INTRODUCTION
Amino acids (AA) and crude protein (CP) intake during the rearing phase in broiler breeders have demonstrated to affect body composition and reproductive performance in the laying period (Joseph et al., 2000; Ciacciariello and Tyler, 2013; van Emous et al., 2013, 2015a, 2015b). Modifications of these nutrients added up to the adjustment of feed allocation may alter BW (van Emous et al., 2013; Soumeh et al., 2018), organ development (Lesuisse et al., 2017), egg production (Hocking et al., 2002), egg traits, hatchability, and fertility (Walsh and Brake, 1999; Shafey, 2002) from the onset of production onward. Levels of 16 and 18% CP in the developer phase did not influence BW but increased egg size and production rate (Joseph et al., 2000). Some reports have suggested that grower and prebreeder diets with CP levels lower than 13% may increase abdominal fat deposition, reduce breast weight, and enhance incubation from 22 to 45 wk of age (van Emous et al., 2013, 2015b). Soumeh et al. (2018) described a linear increase in breast weight when CP levels during rearing ranged from -20% to +5% of the standard Ross 308 (Aviagen, 2016). However, the abdominal fat deposition decreased linearly with dietary CP concentration without affecting egg production per hen housed (HHEP) nor fertility percentage (Soumeh et al., 2018). In contrast, Lesuisse et al. (2017) reported that reducing CP concentrations by 25% (starter 14.30, grower 11.76, and prebreeder 12.65% CP) during the rearing phase decreased egg production, fertility, and the egg traits up to 39 wk of age.
Despite existing nutritional recommendations for broiler breeders (Aviagen, 2016; Rostagno et al., 2017; Cobb-Vantress, 2018, 2020), very few published studies have described the effects of AA levels close to these recommendations for pullets and their impact after the onset of egg production to estimate optimums. One of the key factors during rearing to obtain the best reproductive performance is the target BW at photostimulation age (Decuypere et al., 2010; van der Klein et al., 2018a, b; Salas et al., 2019), which is associated with an adequate ovarian function (Hocking, 1993, 1996; Hocking et al., 2002). An excess of Lys intake may produce greater muscle depositions leading to an increment of BW and, consequently, a low reproductive performance (Lopez and Leeson, 1995; Mejia et al., 2012b). Moreover, Da Silva et al. (2015) observed that egg production decreased linearly between 24 and 40 wk of age when Met+Cys in rearing diets changed from 0.688 to 0.988%, estimating a minimum requirement of 0.688% of these AA for Cobb 500 pullets. The same study demonstrated that pullets fed diets with 0.92% of Met+Cys resulted in lower hatchability at 40 wk.
Several studies have evaluated the effect of CP and AA levels for broiler breeders during the laying phase (Lopez and Lesson, 1995; Enting et al., 2007; Mejia et al., 2012a, b; Ekmay et al., 2013; Butler, 2019; Cerrate et al., 2019). However, estimations of optimum requirements for these nutrients during the rearing period and their impact in the laying phase are scarce. Hence, the objective of this study was to evaluate the effects of 4 AA levels during rearing on egg quality and production, fertility, hatchability, and embryo mortality from 24 to 65 wk of age on Cobb 500 slow-feathering (SF) hens. To estimate the best AA levels during rearing, diets fluctuating from 20% below to 10% above the Cobb 500 recommendations (Cobb-Vantress, 2018) were evaluated in the grower and developer phases. We hypothesized that AA levels would affect BW and reproductive performance.
MATERIALS AND METHODS
The experiment was conducted with the approval of animal use by the Animal Care and Use Committee and in compliance with the Guidelines for Care and Use of Laboratory Animals at North Carolina State University. Specific details of treatments, husbandry, and data collection during the rearing phase were presented by Alfaro-Wisaquillo et al. (2021).
Experimental Treatments
All females were fed one common starter diet (Table 1) in crumbles from placement to 28 d of age to obtain pullets of similar BW before starting the experimental period. After 4 wk, 4 AA levels were evaluated during the grower and developer phases. These levels were 80% (low-AA), 90% (moderate-AA), 100% (standard-AA), and 110% (high-AA) of the recommendations for Cobb 500 SF pullets (Cobb-Vantress, 2018). Amino acids were guided by an ideal protein profile based on digestible (dig) Lys, according to the Cobb-Vantress (2018) parent stock guidelines. Grower and developer diets were formulated to meet or slightly exceed AA recommendations according to the desired level and under practical feed formulation (Table 1). Pullets were fed with grower diets until 105 d of age with 0.48, 0.54, 0.60, and 0.66% of dig Lys and developer diets from 106 d up to onset of egg production that occurred at 24 wk of age with 0.51, 0.57, 0.63, and 0.69% dig Lys. All diets were offered in the coarse mash with approximately 1,000 μm for the grower and 1,300 μm geometric mean particle size for developer diets. Diets contained 2,700 kcal/kg of AMEn and 2,800 kcal/kg of AMEn for the grower and developer phases, respectively. Corn, soybean meal, and wheat midds were analyzed prior to feed formulation of experimental diets by near-infrared spectroscopy (NIRS, DS2500, FOSS, Hillerod, Denmark). For nutrient content, all diets were analyzed according to the AOAC (2006) methods reported in Alfaro-Wisaquillo et al. (2021). The composition of CP and AA were closed to formulated values (Table 1).
Husbandry of Broiler Breeders
Pullets A total of 1,360 Cobb-500 slow-feathering broiler breeder pullets and 288 Cobb MV male chicks were obtained from a commercial hatchery (North Carolina Cobb Hatchery, Wadesboro, NC). In a solid-sided rearing house with negative pressure ventilation, these birds were randomly distributed in 16 pullet floor pens (85 pullets/pen) within 4 treatments with 4 replicates each and a density of 4.6 pullets/m2. Cockerels were placed in 16-floor pens (18 cockerels/pen) with a density of 3.4 cockerels/m2. No treatments were assigned to males.
