Introduction
Under normal commercial conditions, poultry tend to eat to satisfy their energy needs, thus dietary energy level drives feed intake, which in turn, is the basis of diet formulation. Feed is the major cost for poultry production and accurate diet formulation is therefore of paramount importance to the profitability of poultry enterprises.
The metabolisable energy (ME) bioassay is used as the default system of determining dietary energy values from feed ingredients for poultry in most countries (Bourdillon et al., 1990; Farrell, 1999). However, despite the best effort across the globe to standardise the AME assay, the energy values obtained using the AME system vary tremendously, due to factors including source of ingredients, age and strain of birds, geographical location where the ingredients are grown, and laboratories where the assay is conducted (Sibbald, 1976; Farrell, 1978; Leeson and Summers, 2005; Lopez et al., 2007). The economic cost of feed energy variation to the poultry industry must therefore be great but is extremely difficult to assess.
Of course, other systems, such as the Fraps’ (1946) productive effective energy system, Emmans’ (1994) effective energy system, and the net energy system (Nehring and Haenlein, 1973; de Groote, 1974), have been proposed but their practical implementation has not been widespread. In the meantime, many attempts have been made to improve the accuracy of dietary energy measurements and the topic has been the subject of many in-depth reviews (Sibbald, 1980; Pirzgoliev and Rose, 1999; Farrell, 1999; Lopez and Leeson, 2008). The key debates about dietary energy evaluation in poultry are concerned with (a) bioassays of metabolisable energy (Davidson et al., 1957; Hill and Anderson, 1958; Sibbald, 1980; Härtel, 1986); (b) corrections applied to ME values, i.e., zero N balance (Sibbald and Wolynetz, 1986; van der Klis et al., 2010), endogenous losses (Farrell, 1981; Sibbald, 1985; Härtel, 1986), and extra caloric effect of lipids (Sell et al., 1976; van der Klis and Fledderus, 2007); (c) prediction of ME values, i.e., through chemical composition (Fisher, 1982; Carré, 1990; Bourdillon et al., 1990), using indicators (Scott and Boldaji, 1997; Scott and Hall, 1998); via in vitro digestibility assays (Carré and Brillouet, 1986; Valdes and Leeson, 1992a), applying near infrared reflectance spectroscopy (Valdes and Leeson, 1992b, Black et al., 2009), and devising mathematical models (Emmans, 1994; Gous, 2010).
Indeed, energy evaluation is a topic of immense interest, and at times, of great controversy. This paper will not attempt to review “the reviews” on the topic, nor will it discuss the merit or otherwise of the various AME systems. Rather it will summarise published data on energy variability of individual ingredients, and outline the potential usefulness of a net energy system. Also included is discussion on the process of setting up a closed-circuit calorimetric system for the determination of the net energy values of feedstuffs for broiler chickens.
The two sides of metabolisable energy variation
The ingredients
In practice, the ME values of feedstuffs are tabulated so that they can be used as the basis of feed formulation. By doing so, the values of different feed ingredients are treated as if they were completely additive. This would be acceptable if the ME value were the sole characteristic of the feed. Indeed, the ME value is derived from the interaction between a feed ingredient and an animal, and therefore it reflects variation arising from both the animal and the feed. In fact, it is incorrect to obtain a diet ME value by adding up the individual ME values of the feed ingredients used to make that diet.
Throughout the years, much research has focussed on making the ME value of an ingredient “less variable”, and hence “more consistent” across different ages and classes of poultry. The use of a true metabolisable energy (TME) system or to apply N correction to ME values are two prime examples of such attempts. The biological relevance and the need for various corrections to the ME systems have been questioned (Vohra, 1972; Farrell et al., 1991).
