 13C enrichment in expired CO2 of embryos incubated at two different eggshell temperatures (study III). 13C enrichment in expired CO2 after injecting a single bolus of [U-13C]glucose (1.0 mg in 250 µL sterile water) at day 17.5 of incubation in the chorio-allantoic fluid of embryos incubated at a normal (37.8ºC) or high (38.9ºC) eggshell temperature (EST) from day 10.5 of incubation onward. From day 17.6 until 17.8 of incubation, 13C enrichment in expired CO2 was higher for the high EST compared to the normal EST treatment (P,0.05). doi:10.1371/journal.pone.0059637.g003
Measurements Study III
In the first three batches, 13C enrichment in expired CO2 was determined after injection of a single bolus of [U-13C]glucose (99 atom% 13C, Sigma-Aldrich Chemie B.V.; 1.0 mg in 250 µL of sterile water) into the chorio-allantoic fluid at day 17.5 of incubation. In the fourth batch, 13C enrichment in plasma glucose, lactate, and hepatic glycogen were determined at eight time points between day 17.5 and 21.6 of incubation, and chick quality characteristics (yolk-free body mass and yolk weight) and blood metabolites were measured. The injection of the bolus was performed at day 17.5 of incubation to include the energydemanding hatching process which starts around day 19 of incubation, during the period that glucose has an important role as energy substrate [13,27,28]. Based on results of study I and II, it was concluded that glucose oxidation (i.e. 13C enrichment over the background) was detectable for 1 to 2.5 days after tracer administration.
In the first three batches, total CO2 and 13CO2 production were measured at 6 minute intervals as described by [26] from day 10.5 until 21.6 of incubation. The 13C enrichment in excess of the natural background of 13C enrichment in CO2 was calculated from these measurements, as described for study I and II. The background enrichment was determined by calculating the mean 13C enrichment in CO2 from day 10.5 until 17.5 of incubation. 13C enrichment in expired CO2 was expressed per hour of incubation. All chickens were euthanized with CO2 at 21.6 days after the start of incubation.
In the fourth batch, at eight different time points during incubation 10 animals per timepoint and EST treatment were randomly chosen to determine 13C enrichment in plasma glucose, plasma lactate, and hepatic glycogen. Chicken development and blood metabolites were also assessed. The time points were selected to cover the peak in 13CO2 expiration, estimated from the first three batches, and were at day 17.5, 17.8, 18.2, 18.8, 19.7, 20.4, 20.9, and 21.6 of incubation. Embryos or chickens were weighed before blood collection. Blood samples were taken from the jugular vein in embryos and after decapitation in chickens. All blood samples were collected in a 4-mL blood collection tuve containing 10 mg of sodium fluoride and 8 mg of potassium oxalate (BD Vacutainer, Franklin Lakes, NJ). An extra droplet (0.02 mL) of 10% heparin was added to the collection tube prior to sampling. Blood was centrifuged (2,900xg) for 15 minutes and plasma was decanted and stored at -20ºC until further analysis.
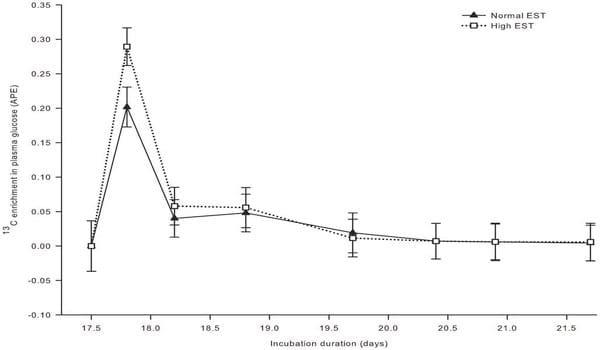
Figure 4. 13C enrichment in plasma glucose of embryos incubated at two different eggshell temperatures (study III). 13C enrichment in plasma glucose after injecting a single bolus of [U-13C]glucose (1.0 mg in 250 µL sterile water) at day 17.5 of incubation in the chorio-allantoic fluid of embryos incubated at a normal (37.8ºC) or high (38.9ºC) eggshell temperature (EST) from day 10.5 of incubation onward. At day 17.5, 17.8, 18.2, and 18.9 of incubation, no chickens were hatched yet. At day 19.7 of incubation, 22% of the chickens of the high EST treatment and 10% of the chickens of the normal EST treatment were hatched. At day 20.4 of incubation, 80% of the chickens of the high EST treatment were hatched and all chickens of the normal EST treatment were hatched. At day 20.9 and 21.6 of incubation, all chickens had been hatched. doi:10.1371/journal.pone.0059637.g004
Plasma glucose, lactate, and uric acid concentrations were determined with commercial kits (DiaSys Diagnostic Systems International, Holzheim, Germany). After bleeding, livers of embryos and chickens were immediately dissected, weighed, and frozen in liquid nitrogen. Livers were stored at -80ºC until further analysis. The yolk was removed and weighed. Yolk-free body mass (YFBM) was calculated as body weight minus yolk weight. YFBM is assumed to reflect chicken development better than body weight because the latter contains an amount of residual yolk that is not metabolized yet [29].
To determine 13C enrichment in plasma glucose [30], 1 mL icecold ethanol (96%) was added to 100 µL of plasma and this mixture was stored overnight at -20ºC before centrifugation (15,339xg) at 4ºC for 20 minutes. After evaporating the supernatant to dryness, 75 µL acetic acid anhydride-pyridine (10:5 vol/vol) was added for 1.5 hours at room temperature to convert glucose to its penta-acetate derivate. The reagent was evaporated to dryness and dissolved in 500 µL chloroform. The 13C/12C ratio was then determined by gas chromatographycombustion isotope ratio mass spectrometry (GC-C-IRMS; Delta C, Finnigan MAT, Bremen, Germany). The measured 13C enrichment was corrected for the dilution of C atoms during derivatization.