Feeding Program The feeding program from 1 to 2 wk was ad libitum, and after 3 wk, it was restricted. A 6/1 (skip-a-day) program (fed Monday through Saturday only) was established from 4 to 5 wk. Finally, from 6 wk onward until the end of the trial, a 5/2 (skip-two-days) program was fixed (fed on Monday, Tuesday, Thursday, Friday, and Saturday). On the developer phase, feed amounts varied slightly (±3 g/bird/day) among treatments to maintain BW target close (±2%) regarding Cobb-Vantress (2018) guidelines (Alfaro-Wisaquillo et al., 2021). After 25 wk, all hens were fed with one standard layer diet in 2 phases, layer 1, from the first egg to 40 wk, and layer 2, from 41 to 65 wk with a similar amount of feed offered (Figure 1). Feed increments were made according to egg production by treatment, and feed offered was decreased after the peak of egg production between 0.5 and 1g (Figure 1). In the whole experiment, all males were not subjected to treatments.
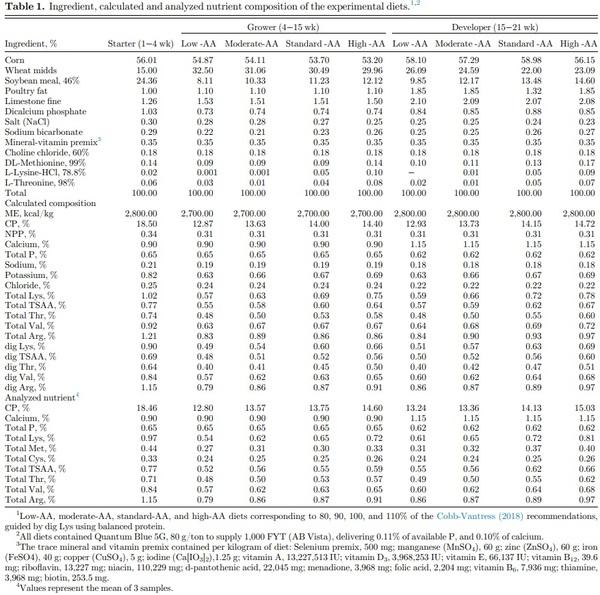
Cockerels were fed the standard treatment grower and developer diets during rearing and male diets during the laying phase (Table 2), following Cobb-Vantress (2018) recommendations.
Lighting and Vaccination Program The day length was 14 h at 22 wk, 14.5 h by 5% of lay approximately at 25 wk, and 15 h by 50% of production at 28 wk. Light hours were provided from 5:30 am to 8:30 pm each day in the laying phase. Vaccinations were applied only in the rearing phase for pullets and cockerels and were reported on Alfaro-Wisaquillo et al. (2021).
Laying Phase At 20 and 21 wk of age, all birds were individually weighed to select pullets and cockerels to be transferred to the laying house. Only pullets and cockerels representing the average BW per pen plus or minus 3 standard deviations were used. Pullets were fed with the same dietary treatment after the transfer, and cockerels were randomized to be assigned on each pen. Consequently, 1,040 females and 112 males were placed into 16-floor pens with slats (17.35 m2 total area/pen) in a curtain-sided breeder house with a distribution of 65 hens and 7 roosters per pen to keep a female:male ratio of 9.28:1. During laying, each breeder pen was equipped with 4 tube female feeders, one tube male feeder, and special grills were installed around them to guarantee separate sex feeding. Access to water was limited to 8 h per day using 2 automatic bell-type drinkers per pen to avoid overdrinking and negative effects on droppings and litter moisture. Male and female mortality was verified, weighed, and recorded twice a day.
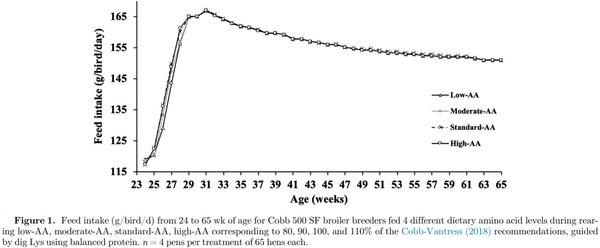
Data Collection
Results of feed and nutrient intake, growth, fleshing, and bone development during the rearing phase were presented in Alfaro-Wisaquillo et al. (2021) and only will be used for discussion.
Body Weight, Flock Uniformity, and Body Composition At 25 wk of age, 3 breeders per pen, 12 breeders per treatment were randomly selected and sacrificed by cervical dislocation after fasting of 36 h to obtain breast score and weight of the breast, fat deposits, liver, and ovary. The relative weight (RW) for each section was calculated and was expressed as a percentage of live empty BW after 24 h feed withdrawal. Additionally, the whole intestine, ceca, and oviductal parts were measured, and relative length (RL) was calculated. Furthermore, individual BW of all broiler breeders was collected before feeding time and recorded to calculate BW gain and uniformity (CV% of individual BW/pen) at 25, 32, 36, 40, 46, 50, 56, 60, and 65 wk of age. Feed intake (FI) (g/bird/d) was calculated to keep target BW, and egg production from 24 to 65 wk.
Reproductive Performance Egg production, fertility, and hatchability were determined up to 65 wk. Eggs were collected 3 times daily and stored in an egg cooler at 18°C and 50% relative humidity for 4 to 6 d before incubation. Although, the eggs laid outside the nests were collected separately, recorded, and no incubated. The percentage of egg production (EP) and total HHEP were calculated weekly. For incubation parameters, a total of 15 incubations with 2,880 eggs each (180 eggs/breeder pen) were carried out every 2 or 3 wk. Eggs were set on trays identified with pen number and placed into a single-stage Jamesway J-12 incubator (Butler Manufacturing Co., Ft. Atkinson, WI). Standard conditions of incubation (37.78 °C eggshell temperature) were established not to exceed 38.3°C (100.9°F) of eggshell temperature at the last incubation period with approximately 50% relative humidity and turning angle each hour. At 18 d of incubation, eggs were transferred to hatching baskets preserving the tray number from the incubator, and were placed into a Jamesway J-12 hatcher. At hatch, unhatched eggs were broken out to evaluate the cause of embryo mortality through standard embryo diagnosis techniques. The embryo stages were classified and recorded as follows: infertile, early dead 24 h and 48 h, blood ring, black eye, presence of feathers, malposition, internal and external pipping, eggs contaminated, and cracked shells. Later these categories were grouped in early (1−7 d), middle (8−17 d), and late (18−21 d) embryo mortality. Fertility was calculated as 100, minus the percentage of eggs classified as infertile in the embryo diagnosis. Hatchability was calculated as the number of chickens hatched divided into hatching eggs set times 100.