Variability in the ME content of feedstuffs is natural. Table 1 shows the variation of ME values of selected feed ingredients in broilers and layers (Black et al., 2005). The ME trials for broilers and layers were conducted over a 7-day period, with broilers starting on day 22, and hens starting in mid-lay, respectively. The mean daily intakes ranged from 104g to 108g per broiler bird and 115g to 120g per laying hen. There was no correlation between feed intake and ME values. As previously mentioned, the ME values of ingredients vary due to many factors, however, in this study, the variation arising from age and strain of bird, level of feeding, feed processing, feed additives, methodology, laboratory, and other environmental factors was eliminated. Thus, the large variation displayed within a cereal grain would have come primarily from two sources, i.e., cereal variety and geographical locations where the grains were grown.
Table 1 - Ranges in ME values (kcal/kg dry matter) of selected cereal grains in laying hens and broiler chickens (Black et al., 2005).
Purely from the ingredient point of view, for viscous grains such as wheat, barley, triticale, oats and rye the most important chemical component causing such variation in their ME values is the content of soluble non-starch polysaccharides (NSPs) (Choct and Annison, 1990; Annison, 1991) (Figure 1). This is because soluble NSPs have antinutritive activities in poultry diets, reducing nutrient digestion and absorption, in particular, starch, protein and lipids (Choct and Annison, 1992a,b).
In non-viscous grains such as corn, sorghum and rice, the cell wall architecture and constituents (Black et al., 2005; Gidley et al., 2011) and the rate of starch digestion (Weurding et al., 2003) have been suggested as possible reasons for variation in their energy content. D’Alfonso (2003) reported the ileal digestibility values for the starch, protein, lipid and energy of 93 corn samples in broilers. The ranges in starch, protein and lipid contents were: 65.8 to 72%, 7.1 to 9.7%, and 3.5 to 5.9%, respectively. The average ileal digestible energy value was 3205 kcal/kg DM with a standard deviation of 488kcal/ kg DM. Starch digestibility rate of the samples was measured in vitro and reported to vary between 37% to 53%. This appears to suggest that some of the corn samples contained starch that was not digested rapidly in the small intestine. This may have a number of implications.
Figure 1 - Relationship between NSP level and energy metabolisability in broiler chickens (Choct and Annison, 1992a).
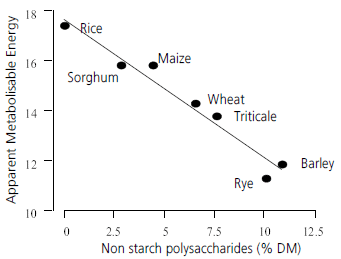
First, starch that is not digested in the small intestine will end up in the caeca. The energetic efficiency of using starch via enzymatic digestion vs. microbial digestion is vastly different. Carré et al., (1995) demonstrated that the net efficiency of utilising digestible energy via hind gut fermentation, compared with being absorbed as glucose in the upper gut, was 65% in cockerels and 50% in broilers. Furthermore, it is questionable whether starch entering the caeca is fermented to yield available energy. Weurding et al., (2001) conducted a comprehensive study where the site of digestion of starch contained in various ingredients differing widely in the rate of digestion. Their findings revealed that there was hardly any difference in starch digestibility values between the posterior ileum and the total tract, regardless of the starch source and its rate of digestion. This demonstrates that the caeca may play little role in starch digestion even if a large amount of starch ends up in them.
There are numerous factors that affect the rate of starch digestion, including the granular size and structure of starch (Moran, 1982), amylose to amylopectin ratio (Zhou et al., 2010), formation of resistant starch due to processing (Hoover and Manuel, 1996; Ito et al., 1999; Creswell and Bedford, 2006), and formation of annealed starch (Leszcynski, 2004).
Second, it is proposed that cell walls composed mainly of insoluble NSPs together with some proteins, phenolics and phytate encapsulate nutrients, such as starch and lipids, to act as a physical barrier to endogenous enzymes reaching these substrates in a timely manner (Choct, 2010). Within the short resident time of digesta in poultry, this can affect nutrient digestion in the upper part of the digestive tract, leading to reduced energy availability to the animal. In the case of sorghum, !¨-kafrins make up a small part of the proteins but are highly resistant to digestion due to their sulphur cross links (Klopfenstein and Hoseney, 1995).