13C enrichment in plasma lactate was determined by GC-CIRMS analysis (Delta V Plus; Finnigan MAT, Bremen, Germany) of the 2-O-ethoxycarbonyl ethyl ester of lactic acid. Atomic composition of lactic acid was obtained by correction for carbón added in derivatization using a mass balance equation as previously described [31].
To determine hepatic glycogen enrichment, the whole liver was homogenized with a glass-stirring spoon after the addition of 1 µL of 7% HCLO4/mg of wet tissue. The suspension was centrifuged (2,900xg) at 4°C for 15 minutes. The supernatant was decanted and cleaned with 1 mL of petroleum ether. To extract hepatic glycogen, 2.5 mL ice-cold ethanol (96%) was added to 400 µL supernatant for 120 minutes at -20°C. Then, the solution was centrifuged (2,900xg) at 0ºC for 15 minutes, the fluid was removed and a glycogen pellet was left on the bottom of the tube. The glycogen pellet was dissolved in 1.025 mL water, and the glucose units were released by incubating the solution with a mixture of 25 µL amyloglucosidase (3,200 U/mL; Omnilabo, Breda, the Netherlands), 0.875 mL distilled water and 125 µL 2 M acetatebuffer (2M acetic acid:2M acetate, 4:6 vol/vol; pH = 4.8) at 60ºC for 1 hour. The reaction was finished by placing the tubes on crushed ice, and 100 µL of the mixture was used to determine the 13C/12C ratio in the released glucose units by GC-C-IRMS using the procedure described for plasma glucose enrichment [30]. The measured 13C enrichment was corrected for the dilution of C atoms during the derivatization.
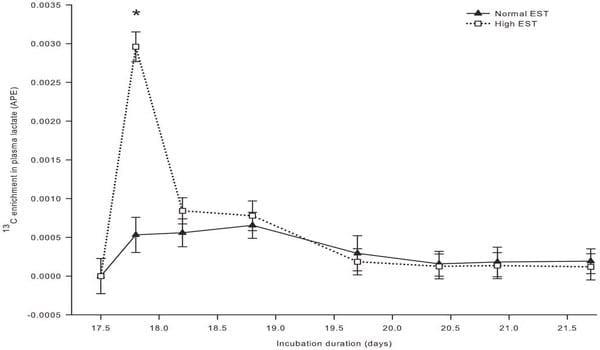
Figure 5. 13C enrichment in plasma lactate of embryos incubated at two different eggshell temperatures (study III). 13C enrichment in plasma lactate after injecting a single bolus of [U-13C]glucose (1.0 mg in 250 µL sterile water) at day 17.5 of incubation in the chorio-allantoic fluid of embryos incubated at a normal (37.8ºC) or high (38.9ºC) eggshell temperature (EST) from day 10.5 of incubation onward. *Significant difference between EST treatments (P,0.05). At day 17.5, 17.8, 18.2, and 18.9 of incubation, no chickens were hatched yet. At day 19.7 of incubation, 22% of the chickens of the high EST treatment and 10% of the chickens of the normal EST treatment were hatched. At day 20.4 of incubation, 80% of the chickens of the high EST treatment were hatched and all chickens of the normal EST treatment were hatched. At day 20.9 and 21.6 of incubation, all chickens had been hatched. doi:10.1371/journal.pone.0059637.g005
The remaining mixture was used to determine the hepatic glycogen concentration. Proteins were precipitated by adding 200 µL of Carrez I (106 g potassium hexacyanoferrate (II) trihydrate dissolved in 1 L water) plus 200 µL of Carrez II (219.5 g zinc acetate dihydrate and 30 g acetic acid dissolved in 1 L water) solution to the mixture. After centrifugation (15,339xg) at 4ºC for 10 minutes, the supernatant was used to determine glucose concentration with a commercial kit (DiaSys Diagnostic Systems International, Holzheim, Germany). The glucose concentrations were corrected with 0.9 to obtain hepatic glycogen concentrations, expressed as mg/g wet tissue.
Enrichment in plasma glucose and lactate was corrected for the natural background that was determined in embryos samples at day 17.5 of incubation i.e. these embryos did not receive [U-13C] glucose, and was expressed in APE. 13C in hepatic glycogen was calculated in mmol over the baseline by multiplying the hepatic glycogen concentration by liver weight and by the 13C enrichment (in APE).
Calculation Recovery Study III
Recovery of 13C in expired CO2 was calculated by expressing 13CO2 production in excess of the background relative to the dose injected at day 17.5 of incubation. Embryos were not included to calculate recovery of the 13C tracer as 13CO2 when they died during incubation (i.e. from the moment of death onwards).