Egg Composition and Quality At 30, 36, 42, 48, 53, 58, and 63 wk of age, egg weight, egg uniformity (CV%), and quality parameters were determined on 30 eggs per each breeder pen. In each evaluation, the eggs were collected in 1 d, specifically in the morning. Dirty, cracked, or double-yolked eggs were rejected for these analyses. The albumen height and Haugh units (HU) were measured using an electronic tripod micrometer and recorded with the TSS QCD system (Technical Services and Supplies, Dunnington, York, UK). Albumen was rinsed from the shell with water, and the shell with membrane intact was air-dried. Afterward, the weight of wet albumen was calculated by subtracting the yolk weight and the air-dried shell weight from the total egg weight. Egg components (shell, yolk, and albumen) were expressed as a percentage of initial egg weight, and the ratio for yolk:albumen ratio (Y:A) was calculated. Shell strength (N), and elasticity (mm) were evaluated using the TA-XT Plus Texture Analyzer (Model TA-XTplus, Texture Technologies, Scarsdale, NY 2016). Shell thickness (mm) was measured at the equator with an electronic caliper (iGAGING Absolute Origin SpeedMic Micrometer, San Clemente, CA).
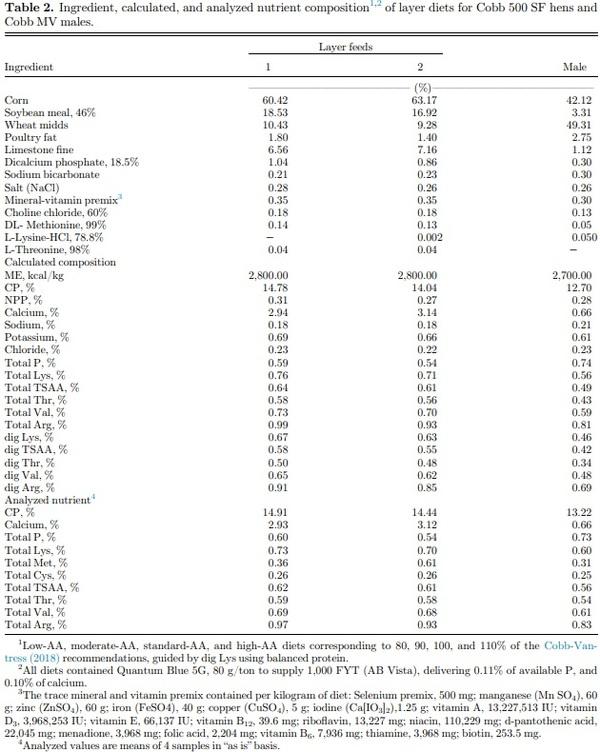
Statistical Analysis
All data were analyzed with statistical software JMP PRO 14 (SAS Institute, Cary, NC) in a completely randomized design with 4 levels of AA. Each treatment had 4 replicate pens with 65 hens and 7 cockerels each. One-way ANOVA was used to detect the effects of levels of AA and Tukey’s HSD test for mean comparisons. Quadratic and broken line (Robbins et al., 2006) regression analyses were used to estimate the optimum level of AA. Response surface analysis was used to identify the effects of AA levels during rearing as breeders aged on egg weight and composition, fertility, hatchability, and embryo mortality. Pairwise correlations were conducted to determine important factors among breast score, BW, the relative weight of breast, fat, liver, ovary, and relative length of the whole intestine, ceca, and oviductal parts. Percentage data for hens and embryo mortality were transformed using the arcsine square root percentage for analysis. Statistical significance was established to be P < 0.05.
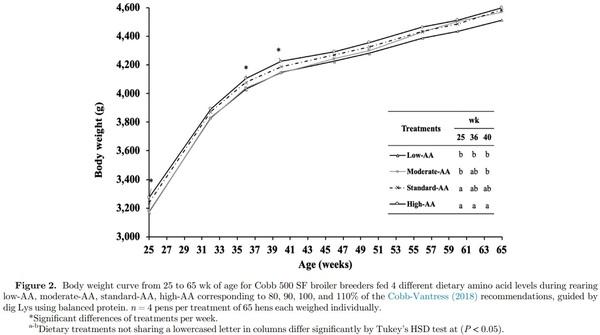
RESULTS
Growth and Body Composition
Results related to BW and body composition up to 22 wk of age were evaluated during growing and presented by Alfaro-Wisaquillo et al. (2021). Significant effects (P < 0.05) of AA levels were detected on BW at 25, 36, and 40 wk of age (Figure 2). At 25 wk, heavier breeders were observed when pullets were fed diets containing either 100 or 110% AA during rearing compared to breeders fed diets with 80 or 90% of AA content. At 36 wk, the mean difference indicated that breeders fed diets during grower and developer phases, with 80% AA were lighter compared to breeders from the treatment with the highest AA level. Rearing diets with high AA concentration resulted in heavier hens at 40 wk, than those fed diets with low and moderate AA levels. Additionally, BW increased linearly as AA levels augmented in rearing diets at 25 wk (BW, g = 2,863.174 + 3.713 * AA%; P < 0.001; R2 = 0.56), 36 wk (BW, g = 3,818.564 + 2.569 * AA%; P = 0.008; R2 = 0.40), and 40 wk of age (BW, g = 3,922.844 + 2.665 * AA%; P = 0.005; R2 = 0.43). No significant differences in BW, nor linear or quadratic effects of AA levels were detected (P > 0.05) at 32 wk and from 46 wk to the end of the experiment. Flock uniformity (CV%) was not affected (P > 0.05) by dietary treatments along with the laying phase.
At 25 wk, breeders fed diets with less AA content (low-AA and moderate-AA) had 0.4 g more abdominal fat (P = 0.034) than those fed standard-AA and highAA diets (1.25 vs. 0.86% of BW). Moreover, there were (P < 0.001) negative correlations (r = -0.63) between BW and RL of small intestine and between BW and RL ceca (r = -0.42). No other significant correlations were found (P > 0.05) on the relative weight of the breast, fat deposits, liver, and ovary, nor the relative length of oviductal parts. During the laying phase, total hen mortality was 12.02% for low-AA, 9.98% for moderate-AA, 13.31% for standard-AA, and 9.61% for high-AA level, and was not affected (P > 0.05) by dietary treatments during rearing. However, higher mortality (P < 0.05) was observed between 55 and 60 wk on hens fed standard-AA diets (1.70%) compared to breeders in the lowAA (0%) and moderate-AA (0.42%) treatments; highAA levels resulted in an intermediate response (1.65%).