Phytate and tannins are other possible factors that contribute to the variability of energy content of sorghum although the samples used in Black et al., (2005) were known to contain little or no condensed tannins (Bryden et al., 2009). Indeed, Black et al., (2005) concluded that neither tannins nor phytate had anything to do with the available energy in sorghum.
The bird
Hughes and Choct (1997) conducted a study using broiler chickens from the same hatch reared under identical conditions. A single sample of wheat was fed to 48 individually-housed broiler chickens at day 24. The ME trial lasted a week with the first three days acting as an adaptation period and the last four days as the excreta collection period. This was to test whether drastically increasing the number of replicates (to 48 in this case) would improve the precision of ME measurement. The results showed that individual birds displayed tremendous variation with the AME value of that particular wheat sample (kcal/kg DM) ranging from 2294 to 3513. Table 2 shows the AME value, feed intake, dry excreta output and excreta energy content of five birds per group showing high, medium and low AME values.
Table 2 - Variability in characteristics of excreta from five chickens showing high, medium and low responses to a single wheat sample.
Table 2 reveals an interesting picture. The high responders ate less, excreted less and retained more of the dietary energy (less excreta energy), whereas the reverse was true for the low responders. Another experiment conducted at the same time demonstrated that the addition of a carbohydrase cocktail to the same diet significantly lifted the average AME value of the wheat, while reducing the variation three fold. What this seems to indicate is that the main factor contributing to this large between-bird variability is due to the anti-nutritive action of NSPs. Why some individual birds can cope with NSPs whilst others from the same hatch cannot is not understood. This is one of many known and unknown examples of diet x bird interactions that affect ME.
Indeed, it is not surprising that genetic factors affect energy metabolism. Mignon-Grasteau et al., (2004) demonstrated a 13% difference in AMEn of wheat by selecting broilers based on their ability to use wheat. In a more recent study, Moore et al., (2011) reported that high and low performing broiler chickens differentially expressed some genes. They showed that 1,372 genes were more highly expressed in high performing birds, compared with 231 genes that were higher in the low performing birds. In the same study, the researchers also found that 26 bacterial communities were differentially abundant between the good and poor performing birds. It has not been demonstrated whether these findings are repeatable in birds that come from different hatches and which are reared at different locations. Nevertheless, this demonstrates the complexity of energy metabolism in birds where a large proportion of variation in energy content of feed may arise from the bird alone, which, in turn, is linked to host gene expression and gut microflora composition. Indeed, there seems to be a lack of correlation between the ME content of the diet and bird performance, such as feed conversion efficiency (Scott et al., 1999; Black et al., 2005). In general, the measurement of feed conversion efficiency (FCR) is straightforward and accurate. Thus, the lack of correlation between ME and FCR would point to the fact that the inaccuracy may arise from the ME side of the equation.
To obtain some answers to the questions regarding between bird-variation and the correlation between available energy and bird performance, it is important to have an energy evaluation system that can differentiate between energy used for maintenance and that required for production and reproduction, with the possibility of determining heat increment (HI) of feeding as well as partitioning of energy into fat and protein deposition. Would a net energy system, for instance, be less variable and thus give a more accurate estimation of dietary energy for poultry?
It is hardly surprising that there is a significant difference in the nutritive value between varieties of the same ingredient (e.g., wheat). But in practical diet formulation, such variation is often ignored because there is no rapid, real time system to determine individual feed ingredients entering the feed mill. Furthermore, it is possible that some feed additives, such as enzymes, may elicit a significant nutrient and energy sparing effect in poultry. Unless the net utilisation of energy and nutrients is determined, such an effect is not clearly known (Choct, 2010; Pirgozliev et al., 2011).