Statistical Analysis
Data from study I and II were not statistically analyzed because these studies were designed to develop the technique to measure [U-13C]glucose oxidation and to provide an initial insight in the effect of EST. Repeated measurements on the same treatment combination were therefore not performed. In the first three batches of study III, 13C enrichment in expired CO2 was calculated per hour and analyzed using the MIXED procedure for repeated measurements in SAS [32] (Version 9.1) with EST, day of incubation, and their interaction as fixed factors. Day of incubation was the repeated factor and an auto-regressive covariance structure was used. All other variables were analyzed using a GLM procedure with EST, day of incubation, and their interaction as fixed factors. Recovery of 13C in expired air was analyzed using a GLM procedure with EST treatment as a fixed effect and batch 1 to 3 as a block. CRC was the experimental unit in batches 1 to 3 and embryo or chicken was the experimental unit in batch 4. Distributions of the means and residuals were examined to check model assumptions. Least squares means were compared using Bonferroni adjustments for multiple comparisons. Data are presented as least squares means ±SEM. In all cases, a significant difference was considered at P≤0.05 and a tendency was considered at 0.05<P<0.10.
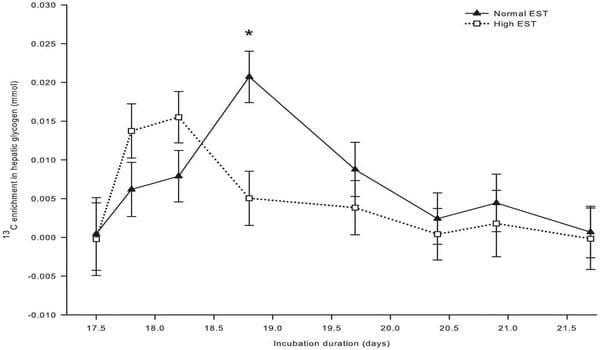
Figure 6. 13C in hepatic glycogen of embryos incubated at two different eggshell temperature (study III). 13C in hepatic glycogen after injecting a single bolus of [U-13C]glucose (1.0 mg in 250 µL sterile water) at day 17.5 of incubation in the chorio-allantoic fluid of embryos incubated at a normal (37.8ºC) or high (38.9ºC) eggshell temperature (EST) from day 10.5 of incubation onward. *Significant difference between EST treatments (P,0.05). At day 17.5, 17.8, 18.2, and 18.9 of incubation, no chickens were hatched yet. At day 19.7 of incubation, 22% of the chickens of the high EST treatment and 10% of the chickens of the normal EST treatment were hatched. At day 20.4 of incubation, 80% of the chickens of the high EST treatment were hatched and all chickens of the normal EST treatment were hatched. At day 20.9 and 21.6 of incubation, all chickens had been hatched. doi:10.1371/journal.pone.0059637.g006
Results
Study I and II: 13C Enrichment in Expired CO2
In study I, a single bolus of [U-13C]glucose was injected at day 14.5 of incubation and 13C enrichment in expired 13CO2 showed a peak for both EST treatments within 8 hours after the injection (Figure 1A). The increase in 13C enrichment after the single bolus of [U-13C]glucose was numerically 13% higher at the high EST treatment compared to the normal EST treatment. From day 14.8 of incubation onward, 13C enrichment in expired CO2 decreased gradually in both EST treatments but did not return to the baseline until day 17.6 of incubation.
In study II, a bolus of [U-13C]glucose was injected on 4 consecutive days from day 14.5 of incubation onward. 13C enrichment in expired CO2 responded to each bolus (Figure 2), but the height of the 13C enrichment peak increased with each of the four injections (respectively 0.0237, 0.0449, 0.0920 and 0.1465 APE). The time to reach this maximum 13C enrichment decreased with each of the four injections (from approximately 5.5 to 1.5 hours). At approximately 1 day after the 1st and 2nd injection, the increase in 13C enrichment in expired CO2 was numerically lower at the high than at the normal EST treatment. In contrast, after the 3rd and 4th injection, the 13C enrichment in expired CO2 was numerically higher at the high than at the normal EST treatment.
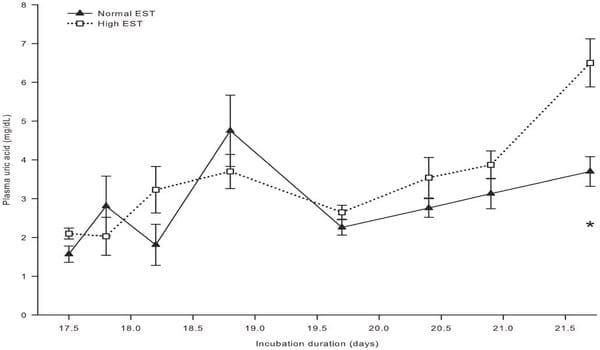
Figure 7. Plasma uric acid concentration of embryos incubated at two different eggshell temperatures (study III). Plasma uric acid concentration of embryos incubated at a normal (37.8ºC) or high (38.9ºC) eggshell temperature (EST) from day 10.5 of incubation onward. *Significant difference between EST treatments (P<0.05). At day 17.5, 17.8, 18.2, and 18.9 of incubation, no chickens were hatched yet. At day 19.7 of incubation, 22% of the chickens of the high EST treatment and 10% of the chickens of the normal EST treatment were hatched. At day 20.4 of incubation, 80% of the chickens of the high EST treatment were hatched and all chickens of the normal EST treatment were hatched. At day 20.9 and 21.6 of incubation, all chickens had been hatched. doi:10.1371/journal.pone.0059637.g007
Study III: 13C Enrichment in Expired CO2
For both EST treatments, the 13C enrichment in expired CO2 showed a biphasic pattern after a single injection of [U-13C]glucoseat day 17.5 of incubation (Figure 3). The first peak occurred within 3 hours after injection and this peak was approximately 36% higher in the high EST treatment than in the normal EST treatment (Δ= 0.02512; P<0.05). Eggs in the normal EST treatment were injected approximately 1.5 hours prior to eggs in the high EST treatment, resulting in a small time lag between the two treatments for the first peak in CO2 enrichment (at day 17.5 vs 17.6 of incubation, respectively). Nonetheless, the 2nd peak occurred 1.3 days after the injection at the normal EST treatment and already at 0.9 days after the injection at the high EST treatment. The height of the peak did not differ between EST treatments (P>0.05). 13C enrichment in expired CO2 decreased with time after the 2nd peak and returned to baseline at day 19.5 of incubation for the high EST treatment and at day 19.8 of incubation for the normal EST treatment (P>0.05).