Reproductive Performance
Egg Production Breeders came into production at 165 d of age, and 5% of production was reached at 167 d of age. AA levels evaluated did not affect (P > 0.05) the onset of EP. The EP (Figure 3) and HHEP were calculated from the first egg to 65 wk of age. Breeders fed diets during rearing with moderate-AA or high-AA reached the peak production at 30 wk with 85.92 and 86.72%, respectively, while the highest weekly EP for hens fed with low-AA was observed at 31 wk with 81.37%. Diets with standard-AA resulted in the last treatment to reach the maximum EP at 32 wk with 85.61%. Significant differences (P < 0.05) due to treatments were observed on EP at 25, 26, 30, 39, and from 46 to 65 wk of age, except at 53 and 63 wk (Figure 3). Overall, the standard-AA diet was the treatment showing the best weekly EP consistently during the whole experiment. The EP increased linearly as AA levels augmented in the rearing diets at 25 (EP, % = -8.216 + 0.314 * AA%; P = 0.003; R2 = 0.50), 26 (EP, % = 16.425 + 0.376 * AA%; P = 0.015; R2 = 0.38), 29 (EP, % = 67.640 + 0.166 *AA%; P = 0.028; R2 = 0.32), and 30 wk (EP, % = 67.009 + 0.182 * AA%; P = 0.015; R2 = 0.38). The best dietary AA concentration during rearing was estimated to be at 96% by constant quadratic effects (P < 0.05) from 34 up to 65 wk of age. No differences among means (P > 0.05) on EP were detected at 24 wk, and from 27 to 45 wk of age, except at 30 and 39 wk.
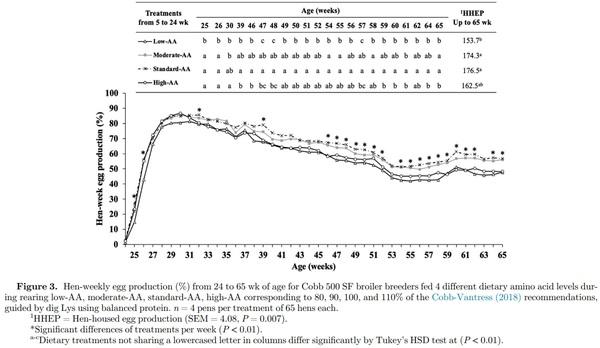
Significant effects (P = 0.007) of rearing diets were detected on total (65 wk) HHEP. Broiler breeders fed diets during rearing with 90 and 100% AA had the greatest average HHEP at 65 wk with 174.3 and 176.5 eggs, respectively. In contrast, breeders fed low-AA laid 153.7, and high-AA resulted in 162.5 cumulative eggs at the end of this trial (Figure 4). Quadratic effects (P = 0.002) of treatments were detected in HHEP at 65 wk (HHEP = -627.946 + 16.670 * AA% - 0.086 * AA%2 ; R2 = 0.65) with an optimum AA level of 96.7% during rearing. Moreover, the simple broken line analysis estimated that 89.7% AA was an optimum level for HHEP (RMSE = 9.28). However, a better fit of data (RMSE = 7.85) was observed in the quadratic broken line regression, demonstrating a maximum level for HHEP at 65 wk of 94.7% AA during rearing (Figure 4).
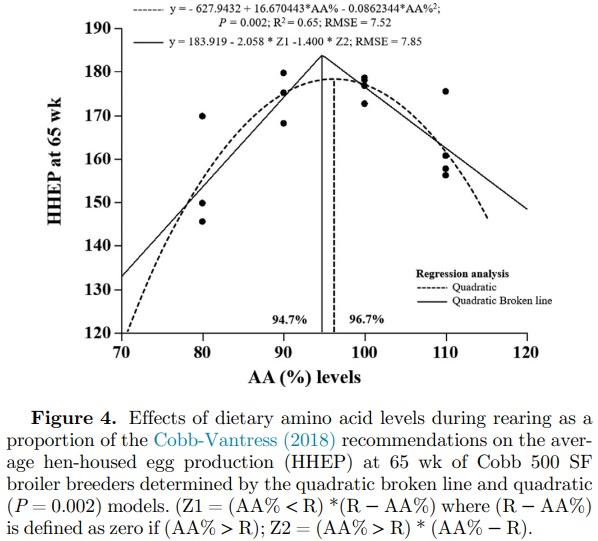
Egg Composition and Egg Properties Significant differences (P < 0.05) on egg weight due to AA levels were identified on 26, 28, 30, 37, 46, and 51 wk (Figure 5). At 26 wk, standard-AA and high-AA demonstrated to produce the heaviest eggs (P < 0.001), compared to low-AA levels that produced the lightest. In the same way, at 28 wk, only high-AA diets during rearing resulted (P = 0.009) in heavier eggs compared to low-AA diets. Simultaneously, linear effects (P < 0.01) indicated that egg weight increased as AA dietary concentration rise in the rearing diets at 26 (Egg weight, g = 45.099 + 0.076 * AA%; R2 = 0.74), and 28 wk of age (Egg weight, g = 52.346 + 0.042 * AA%; R2 = 0.47). At 37 wk, eggs from 110% AA diets were 1.56 g heavier (P = 0.002), on average, than eggs from the other treatments (66.75 vs. 65.19 g). Furthermore, the worst AA level for egg weight was identified at 89% AA according to the quadratic effect (Egg weight, g = 96.982−0.717 * AA% + 0.004 * AA%2; P = 0.036; R2 = 0.52). At 46 wk, mean difference (P < 0.001) demonstrated that egg weight from dietary treatments with 90%AA was 2.3 g higher that weight from other treatments (72.40 vs. 70.11 g). At 51 wk, 110%AA, and 100%AA showed the heaviest and the lightest eggs (P = 0.039), respectively, and a quadratic (P = 0.028) effect (Egg weight, g = 107.884−0.803 * AA% + 0.004 * AA%2; R2 = 0.39) determined the worst AA level for egg weight at 92%. At 58 wk, regression analysis indicated (P = 0.012) that egg weight increased with the concentration of AA in the pullet diets (Egg weight, g = 67.835 + 0.047 * AA%; R2 = 0.39). In general, the lightest egg weight (P = 0.022) during the whole cycle resulted from 89% of AA level on rearing diets, while the maximum egg weight (P < 0.001) was reached at 54 wk of age according to the response surface analysis (Egg weight, g = 21.660−0.370 * AA% + 0.002 * AA%2 + 2.456 *Age − 0.022 * Age2; R2 = 0.95), and egg uniformity was not altered (P > 0.05) due to treatments.