Setting up a net energy test facility
Background
The metabolisable energy system measures the loss of energy between ingestion and excretion, rather than the true availability of energy for maintenance and production. MacLeod (1994) argued that the simplicity and repeatability of the ME system are achieved at the cost of ignoring the metabolic fate of absorbed nutrients. Another concern with the ME system is that the values obtained for individual ingredients are assumed to be additive when formulating a diet whereas in reality they are not. This is because anti-nutrients from one ingredient will affect the available energy of another in the complex ecosystem of the gut. Other systems, such as the Fraps’ “Productive Energy System” (Fraps, 1946), which was based on comparative slaughter technique, and the Emmans’ “Effective Energy System” (Emmans, 1994), which takes into account the energy costs required to process a diet, are in effect variants of the net energy system. Although these systems still cannot address the additivity issue, they eliminate an important source of variation in the ME system, HI of feeding, thus should provide a better system for energy evaluation.
The heat loss in birds fed different feedstuffs is not proportional to the AME values of those feedstuffs. An accurate measurement of HI is therefore the cornerstone of a robust net energy system. There are several ways to measure HI. It can be calculated as the difference between ME intake and energy gain as body tissues via comparative slaughter; measured using whole animal calorimetry, i.e., directly measuring the heat given off during feeding; or determined using indirect calorimetry. Indirect calorimetry is the most common technique where heat production is calculated from the amount of oxygen consumed and the amount of carbon dioxide expired by the animal within a set environment and timeframe.
The net energy facility now established at the University of New England (UNE) is an indirect calorimetry system using closed-circuit respiratory chambers.
Indirect calorimetry
The respiratory chamber can be open-circuit or closed-circuit. The former allows air to enter the chamber and oxygen consumption is then calculated using the oxygen content in air, i.e., 21%, and measuring that in the volume of air leaving the chamber. This can be done in two ways. Some researchers remove the CO2 from the air entering the chamber and then trap the CO2 generated in the chamber by the animal before measuring the amount of oxygen exiting the chamber, whereas others measure it without removing the CO2 from the air entering the chamber or generated by the animal.
In an open-circuit system, depending on the size of the chamber relative to the size of the animal, a real time measurement of oxygen consumption requires a very high precision in measuring oxygen volumes on both ends of the chamber. As pointed out by Arch et al., (2006), a 1% error in calibration of one of the flow meters relative to the other would result in an error of at least 21% in the calculated value of oxygen consumption. Such a system would be less suited for an accurate measurement of oxygen consumption and CO2 output from a chicken in a dynamic manner. This is because (a) the chamber needs to be quite large to cater for physical activity of the bird, and (b) the amount of air exhaled by a chicken is very small relative to the size of the chamber and it is difficult to guarantee a steady flow of the expired CO2 through the chamber, which is then accurately measured. For example, a 1.6 kg female chicken breathes 23 times per min with a tidal volume of 33 mL per breath (Fedde, 1993), giving a total volume of 759 mL expired breaths. In a chamber measuring 100cm x 70cm x 65 cm like the system at UNE, the amount of air expired by a bird accounts for only 0.167% of the chamber volume. According to Freeman (1984), expired chicken breath contains approximately 5% CO2, so the amount of CO2 is 0.00835% in the chamber in any given minute if all the air is homogenously mixed. This makes accurate measurement of expired CO2 even on an extended period, say, every 4 hours, a challenging task. Of course, the accuracy of an open-circuit system can be enhanced by increasing the number of birds housed in the chamber, provided that the humidity of the chamber is properly controlled. Alternatively, all expired CO2 may be trapped over a 24h period and then measured accurately by gravimetry.
On the other hand, a closed-circuit system is a highly accurate alternative. As the name suggests, a closed-circuit system is not open to the outside air. The animal is housed in a closed chamber where it consumes the oxygen and produces CO2. At one end of the chamber, there is a supply of pure oxygen via a well-calibrated flow meter and at the other end there is a pump that draws out the chamber air. The exited air is then desiccated and all the CO2 produced by the animal is trapped. This removal of expired CO2 causes a drop in the chamber pressure, activating a solenoid valve to replenish the chamber with oxygen. Since a typical run lasts 22 hours, the amount of CO2 trapped over the period is high and the error that could potentially arise from measuring a very small amount of CO2 continuously is avoided. The fact that 3-4 runs per bird with 4-6 replicates per treatment are used makes the closed-circuit system very accurate and reliable.