The 13C enrichment in expired CO2 was higher at the high EST treatment than at the normal EST treatment from day 17.6 until 17.8 of incubation (P<0.05). Recovery of 13C in expired CO2 relative to the dose of 13C that was injected as glucose in the eggs tended to be higher (P = 0.07) at the high (46.7±0.96%) than at the normal (42.0±0.96%) EST treatment.
Study III: 13C Enrichment in Plasma Glucose and Lactate, and Hepatic Glycogen
13C enrichment in plasma glucose (Figure 4) showed a single peak at day 17.8 of incubation, and was not affected by EST (P = 0.35). 13C enrichment in plasma lactate was 82% higher at the high than at the normal EST treatment at day 17.8 of incubation (interaction EST x day of incubation; P<0.001; Figure 5). For both EST treatments, 13C enrichment in plasma lactate exceded the background enrichment until day 18.8 of incubation. 13C in hepatic glycogen was lower at the high EST than at the normal EST treatment at day 18.8 of incubation (interaction EST6day of incubation; P,0.05; Figure 6). 13C in hepatic glycogen was higher at day 18.8 than at day 17.5 of incubation in the normal EST treatment.
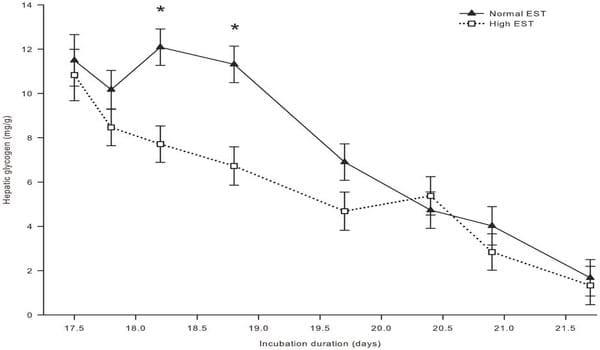
Figure 8. Hepatic glycogen concentration of embryos incubated at two different eggshell temperatures (study III). Hepatic glycogen concentration of embryos incubated at a normal (37.8ºC) or high (38.9ºC) eggshell temperature (EST) from day 10.5 of incubation onward. *Significant difference between EST treatments (P<0.05). At day 17.5, 17.8, 18.2, and 18.9 of incubation, no chickens were hatched yet. At day 19.7 of incubation, 22% of the chickens of the high EST treatment and 10% of the chickens of the normal EST treatment were hatched. At day 20.4 of incubation, 80% of the chickens of the high EST treatment were hatched and all chickens of the normal EST treatment were hatched. At day 20.9 and 21.6 of incubation, all chickens had been hatched. doi:10.1371/journal.pone.0059637.g008
Study III: 13C Enrichment in Plasma Glucose and Lactate, and Hepatic Glycogen
13C enrichment in plasma glucose (Figure 4) showed a single peak at day 17.8 of incubation, and was not affected by EST (P = 0.35). 13C enrichment in plasma lactate was 82% higher at the high than at the normal EST treatment at day 17.8 of incubation (interaction EST x day of incubation; P<0.001; Figure 5). For both EST treatments, 13C enrichment in plasma lactate exceeded the background enrichment until day 18.8 of incubation. 13C in hepatic glycogen was lower at the high EST than at the normal EST treatment at day 18.8 of incubation (interaction ESTxday of incubation; P<0.05; Figure 6). 13C in hepatic glycogen was higher at day 18.8 than at day 17.5 of incubation in the normal EST treatment.
Study III: Blood Metabolites and Liver Glycogen
Plasma glucose and lactate concentrations were not affected by EST (both P>0.50), but both increased with time of incubation (P<0.001). Plasma glucose concentrations increased from 172.1 mg/dL at day 17.5 to 223.1 mg/dL at day 21.6 of incubation. Plasma lactate concentrations increased from 18.4 mg/dL at day 17.5 to 45.9 mg/dL at day 20.9, and decreased thereafter to 35.7 mg/dL at day 21.6 of incubation (P<0.05; data not shown). Plasma uric acid concentrations were 43% higher for chickens incubated at a high than at a normal EST treatment at day 21.6 of incubation (Δ = 2.8 mg/mL; interaction EST x day of incubation; P<0.05; Figure 7). Hepatic glycogen concentrations were lower for chickens incubated at a high than at a normal EST treatment at day 18.2 of incubation (Δ= 4.37 mg/ g) and at day 18.8 of incubation (Δ = 4.59 mg/g; interaction EST x day of incubation; P<0.05; Figure 8).
Study III: Embryo and Chicken Characteristics
At day 17.5, 17.8, 18.2, and 18.9 of incubation, no chickens were hatched yet. At day 19.7 of incubation, 22% of the chickens of the high EST treatment and 10% of the chickens of the normal EST treatment had hatched. At day 20.4 of incubation, 80% of the chickens of the high EST treatment were hatched and all chickens of the normal EST treatment were hatched. At day 20.9 and 21.6 of incubation, all chickens had been hatched.