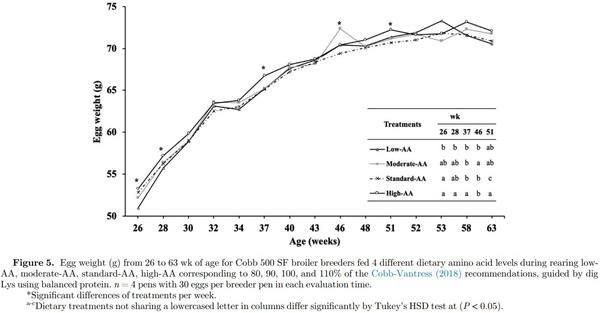
Levels of 80 and 90% AA improved (P = 0.038) the shell strength as compared to diets with 110% AA at 30 wk (42.6 vs. 37.9 N). In this study, high-AA content resulted in a better (P = 0.038) eggshell elasticity (0.23 mm) than moderate-AA (0.21 mm) at 36 wk. A similar effect (P = 0.040) was detected at 58 wk, low-AA, and high-AA content resulted in more elastic eggshells (0.21 mm) than those from moderate-AA and standard-AA diets (0.20 mm). Moderate-AA treatment presented the highest (P = 0.034) shell weight (5.97 g) in comparison to low-AA levels (5.64 g) at 36 wk. Conversely, this last treatment resulted, on average, 0.32 g heavier (P = 0.016) eggshells than moderate-AA and high-AA content (6.46 and 6.47 g, respectively) at 53 wk. Egg analysis showed a linear decrease in shell strength (Shell strength, N = 56.588 −0.169 * AA%; P = 0.002; R2 = 0.50) and elasticity (Elasticity, mm = 0.304−0.0005 * AA%; P = 0.023; R2 = 0.50) at 30 wk, for yolk percentage (Yolk % = 32.580−0.0260 * AA%; P = 0.038; R2 = 0.27), and Y:A ratio at 42 wk (Y:A Ratio = 0.562−0.0007 * AA%; P = 0.002; R2 = 0.32), and for shell weight at 53 wk (Shell weight g = 7.563−0.011 * AA%; P = 0.018; R2 = 0.32). A linear increase in albumen weight at 30 wk (Albumen weight, g = 31.933 + 0.057 *AA%; P = 0.006; R2 = 0.42), elasticity at 36 wk (Elasticity, mm = 0.156 + 0.0006 * AA%; P.
According to the response surface, between 30 and 63 wk of age, shell weight augmented linearly (P = 0.024) with AA levels, and age had a quadratic effect with the heaviest egg shell (P < 0.001) estimated at 53 wk (Shell weight, g = -0.887 + 0.003 * AA% + 0.267 * Age - 0.002 * Age2; R2 = 0.89). The response surface analyses also indicated that yolk percentage decreased linearly (P = 0.001) as AA levels increased in the rearing diets; while, a quadratic response (P < 0.001) was observed as breeders aged, indicating that the maximum yolk percentage was reached at 56 wk of age (Yolk, % = 7.534 −0.012 * AA% + 0.894 * Age - 0.008 * Age2; R2 = 0.92). On the contrary, the albumen percentage had a positive linear response (P = 0.002) as AA content augmented during rearing, and age had a quadratic effect with a maximum percentage (P < 0.001) observed at 54 wk (Albumen, % = 85.374 + 0.014 * AA% - 0.992 * Age + 0.009 *Age2 ; R2 = 0.92). A similar linear response was observed in albumen weight (P = 0.020) with a maximum weight (P < 0.001) detected at 55 wk (Albumen weight, g = 14.024 + 0.025 * AA% + 0.985 * Age - 0.009 * Age2; R2 = 0.71). Consequently, the Y:A ratio was reduced (P = 0.004) as AA levels increased in the rearing diets, with the highest ratio (P< 0.001) at 56 wk of age (Y : A ratio = -0.040 - 0.0003 AA% + 0.021* Age - 0.0002 + Age2; R2 = 0.92).
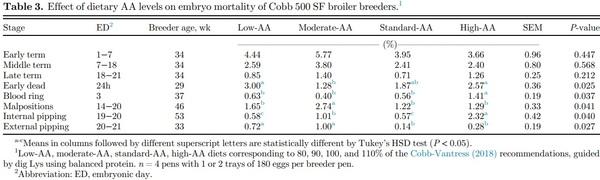
Fertility and Hatchability Fertility and hatchability during the whole reproductive phase on a week-by-week basis were not affected by AA levels in the rearing diets (P > 0.05). However, the highest fertility was observed at 39 wk for low and high-AA with 99.81% each, and at 46 wk for moderate and standard-AA with 99.86 and 99.60%, respectively. The best hatchability by treatment was reached by low-AA diets at 34 wk with 90.80%, moderate-AA at 30 wk with 91.03%, standard-AA at 39 wk with 91.73%, and high-AA at 40 wk with 94.62%. The highest overall flock fertility was observed at 46 wk with 99.69%. Meanwhile, the greatest flock hatchability was observed at 34 wk with 90.71%. Effects of dietary AA during rearing analyzed by response surface indicated that 97% AA levels resulted in the best hatchability (P = 0.046) from 29 up to 50 wk, and age also had a quadratic effect (P = 0.014) with the highest percentage at 38 wk of age (Hatchability, % = 37.331 + 1.944 * AA% 0.010 * AA%2 + 1.707 * Age 0.022 * Age2; R2 = 0.40). A linear effect (P = 0.004) was detected from 51 to 65 wk of age, indicating that hatchability percentage declined as AA levels augmented in rearing diets, and age had a quadratic effect (P = 0.011) with the maximum percentage at 58 wk of age (Hatchability, % = 148.955−0.120 * AA% + 8.525 * Age − 0.073 * Age2; R2 = 0.25). However, no effects of treatments were detected (P > 0.05) on fertility.