The design of the calorimeter
To set up a closed-circuit respiratory chamber, the following must be considered: (a) the size of the chamber to cater for sufficient activity by the bird; (b) an appropriate air flow through the chamber so that CO2 expired by the bird is removed in a steady stream whilst maintaining a proper chamber pressure; (c) an electronic control system capable of detecting minute pressure changes in the chamber so that inspired oxygen is replenished constantly; (d) a CO2 trap that is sufficient to absorb all expired CO2 without affecting air circulation through the chamber; (e) a desiccating devise that removes all the moisture generated by the bird; and finally but extremely importantly, the whole system that incorporates feeders, drinkers, and an excreta collection tray must be completely air-tight.
In addition, the chamber must be located in an environment-controlled room where the temperature is kept within the thermo-neutral zone of the bird, preferably within 2 oC of the most comfortable temperature setting for the bird. Logistical issues, such as the ease of operation in terms of the time taken to change over various parts of the chamber after a 22-hour run, including the collection of excreta, collection of absorbed CO2, renewal of the desiccant, measurement of oxygen intake, and re-sealing of the chamber etc., must also be considered. The closed-circuit system at UNE has 24 chambers. The dimension of each chamber is 100cm long x 70cm wide x 65cm high, with sufficient space to house 2-4 birds below commercial stocking density.
Calibration of a closed-circuit calorimeter
A sufficient number of chambers is required to avoid the introduction of errors associated with timing or stacking of different runs of NE trials for a single diet. A control diet must accompany each run. Multiple chambers mean that calibration is required not only of each chamber, but also cross validation of all chambers. The trials at UNE used an ethanol lamp (100mL pure ethanol) to validate each chamber. When a carbohydrate is completely oxidised, the following is expected:
CxHyOz + (x + y/4 - z/2) O2 ---> x CO2 + (y/2) H2O
The molecular formula for ethanol is C2H6O. Using the above formula, the RQ is x/ (x + y/4 - z/2) = 2/(2 + 6/4 - 1/2) = 2/3 " 0.667.
Indeed, the initial 16 chambers in use yielded the same RQ value of 0.667.
The conduct of an NE trial
Heat increment of a given diet varies if the range in ME intake is wide (Noblet et al., 2010). Thus, it is important that HI is measured under a reasonably stable condition, i.e., using birds with similar feed intakes and at similar ages. Fortunately, broilers at 20-30 days of age provide a good model for NE determination as they achieve ad libitum feed intake and in positive N balance. In the UNE NE facility, all birds are reared from day-old and then are transferred to the chambers for adaptation at day 22 for start of the NE trial on day 25. Each diet uses 4-6 chambers and each chamber is repeated over 3 runs of 22 hours per run. At the end of the trial, a 4th run is conducted overnight to determine “Fasting Heat Production”. For each trial a control diet, which has been tested for its HI and NE values in every chamber, is included to keep consistency between trials.
The practical objective
Fraps (1946) produced a set of productive energy values for 62 feedstuffs using the comparative slaughter technique in poultry. The data set was meticulous and comprehensive. Since its release, however, it has attracted an enormous amount of scrutiny and much work has been conducted, not to generate more NE data, rather to examine whether an NE system would offer a better alternative to the various ME systems (Hill and Anderson, 1958; de Groote, 1974; Farrell, 1974; MacLeod, 1994; Emmans, 1994). It is recognised that since HI is affected by chemical composition of the feed, the physical activity level of the bird and feed intake, it is not possible to tabulate HI, and hence NE, values for individual ingredients directly through conducting NE trials.