Eggshell temperature did not affect YFBM until day 20.4 of incubation. YFBM was lower at the high than at the normal EST treatment at day 20.9 (Δ = 2.74 g) and day 21.6 (Δ = 3.81 g) of incubation (interaction EST x day of incubation; P<0.001; Figure 9). Yolk weight decreased from 12.5 g to 4.2 g from day 17.5 to 21.6 of incubation (P<0.001), but this decrease was not affected by EST (P>0.10). Liver weight increased during incubation and was 0.112 g lower at the high EST than at the normal EST treatment at day 21.6 of incubation (interaction EST xday of incubation; P<0.05; data not shown).
Discussion
Pattern of Glucose Oxidation in Chicken Embryos
To our knowledge, this is the first study that describes the pattern of glucose oxidation in the chicken embryo, which is a unique model to study embryonic metabolism without maternal influences. Glucose oxidation in the chicken embryo was detected between 48 and 72 hours after injection of a single bolus of [U-13C]glucose in the chorio-allantoic fluid. This is three times longer than commonly found in other species in their postnatal life. [U-13C]glucose is normally completely oxidized within 20 hours after an oral bolus in human infants [33]and adults [34], mice [35], rats [36], milk-fed calves [37], pigs [38], and goats [39]. In male chickens, oxidation of dietary [U-13C]glucose was completed approximately 10 hours after oral administration [40]. The relatively low rate of glucose oxidation in chicken embryos may be explained by the stage of development. Chicken embryos may have a lower glucose entry rate into the systemic circulation because the glucose supply predominantly originates from gluconeogenesis [11,13,41], whereas the majority of the glucose supply in growing and adult animals originates from exogenous (i.e. dietary) sources. Whole body glucose turnover might be reduced in chicken embryos compared to animals in their postnatal life. The longer period of glucose oxidation in chicken embryos can also be related with the absorption rate or other characteristics of the extra-embryonic allantoic fluid.
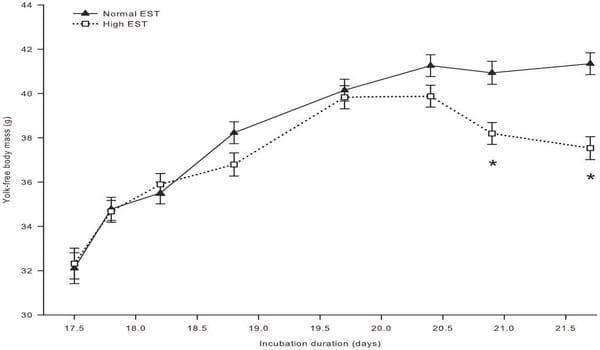
Figure 9. Embryo and chicken yolk-free body mass during incubation at two different eggshell temperatures (study III). Yolk-free body mass of embryos incubated at a normal (37.8ºC) or high (38.9ºC) eggshell temperature (EST) from day 10.5 of incubation onward. *Significant difference between EST treatments (P<0.05). At day 17.5, 17.8, 18.2, and 18.9 of incubation, no chickens were hatched yet. At day 19.7 of incubation, 22% of the chickens of the high EST treatment and 10% of the chickens of the normal EST treatment were hatched. At day 20.4 of incubation, 80% of the chickens of the high EST treatment were hatched and all chickens of the normal EST treatment were hatched. At day 20.9 and 21.6 of incubation, all chickens had been hatched. doi:10.1371/journal.pone.0059637.g009
Interestingly, glucose oxidation in the current study was characterized by a biphasic pattern when the bolus was injected at day 17.5 of incubation (study III) but not when the injection was applied at day 14.5 of incubation (study I). The second peak in glucose oxidation may be related with the uptake of glucose from the allantoic fluid prior to the hatching process. The allantoic fluid is gradually absorbed from day 13 until 20 of incubation [42]. A study in the Australorp chicken embryo showed that a large portion of the allantoic fluid was absorbed between day 18 and 19 of incubation with a concurrent increase in blood glucose concentration [43]. The increased glucose uptake from the allantoic fluid occurs simultaneously with the higher glucose requirement around day 19 of incubation when the hatching process starts [11,13]. The hatching process is energy-demanding and coincides with marginal oxygen availability because of the limited gas exchange of the eggshell conductance and the chorioallantoic membrane [11,14,44]. Therefore, active muscles require anaerobic glycolysis to meet the high physical requirements [11,12,18] and glucose is an important ATP-yielding substrate during the hatching process [13,27,28]. Glucose is mainly generated from glycogen stores [11,12,18] as shown by the decrease in hepatic glycogen in the current study as well, but glucose absorbed from the allantoic fluid may also contribute during the hatching process. Combining the [U-13C]glucose tracer with an appropriate tracer for glucose uptake from the allantoic fluid would allow further refinement of the tracer technique and provide better insight in the biphasic oxidation pattern in the avian embryo.
In addition, the chicken embryo may deaminate glucogenic amino acids during the hatching process which are either immediately oxidized for ATP production [45] or first converted to glucose by gluconeogenesis [46] to allow anaerobic glycolysis. The higher plasma uric acid concentrations in the normal EST treatment at day 18.8 compared to 17.5 of incubation may suggest that gluconeogenesis of glucogenic amino acids may increase around the start of the hatching process. This was less pronounced in the high EST treatment, but this may be related with the sampling time and the physiological status of the embryo or chicken.