EmbryodiagnosticAfter evaluating 15 different incubations and considering each one as a replicate, there were no significant effects (P > 0.05) of AA levels during rearing on early (1−7 d), middle (8−17 d), and late (18−21 d) embryo mortality stages, only sporadic effects of dietary treatments during rearing within specific incubations were observed on mortality at some stages of embryo development. In early dead (24 h) at 29 wk (P = 0.024) effects of treatments were detected. Broiler breeders fed with 80 and 110% AA levels had the highest mortality at 29 wk compared to treatment containing 90% AA. No other significant effects (P > 0.05) on early 24 h nor 48 h dead were observed. Effects in embryo mortality in the blood ring stage were observed only at 37 wk (P = 0.037), where breeders fed during rearing with 110%AA level presented the highest mortality compared to other treatments. Furthermore, mortality due to malposition or turned chicks was significantly different (P = 0.041) at 46 wk. Moderate-AA presented 2 times more this condition (2.74%) than other treatments (1.38% on average). No other effects of treatments (P > 0.05) on this phase, black eye, or feathers period were observed (Table 3). Mortality at internal and external pipping phases presented effects of treatments (P < 0.05) at 53 and 33 wk of age, respectively. Diets with high-AA content produced 1.31% more internal pipping mortality (2.32%) at 53 wk than moderate-AA (1.01%) and 1.75% more than low and standard AA diets that resulted in 0.58%. Contrarily, standard and high AA diets produced less external pipping mortality (0.14 vs. 0.28%) at 33 wk, in comparison to low (0.72%) and moderate AA diets (1.00%), which accounted for the highest mortality. No other significant effects (P > 0.05) due to dietary treatments during rearing in embryo late mortality, contaminated eggs, or cracked shells were observed in the embryo diagnostic.
Incubation at 34 wk was selected to present data of early, middle, and late embryo mortality stages (Table 3) since the highest overall flock hatchability was observed at this week. However, no significant (P > 0.05) effects of dietary AA treatments during rearing were identified either on mortality stages or individual embryonic days. Neither significant effects (P > 0.05) of AA levels and breeders age were detected through response surface analysis.
DISCUSSION
Growth and Body Composition
In this study, heavier breeders resulted from standard and high AA levels at 25, 36, and 40 wk, following the same trend reported by Alfaro-Wisaquillo et al. (2021) during rearing. At 21 wk, pullets fed with 100 and 110% AA content were 3% heavier than those fed with 80 and 90% AA levels. Total feed allocation, and intake of AMEn, Ca, and P up to the onset of egg production decreased as AA increased in the diets, while CP and AA intake increased linearly (Alfaro-Wisaquillo et al., 2021). In previous research reports, no significant effects in breeder BW were reported when using CP levels of 14, 16, and 18% in Cobb 500 (Joseph et al., 2000), high levels (starter 16.65, grower 14.15, prebreeder 15.11%) in Ross 308 (van Emous et al., 2015b), and diets with a reduction on 25% in CP for starter (14.30%), grower (11.76,%), and prebreeder (12.65%) in a pure line (Lesuisse et al., 2017). The present results could be associated with the fact that the pullets probably fulfilled their AA requirements for growth during rearing before photostimulation with a cumulative intake of 58.20 (standard-AA) and 62.58 g (high-AA) of dig Lys, reaching an average BW of 2,607 and 2,661 g, respectively. In contrast, low-AA and moderate-AA resulted in a higher feed supply (115 and 82 g of feed, respectively), only consumed 49.26 (low-AA) and 53.57 g (moderate-AA) of dig Lys, obtaining a BW of 2,542 and 2,572 g for each treatment, respectively.
Results of this experiment during the rearing phase presented by Alfaro-Wisaquillo et al. (2021) reported a reduction of abdominal fat deposition at 15 wk and an increase of relative breast weight at 20 wk on these pullets fed high AA levels compared to low AA content. However, during the laying phase, these effects on breast muscle were not detected. At 25 wk, abdominal fat had a similar result as observed at 15 wk. Low-AA diets resulted in a better fat deposition estimated at 1.18 % compared to high-AA, which presented the lowest abdominal fat at 0.81%. These outcomes agree with those found by van Emous et al. (2013, 2015a). In those studies, using low (Starter 14.59, grower 12.27, and prebreeder 12.79%) and medium levels of CP (Starter 17.96, grower 12.96, and prebreeder 13.96%), with a reduction of 16% and 8% of dig AA, respectively, fat deposition increased finalizing the rearing phase at 20 wk, without effects on the laying phase. In contrast, in diets between -20 and +5% of CP compared to Ross 308 guidelines (2016), Soumeh et al. (2018) found that abdominal fat was reduced linearly as observed in the present experiment.
Negative correlations between BW and RL of small intestine indicated that heavier hens fed with 110% AA level had shorter intestine at 25 wk. In comparison, pullets with longer intestines were fed with 80% AA. These are effects from the rearing phase (Alfaro-Wisaquillo et al., 2021) that continued until the onset of egg production. Probably, longer sections of the intestine (Jejunum and ileum) are an adaptation to lower nutrient availability and vice versa (Taylor and Jones, 2004; de Verdal et al., 2010). Future experiments could evaluate the mechanisms that lead to the changes observed in intestinal length and breast muscle development when feed restricted pullets fed with different AA levels during rearing phase present these modifications on intestinal length along the whole life, develop different nutrient absorption capacity, fat deposition or breast muscle development that can explain a low EP.