Noblet et al., (1994) measured the HI values of 61 diets for growing pigs that met the nutritional requirements of the animals used but differed widely in the levels of various constituents, including fat, protein, fibre, and starch. The resulting data were used to establish NE prediction equations. These equations have been used under practical conditions in a number of pig companies around the world. The strength of the approach is that the NE data generated arose from nutritionally balanced experiments under near commercial conditions, taking into account true differences attributable to the chemical and nutritional characteristics of the diets. Another important point is that the equations rely on parameters that are relatively easy to determine. A similar approach in poultry may prove economically beneficial.
Conclusion
Energy cost will only increase as more feedstock is diverted to the production of biofuels and more areas of the world are affected by frequent droughts and floods arising from climate change. Here is a quote from Pirzgoliev and Rose (1999): “Modern poultry meat production is now highly competitive and a small difference in the efficiency of utilisation of the supplied feed can be economically significant. Although ME systems have given estimates of energy availability that have acceptably low variability and high repeatability, it is possible that further process in describing and understanding the differences in energy availability between feeds may only be achieved by using an NE system”.
References
ANNISON, G. (1991) Relationship between the levels of soluble nonstarch polysaccharides and the apparent metabolizable energy of wheats assayed in broiler chickens. Journal of Agriculture and Food Chemistry, 39:1252-1256.
ARCH, J.R.S., HISLOP, D., WANG, S.J.Y. and SPEAKMAN, J.R. (2006) Some mathematical and technical issues in the measurement and interpretation of open-circuit indirect calorimetry in small animals. International Journal of Obesity 30:1322-1331.
BLACK, J.L., HUGHES, R.J., NIELSEN, S.G., TREDREA, A. M., MCALPINE, R. and VAN BARNEVELD, R.J. (2005) The energy value of cereal grains, particularly wheat and sorghum, for poultry. Australian Poultry Science Symposium 17:21-29.
BLACK, J.L., HUGHES, R.J., NIELSEN, S.G. TREDREA, A.M. and FLINN, P.C. (2009) Near infrared reflectance analysis of grains to estimate nutritional value for chickens. Australian Poultry Science Symposium 20:31-34.
BOURDILLON, A., CARRÉ, B., COGNAN, L., HUYGHEBAERT, G., LECLERCQ, B., LESSIRE, M., MCNAB, J. and WISEMAN, J. (1990) European reference method for the in vivo determination of metabolisable energy with adult cockerels: Reproducibility, effect of food intake and comparison with individual lab methods. British Poultry Science 31:557-565.
BRYDEN, W.L., SELLE, P.H., CADOGAN, D.J., LI X., MULLER, N.D., JORDAN, D.R., GIDLEY, M.J. and HAMILTON, W.D. (2009) A Review of the nutritive value of sorghum for broilers. Rural Industries Research and Development Corporation (RIRDC) Publication No 09/077, Canberra, Australia.
CARRÉ, B. (1990) Predicting the dietary energy value of poultry feeds, in: WISEMAN, J. and COLE, D. J. A. (Eds) Feedstuff Evaluation, pp. 283-300, Butterworths, London.
CARRÉ, B. and BRILLOUET, J.M. (1986) Yield and composition of cell wall residues isolated from various feedstuffs used for non-ruminant farm animals. Journal of the Science of Food and Agriculture 37: 341-351.
CARRÉ, B., GOMEZ, J. and CHAGNEAU, A.M. (1995) Contribution of oligosaccharide and polysaccharide digestion, and excreta losses of lactic acid and short chain fatty acids, to dietary metabolisable energy values in broiler chickens and adult cockerels. British Poultry Journal 36:611-629.
CHOCT, M. (2010) Feed polysaccharides: nutritional roles and effect of enzymes. http://en.engormix.com/MApoultry- industry/genetic/articles/feed-enzymes-inpoultry- nutrition-t1603/103-p0.htm
CHOCT, M. and ANNISON, G. (1990) Anti-nutritive activity of wheat pentosans in broiler diets. British Poultry Science 31:811-821.
CHOCT, M. and ANNISON, G. (1992a) Anti-nutritive effect of wheat pentosans in broiler chickens: roles of viscosity and gut microflora. British Poultry Science 33:821-834.