Glucose Oxidation during Embryonic Development
In study II, the rate of glucose oxidation increased with each consecutive injection and the time to reach the maximum rate of oxidation decreased. Technically, this could be related to preferential oxidation of 13C labeled glucose, because repeated administration of the tracer may increase substrate-induced oxidation. However, the total amount of exogenous glucose was only 0.73 mg in each of the 4 boluses, which accounts for 0.18% of the average glucose pool (˜415 mg) in a 60 g egg between day 14 and 17 of incubation [43]. When such small amounts of glucose are administered, it is unlikely that the tracer administration has influenced embryonic glucose metabolism (in accordance with [47]). This suggests that the consistent increase in glucose oxidation reflects an increase in glucose requirements during embryonic development. Our findings correspond with the increase in glycolysis in muscle and liver during embryonic development [27]. Furthermore, gluconeogenesis and glycogenesis commonly increase during the second half of incubation [48,49,50,51], indicating that total glucose metabolism is upregulated towards hatching.
Glucose Oxidation at High Embryonic Temperatures
Our hypothesis was that the lower hepatic glycogen stores found in previous studies after high EST incubation [3,52] could be explained by higher glucose oxidation. Indeed, glucose oxidation indicated by the recovery of 13C from glucose tended to be 4.7% higher at the high than at the normal EST treatment, whereas 13C enrichment in hepatic glycogen was lower at the high than at the normal EST treatment at day 18.8 of incubation. These results suggest that embryos incubated at a high EST oxidize more glucose than embryos incubated at a normal EST.
Increased glucose oxidation at the high EST treatment probably explains the lower hepatic glycogen stores at day 18.2 and 18.8 of incubation in the high EST embryos. These findings correspond with previous studies in which embryos incubated at high temperatures had reduced amounts of hepatic glycogen just before the hatching process started [3,52]. When the amount of hepatic glycogen becomes too low, embryos may not survive the hatching process [7,8,24,53,54].
Glucose Oxidation and Body Development
The higher plasma uric acid concentrations in the high EST chickens at day 21.6 of incubation. indicate that glucogenic amino acids were deaminated. Our results are consistent with a previous study that showed that a high EST (38.9ºC vs 37.8ºC) was associated with higher plasma uric acid concentrations and a lower efficiency in protein utilization for growth in chicken embryos [55]. The effects of a high EST on glucose and protein metabolism may explain the decrease in YFBM that was found at day 20.8 and 21.6 of incubation in the current study and in many other studies [7,8,24,25,56,57].
Anaerobic Glucose Metabolism
We hypothesized that anaerobic glycolysis is higher at a high than at a normal EST treatment because of a higher metabolic rate. Although plasma lactate concentrations were similar for both EST treatments, 13C enrichment in plasma lactate was substantially higher (+82%) for the high compared to the normal EST treatment directly after the [U-13C]glucose injection, i.e. at day 17.8 of incubation. This indicates an increased flux of 13C labeled glucose through anaerobic glycolysis for embryos incubated at a high compared to normal EST, and confirms our hypothesis. The absence of a difference in plasma lactate concentrations may be explained by an increased clearance rate of lactate that compensates for a higher production rate (for detailed discussion: [58]). Similarly, a study of [59] reported that lactate recycling increased at high temperatures during the early hatching phase in turkey embryos.
In conclusion, the current study showed that glucose oxidation is relatively slow in chicken embryos but increases consistently with the developmental stage of the embryo. Glucose oxidation in chicken embryos shows a biphasic pattern in the perinatal period. A high environmental temperature in the perinatal period increases the glucose requirement in chicken embryos, as indicated by an increase in glucose oxidation and a decrease in hepatic glycogen, Because of the limited glucose availability in the avian egg, the increased requirement may jeopardize successful hatching and impact body development, possibly mediated by increased gluconeogenesis from glucogenic amino acids to allow anaerobic glycolysis.
Acknowledgments
The authors thank all students and colleagues of Wageningen University (Wageningen, the Netherlands) who assisted with this experiment. The authors thank hatchery Lagerwey B.V. (Lunteren, the Netherlands) for donating eggs and for their assistance.
Author Contributions
Conceived and designed the experiments: R. Molenaar JvdB EH HvdB. Performed the experiments: R. Molenaar EH. Analyzed the data: R. Molenaar. Contributed reagents/materials/analysis tools: MH NBK. Wrote the paper: R. Molenaar JvdB R. Meijerhof BK HvdB.
This article was originally published in PLoS ONE 8(4): e59637. doi:10.1371/journal.pone.0059637. This is an Open Access article distributed under the terms of the Creative Commons Attribution License.
References
1. Van der Lende T, Knol EF, Leenhouwers JL (2001) Prenatal development as a predisposing factor for perinatal losses in pigs. Reprod Suppl 58: 247–261.
2. Taylor PD, Poston L (2007) Developmental programming of obesity in mammals. Exp Physiol 92: 287–298.
3. Molenaar R, Van den Anker I, Meijerhof R, Kemp B, Van den Brand H (2011a) Effect of eggshell temperature and oxygen concentration during incubation on the developmental and physiological status of broiler hatchlings in the perinatal period. Poult Sci 90: 1257–1266.
4. Molenaar R, Hulet R, Meijerhof R, Maatjens CM, Kemp B, et al. (2011b) High eggshell temperatures during incubation decrease growth performance and increase the incidence of ascites in broiler chickens. Poult Sci 90: 624–632.
5. Christensen VL, Wineland MJ, Fasenko GM, Donaldson WE (2011) Egg storage effects on plasma glucose and supply and demand tissue glycogen concentrations of broiler embryos. Poult Sci 80: 1729–1735.