Reproductive Performance
Egg Production In this study, the onset of EP was not affected by dietary AA levels during rearing, probably because all treatments reached similar BW before photostimulation with minimum abdominal fat differences. In contrast, Lesuisse et al. (2017) described a reduction in CP of 25% in starter, grower and developer diets (14.30, 11.76, and 12.65% CP) delayed 5 d the onset of production and presented the lowest values for total HHEP and egg mass. Despite the reduction in CP, the levels used by Lesuisse et al. (2017) were only 0.8% lower with respect to our lowest CP content in the 80% AA treatment. No significant differences were observed in the peak of EP. However, 90 and 110% AA reached the peak EP at 30 wk, while hens fed 100% AA during rearing were the last treatment in achieving the maximum EP at 32 wk. van Emous et al. (2015b) did not find effects on the peak of EP when using diets with high (starter 16.65, grower 14.15, and prebreeder 15.11%) or low CP levels (starter 13.81, grower 11.32, and prebreeder 12.62%). In general, 90 and 100% AA content resulted in the best percentage of hen-weekly EP and HHEP at 65 wk. On average, these treatments produced 21.7 eggs more than breeders fed 80% AA levels, which had the lowest HHEP (153.7 eggs). These results are similar to those reported by Joseph et al. (2000), which indicated that EP at 29 wk decreased with rearing diets of 14% CP compared to pullets fed diets with 18% CP. van Emous et al. (2015b) described that low CP (grower 11.32, and prebreeder 12.62%) compared to high CP levels (14.15 and 15.11%) during rearing caused a positive effect with 3 more total eggs (63.6 vs. 66.6) from 45 to 60 wk of age in Ross 308 breeders. Contrarily, the HHEP in the present study was reduced in 9.74 eggs when comparing standard-AA (59.53 eggs) and diets containing 20% less AA (49.79 eggs) during the same period with Cobb 500 hens. Lesuisse et al. (2017), using a pure line, reported a decline of 14.1 total HHEP (69.3 vs. 55.2 eggs) up to 39 wk of age in pullets fed starter, grower, prebreeder, and breeder diets with 14.30, 11.76, 12.65, and 11.55% of CP respectively. Probably these differences in EP and HHEP among van Emous et al. (2015b), Lesuisse et al. (2017), and this study were due to the genetic lines used (Aviagen, 2016; Cobb-Vantress, 2020) and the levels evaluated in each experiment. Da Silva et al. (2015) also reported a linear reduction of EP between 24 and 40 wk of age when increasing Met+Cys levels in isocaloric and isoproteic rearing diets formulated to be deficient in TSAA and supplemented with DL-Methionine to obtain an AA concentration between 0.688 and 0.988% on Cobb 500 pullets. In the study presented here, pullets fed lower AA diets (80% and 90%) produced higher fat deposits at the onset of EP but resulted at the same time, in the best and the worst HHEP at 65 wk (90%: 174.3 vs. 80%:153.7 total eggs), which indicates that the HHEP was not only influenced by fat deposition but probably for breast development (Vignale et al., 2016; Caldas et al., 2018). Despite a linear decrease in the fat deposits with the increase of CP levels, Soumeh et al. (2018) reported no EP or fertility effects, as we observed in this trial.
An average optimum AA level of 95.7% to maximize HHEP was determined by a quadratic broken line and quadratic models. Considering the nutrient intake during rearing up to onset of egg production for this optimum level estimated (Alfaro-Wisaquillo et al., 2021), Cobb 500 SF broiler breeder pullets should consume 23,854 kcal of AMEn, 1,258.2 g of CP, 55.69 g of dig Lys, and 47.99 g of dig TSAA before photostimulation, including the starter phase, to achieve optimum EP. These values are very close to Cobb-Vantress (2020) recommendations that represent 24,388 kcal, 1,341.9 g of CP, 57.13 g of dig Lys, and 47.97 d of dig TSAA. van Emous et al. (2015b) reported that low CP levels improved HHEP with 3 more total eggs from 45 to 60 wk in Ross 308, with a cumulative intake of 31,015 kcal and 1,488 g of CP per pullet during rearing phase from 2 up to 22 wk of age. The consumption of energy augmented 12.8%, and CP declined 3.7% to reach the target BW compared to high CP diets (27,515 Kcal, and 1,545 g CP). In the results presented herein, AMEn consumption between 2 and 22 wk of age was estimated at 26,878 kcal for low-AA treatment, 26,788 kcal for moderate-AA, 26,643 kcal for standard-AA, and 26,412 for high-AA diets. As observed in the outcomes presented by van Emous (2015b), pullets fed lower CP diets consumed more energy due to the increase of feed allowance to achieve target BW. However, the feed allocation until 22 wk in this study ranged 167 g from the 80% AA (9,930 g) to 110% AA (9,763 g), while the difference expressed by van Emous (2015b) represent 1,320 g between treatments (11,630 vs. 10,310 g).
Egg Composition and Egg Quality During the present experiment, higher AA levels resulted in larger eggs, specifically in early and late production at 26, 28, and 51 wk. This result followed a similar finding reported previously (Summers, 1993; Summers and Leeson, 1994; Joseph et al., 2000), where hens fed high CP levels laid heavier eggs in the early laying period due to an increased BW or albumen weight. The lightest egg weight was obtained with 89% AA in the whole cycle, and the maximum weight was achieved at 54 wk. The results of this experiment could be associated with the fact that high CP and AA levels could improve albumen and consequently increasing egg size (Ekmay et al., 2013). Breeders fed 90 and 100% AA levels during rearing produced more eggs and laid lighter eggs than breeders fed with 80 and 110% AA. Yolk percentage, albumen percentage, and weight, and Y:A ratio were linearly affected by AA levels fed during rearing, with the quadratic effect of age. Higher Y:A ratio is essential for embryo development and hatch due to the yolk function as suppliers of lipids, water, minerals, protein, and other nutrients to guarantee adequate embryo growth (Uni et al., 2012; Nasr, 2015). Albumen percentage and weight increased as AA levels increased, while yolk percentage and Y:A ratio decreased in the same way. A reduction on Y:A ratio indicates lower availability of fatty acids, which are considered the main source of energy for the embryo (Nasr, 2015), and consequently could affect the hatchability and performance of the offspring (Ulmer-Franco et al., 2010). Conversely, Lesuisse et al., 2017 using low CP levels (14.30, 11.76, 12.65, and 11.55%), did not find effects on yolk percentage and Y:A ratio, but albumen percentage decreased 1.6% at 32 wk of age (57.9 vs. 56.3%) enhancing progeny BW.
On the other hand, shell strength and yolk color were affected linearly as AA increased in rearing diets and shell weight and elasticity in a quadratic way. Jiang et al. (2019) reported a linear decrease in the eggshell percentage, thickness, and strength in broiler breeders supplemented with Thr ranging from 0.50 up to 0.98% of total dietary content in the laying phase from 28 to 38 wk of age. However, these levels were higher than those used in the experimental rearing diets and standard layer diets in our experiment. In this study, TSAA:Lys ratios ranged from 0.97 to 0.84 in grower and from 0.98 to 0.86 in developer, decreasing as dietary AA content increased. This result can explain the better eggshell quality parameters observed at 30 and 53 wk since some authors have suggested that increasing TSAA:Lys ratios from 0.82 to 0.97 can supply the sulfur AA requirements for the shell protein matrix synthesis, and improve the Ca binding ability to obtain better eggshell quality (Novak et al., 2006; NassiriMoghaddam et al., 2012). Joseph et al. (2000) indicated that the eggshell weight increased by CP content of 18% during rearing in Cobb 500 broiler breeders. Diets reduced in CP from 3 up to 40 wk of age (14.30, 11.76, 12.65, and 11.55 % CP) in a pure breeder line impaired shell weight, but this reduction did not affect the thickness, yolk weight, and albumen height (Lesuisse et al., 2017) at 26, 32, and 39 wk, as it was observed in the present study.
Fertility and Hatchability Dietary treatments did not influence fertility during rearing, similar to findings reported by van Emous et al. (2015a) using different growth patterns and high (19.21, 14.13, and 14.88%), medium (17.96, 12.96, and 13.96%), and low levels of CP (14.59, 12.27, and 12.79%) on starter, grower and prebreeder diets. These findings could be related to an adequate feed allocation and cumulative intake of AA, energy, and other nutrients at 20 wk that permitted to reach the target BW, producing persistence on fertility, as suggested by Walsh and Brake (1997). In the present experiment, the best fertility was at 39 wk, with 99.81% for low and high-AA, and at 46 wk 99.73% on average for breeders fed moderate and standard-AA without observing significant statistical differences among treatments. Walsh and Brake (1997, 1999) described an improvement in fertility on broiler breeders fed diets with 15.7% of CP and the Lys:(Met+Cys) ratio of 1:0.85 on the growing phase. The results presented herein demonstrated that hatchability increased quadratically between 29 and 50 wk with TSAA ranging from 0.55 to 0.64% in grower and 0.57 to 0.67% in the developer. Using higher TSAA levels from 0.688 to 0.928%, Da Silva et al. (2015) detected a reduction of hatchability at 40 wk in Cobb 500 pullets at levels of 0.928% TSAA. Conversely, van Emous et al. (2015a) observed better fertility of 94.4% and hatchability of 92.2% of fertile eggs on Ross 308 breeders fed rearing diets with high CP content (19.21, 14.13, and 14.88%). Still, medium CP levels (17.96, 12.96, and 13.96%) presented lower hatchability estimated in 87.9%. Another report by van Emous et al. (2015b) described effects in breeders fed rearing diets with a reduction on CP (13.81, 11.32, and 12.62%) that presented the best fertility in 92.2% and hatchability of 89% with respect to breeders fed high CP levels (16.6, 14.1, 15.1%) that resulted on 91.1% and 87.7%, respectively. But, Lesuisse et al. (2017) reported that decreasing 25% the CP with inclusion of 14.30, 11.76, 12.65, and 11.55 % reduced fertility by 14.5% (84.9 vs. 70.4) without effects on hatchability of fertile eggs. However, this experiment indicated that regardless of AA level evaluated during rearing, fertility was not affected, and the results were close to standards indicated by Cobb-Vantress guidelines (2020).
As the breeder aged, the eggs were larger; usually, this is influenced by larger yolk size compared to albumen. Conversely, in this study, a linear effect of AA increased the albumen and decreased yolk percentage, leading to a linear decrease in the Y:A ratio. Consequently, a quadratic response was observed on hatchability between 29 and 50 wk of age, showing a decline in this parameter when pullets were fed diets with more than 97% AA during rearing and a linear decrease from 51 wk onward. An increase in albumen percentage could have affected the gas exchange between oxygen and carbon dioxide, yolk absorption, and oxidation of fatty acids causing high late embryo mortality as observed in young hens (Araujo et al. , 2015). Similarly, a reduction in yolk percentage meant fewer fatty acids available for the embryo, mainly in the days of incubation, decreasing the energy necessary to hatch and less fat reserves for later use during growth (Uni et al., 2012). Additionally, to the effects of AA levels in eggshell traits and Y:A ratio, hatchability and embryo development could be influenced by other egg traits. The optimum seems to be obtained with diets containing 90 and 100% AA during rearing. However, machine conditions also played a role in hatchability and should be considered to interpret the results of this parameter.
Embryodiagnostic The dietary AA levels during rearing did not affect early, middle, and late embryo mortality in any of the 15 incubations that were conducted. But, some sporadic effects of treatments were observed on some phases of embryo development. For example, the highest early dead at 24 h was found at 29 wk on hens fed 80 and 110% AA content. This last treatment had the highest mortality rate in the blood ring stage (3 d) at 37 wk. Mortality by malposition was related to 90% AA during rearing, and only standard AA levels resulted in less late mortality. van Emous et al. (2015b) found in Ross 308 hens between 22 to 45 wk of age, that pullets fed low CP (starter 13.81, grower 11.32, and prebreeder 12.62%) content during rearing showed a 0.9% less embryo mortality from incubation d 10 onward, compared to high CP levels (starter 16.65, grower 14.15, and prebreeder 15.11%). However, in another study by the same author (van Emous et al., 2015a), when hens received medium levels of CP on rearing diets (starter 17.96, grower 12.96, and prebreeder 13.96%), higher average embryo mortality of 3.2% was observed in the late stage of incubation from 18 to 21 d as compared to diets with a reduction of 10% (14.59, 12.27, and 12.79%) or uplift of 7.5% of CP (19.21, 14.13, and 14.88%). Those outcomes were associated with high incubation temperature, resulting in embryo respiratory failure, malposition, and abnormalities.
In conclusion, the hypothesis formulated is accepted since AA levels evaluated during rearing demonstrated to affect BW and reproductive performance except for fertility. Standard and high AA treatments resulted in heavier pullets with less abdominal fat deposition at the end of the rearing phase and did not seem to affect EP directly. The onset of lay was not affected by the dietary AA content, but peak production was reached at 30 wk with 90 and 110% AA. Moderate and standard AA diets produced the best HHEP. In this experiment, the optimum AA levels to maximize HHEP were estimated to be 94.7% and 96.7% of Cobb-Vantress (2018) recommendations through quadratic broken line regression and quadratic models, respectively. Rearing diets with an estimate of 89% of AA resulted in the lightest eggs, and high-AA levels caused an improvement in eggshell quality parameters. Additionally, as AA levels increased during rearing, the yolk percentage and Y:A ratio decreased, and albumen weight and the percentage increased. No treatment effects were observed on fertility, but hatchability was enhanced up to 50 wk of age with 97% of AA, from 51 to 65 wk was linearly reduced.