6. Mota-Rojas D, Orozco-Gregorio H, Villaneuva-Garcia D, Bonilla-Jaime H, Suarez-Bonilla X, et al. (2011) Foetal and neonatal energy metabolism in pigs and humans: a review. Veterinarni Medicina 56: 215–225.
7. Wilemsen H, Kamers B, Dahlke F, Han H, Song Z, et al. (2010) High- and lowtemperature manipulation during late incubation: Effects on embryonic development, the hatching process, and metabolism in broilers. Poult Sci 89: 2678–2690.
8. Willemsen H, Li Y, Willems E, Franssens L, Wang Y, et al. (2011) Intermittent thermal manipulations on broiler embryos during late incubation and their immediate effect on the embryonic development and hatching process. Poult Sci 90: 1302–1312.
9. Romanoff AL, Romanoff AJ (1949) The avian egg. New York, NY, USA: John Wiley and Sons.
10. Kuc?era P, Raddatz E, Baroffio A (1984) Oxygen and glucose uptakes in the early chick embryo. In: Dordrecht, The Netherlands: Junk W, Seymour RS, editors. Respiration and metabolism of embryonic vertebrates. 299–309.
11. Moran Jr ET (2007) Nutrition of the developing embryo and hatchling. Poult Sci 86: 1043–1049.
12. Freeman BM (1965) The importance of glycogen at the termination of the embryonic existence of Gallus domesticus. Comp Biochem Physiol 14: 217–222.
13. De Oliveira JE, Uni Z, Ferket PR (2008) Important metabolic pathways in poultry embryos prior to hatch. World’s Poult Sci J 64: 488–499.
14. Tazawa H, Visschedijk AHJ, Wittmann J, Piiper J (1983) Gas exchange, blood gases and acid-base status in the chick before, during and after hatching. Respir Physiol 53: 173–185.
15. Rahn H, Ar A, Paganelli CV (1979) How bird eggs breathe. Sci Am 240: 46–55.
16. John TM, George JC, Moran Jr ET (1987) Pre- and post-hatch ultrastructural and metabolic changes in the hatching muscle of turkey embryos from antibiotic and glucose treated eggs. Cytobios 49: 197–210.
17. John TM, George JC, Moran Jr ET (1988) Metabolic changes in pectoral muscle and liver of turkey embryos in relation to hatching: Influence of glucose and antibiotic-treatment of eggs. Poult Sci 67: 463–469.
18. Freeman BM (1969) The mobilization of hepatic glycogen in Gallus domesticus at the end of incubation. Comp Biochem Physiol 28: 1169–1176.
19. Garci´a FJ, Pons A, Alemany M, Palou A (1986) Tissue glycogen and lactate handling by the developing domestic fowl. Comp Biochem Physiol 85B: 727– 731.
20. Foye OT, Ferket PR, Uni Z (2007) Ontogeny of energy and carbohydrate utilisation of the precocial avian embryo and hatchling. Avian Poult Biol Rev 18: 93–101.
21. Beattie J (1964) The glycogen content of skeletal muscle, liver and heart in late chick embryos. Br Poult Sci 5: 285–293.
22. Yadgary L, Uni Z (2012) Yolk sac carbohydrate levels and gene expression of key gluconeogenic and glycogenic enzymes during chick embryonic development. Poult Sci 91: 444–453.
23. Lourens A, Molenaar R, Van den Brand H, Heetkamp MJW, Meijerhof R, et al. (2006) Effect of egg size on heat production and the transition of energy from egg to hatchling. Poult Sci 85: 770–776.
24. Lourens A, Van den Brand H, Meijerhof R, Kemp B (2005) Effect of eggshell temperature during incubation on embryo development, hatchability, and posthatch development. Poult Sci 84: 914–920.
25. Lourens A, Van den Brand H, Heetkamp MJW, Meijerhof R, Kemp B (2007) Effects of eggshell temperature and oxygen concentration on embryo growth and metabolism during incubation. Poult Sci 86: 2194–2199.
26. Alferink SJJ, Van den Borne JJGC, Gerrits WJJ, Lammers-Wienhoven SCW, Heetkamp MJW (2003) On-line, continuous determination of 13CO2/12CO2 ratios by non-dispersive infrared absorption in indirect calorimatry facilities. In: Wageningen, The Netherlands, Progress in research on energy and protein metabolism: Wageningen Academic Publishers. 465–468.
27. Pearce J (1971) Carbohydrate metabolism in the domestic fowl. Proc Nutr Soc 30: 254–259.
28. Wittmann J, Weiss A (1981) Studies on the metabolism of glycogen and adenine nucleotides in embryonic chick liver at the end of incubation. Comp Biochem Physiol 69C: 1–6.
29. Wolanski NJ, Renema RA, Robinson FE, Carney VL, Fancher BI (2004) Yolk utilization and chick length as parameters for embryo development. Avian Poult Biol Rev 15: 233–234.
30. Vonk RJ, Hagedoorn RE, De Graaff R, Elzinga H, Tabak S, et al. (2000) Digestion of so-called resistant starch sources in the human small intestine. Am J Clin Nutr 72: 432–438.
31. Tetens V, Kristensen NB, Calder AG (1995) Measurement of 13C enrichment of plasma lactate by gas chromatography/isotope ratio mass spectrometry. Anal Chem 67: 858–862.
32. SAS Institute Inc (2004) SAS/STAT User’s Guide. Version 9.1. SAS Inst. Inc., Cary, NC.
33. Christian MT, Amarri S, Franchini F, Preston T, Morrison DJ, et al. (2002) Modeling 13C breath curves to determine site and extent of starch digestion and fermentation in infants. J Pediatr Gastroenterol 34: 158–164.
34. Robertson MD, Livesey G, Mathers JC (2002) Quantitative kinetics of glucose appearance and disposal following a 13C-labelled starch-rich meal: Comparison of male and female subjects. Br J Nutr 87: 569–577.
35. Ishihara K, Oyaizu S, Mizunoya W, Fukuchi Y, Yasumoto K, et al. 2002. Use of 13C-labeled glucose for measuring exogenous glucose oxidation in mice. Biosci Biotechnol Biochem 66: 426–429.
36. Luengo C, Azzout-Marniche D, Fromentin C, Piedcoq J, Lemosquet S (2009) [13C] GC-C-IRMS analysis of methylboronic acid derivatives of glucose from liver glycogen after the ingestion of [13C] labeled tracers in rats. J Chromatogr 877B: 3638–3644.
37. Van den Borne JJGC, Lobley GE, Verstegen MWA, Muijlaert J, Alferink SJJ, et al. (2007) Body fat deposition does not originate from carbohydrates in milk-fed calves. J Nutr 137: 2234–2241.
38. Bruininx E, Van den Borne J, Van Heugten E, Van Milgen J, Verstegen M, et al. (2011) Oxidation of dietary stearic, oleic and linoleic acids in growing pigs follows a biphasic pattern. J Nutr 141: 1657–1663.
39. Junghans P, Derno M, Gehre M, Ho¨fling R, Kowski P, et al. (1997) Calorimetric validation of 13C bicarbonate and double labeled water method for determining the energy expenditure in goats. Z Ernahrungswiss 36: 268–272.
40. Swennen Q, Verhulst P-J, Collin A, Bordas A, Verbeke K, et al. (2007) Further investigations on the role of diet-induced thermogenesis in the regulation of feed intake in chickens: Comparison of adult cockerels of lines selected for high or low residual feed intake. Poult Sci 86: 1960–1971.
41. Delphia JM, Elliot J (1965) The effect of high temperature incubation upon the myocardial glycogen in the chick embryo. J Embryol Exp Morph 14: 273–280.
42. Baggott GK (2001) Development of extra-embryonic membranes and fluid compartments. In: Lincolnshire, UK. Deeming DC, editors Perspectives in fertilisation and embryonic development in Poultry. Ratite Conference Books. 23–29.
43. Romanoff AL (1967) Biochemistry of the avian embryo: A quantitative analysis of prenatal development. Wiley-Interscience, New York, NY, USA.
44. Whittow GC, Tazawa H (1991) The early development of thermoregulation in birds. Physiol Zool 64: 1371–1390.
45. McArdle WD, Katch FI, Katch VL (1981) Exercise physiology: Energy, nutrition, and human performance. Lea and Febiger, Philadelphia, USA.
46. Hazelwood RL, Lorenz FW (1959) Effects of fasting and insulin on carbohydrate metabolism of the domestic fowl. Am J Physiol 197: 47–51.
47. Sunny NE, Bequette BJ (2010) Gluconeogenesis differs in developing chick embryos derived from small compared with typical size broiler breeder eggs. J Anim Sci 88: 912–921.
48. Kilsheimer GS, Weber DR, Ashmore J (1960) Hepatic glucose production in developing chicken embryo. Proc Soc Exp Biol Med 104: 515–518.
49. Rinaudo MT (1962) Glycogenesis in the liver of chicken embryo. Enzymologia 24: 230–236.
50. Ballard FJ, Oliver IT (1963) Glycogen metabolism in embryonic chick and neonatal rat liver. Biochim Biophys Acta 71: 578–588.
51. Nelson P, Yarnell G, Wagle SR (1966) Biochemical studies of the developing embryo II. Studies on CO2 fixation enzymes. Archs Biochem Biophys 114: 543– 546.
52. Clawson RC (1975) Effect of temperature alteration on liver and cardiac glycogen in the chick embryo. Comp Biochem Physiol 51A: 769–776
53. Christensen VL, Donaldson WE, Nestor KE (1993) Embryonic viability and metabolism in turkey lines selected for egg production or growth. Poult Sci 72: 829–838.
54. Yildirim I, Yetisir R (2004) Effects of different hatcher temperatures on hatching traits of broiler embryos during the last five days of incubation. S Afr J Anim Sci 34: 211–216.
55. Molenaar R, Meijerhof R, Van den Anker I, Heetkamp MJW, Van den Borne JJGC, et al. (2010) Effect of eggshell temperature and oxygen concentration on survival rate and nutrient utilization in chicken embryos. Poult Sci 89: 2010– 2021.
56. Romanoff AL (1936) Effects of different temperatures in the incubator on the prenatal and postnatal development of the chick. Poult Sci 15: 311–315.
57. Leksrisompong N, Romero-Sanchez H, Plumstead PW, Brannan KE, Brake J (2007) Broiler incubation. 1. Effect of elevated temperature during late incubation on body weight and organs of chicks. Poult Sci 86: 2685–2691.
58. Pe´ronnet F (2010) Lactate as an end-product and fuel. Dtsch Z Sportmed 61: 112–116.
59. Christensen VL, Wineland MJ, Grimes JL, Oviedo EO, Mozdziak PS, et al. (2007) Effect of incubator temperature and oxygen concentration at the plateau stage in oxygen consumption on turkey embryo muscle growth and development. Int J Poult Sci 6: 406–412.