CHOCT, M. and ANNISON, G. (1992b) The inhibition of nutrient digestion by wheat pentosans. British Journal of Nutrition 67:123-132.
CRESWELL, D. and BEDFORD, M.R. (2006) High pelleting temperatures reduce broiler performance. Australian Poultry Science Symposium 18:1-6.
D’ALFONSO, T.H. (2003) Factors affecting ileal digestible energy of corn in poultry diets. Proceedings of the Recent Advances in Animal Nutrition in Australia 14:151-156.
DAVIDSON, J., MCDONALD, I. and WILLIAMS, R.B. (1957) The utilisation of dietary energy by poultry. I. A study of the algebraic method for determining the productive energy of poultry foods. Journal of the Science of Food and Agriculture 8: 173-81.
DE GROOTE, G. (1974) A comparison of a new net energy system with the metabolisable energy system in broiler diet formulation, performance and profitability. British Poultry Science 15:75-95.
EMMANS, G.C. (1994) Effective energy: a concept of energy utilization applied across species. British Journal of Nutrition 71:801-821.
FARRELL, D.J. (1974) Effects of dietary energy concentration on utilisation of energy by broiler chickens and on body composition determined by carcass analysis and predicted using tritium. British Poultry Science 15:25-41.
FARRELL, D.J. (1978) Rapid determination of metabolisable energy of foods using cockerels. British Poultry Science 19: 303-308.
FARRELL, D.J. (1981) An assessment of quick bioassays for determining the true metabolizable energy and apparent metabolizable energy of poultry feedstuffs. World’s Poultry Science Journal 37:72-83.
FARRELL, D.J. (1999) In vivo and in vitro techniques for the assessment of the energy content of feed grains for poultry: a review. Australian Journal of Agricultural Research 50:881-888.
FARRELL, D.J., THOMSON, E., DU PREEZ, J.J. and HAYES, J.P. (1991) The estimation of endogenous excreta and the measurement of metabolisable energy in poultry feedstuffs using four feeding systems, four assay methods and four diets. British Poultry Science 32: 483-499.
FEDDE, M.R. (1993) Respiration in birds, in: SWENSON, M.J. and REESE, W.O. (Eds) Dukes’ Physiology of Domestic Animals, 11th Edition, pp. 294-302 Cornell University Press, Ithaca, USA.
FISHER, C. (1982) Energy evaluation of poultry rations, in: HARESIGN, W. (Ed) Recent Advances in Animal Nutrition, pp. 113-39, Butterworths, London. FRAPS, G.S. (1946) Composition and productive energy of poultry feeds and rations. Texas Agricultural Experiment Station Bulletin No. 571.
FREEMAN, B.M. (1984) Physiology and Biochemistry of the Domestic Fowl, Volume 5, p.423. Academic Press, London.
GIDLEY, M.J., FLANAGAN, B.M., SHARPE, K. and SOPADE, P.A. (2011) Starch digestion in monogastrics – mechanisms and opportunities. Proceedings of the Recent Advances in Animal Nutrition – Australia 18: 207-213.
GOUS, R.M. (2010) An effective alternative to the metabolisable energy system. Australian Poultry Science Symposium 21:36-43.
HÄRTEL, H. (1986) Influence of food input and procedure of determination on metabolisable energy and digestibility of a diet measured with young and adult birds. British Poultry Science 27:11-39.
HILL, W.F. and ANDERSON, D.L. (1958) Comparison of metabolisable energy and productive energy determinations with growing chicks. Journal of Nutrition 64:587-603.
HOOVER, R. and MANUEL, H. (1996) The effect of heat-moisture treatment on the structure and physicochemical properties of normal maize, waxy maize, dull waxy maize and amylomaize V starches. Journal of Cereal Science 23:153-162.
HUGHES, R.J. and CHOCT, M. (1997) Low-ME wheat or low-ME chickens? – highly variable responses by birds on the same low-ME wheat diet. Australian Poultry Science Symposium 9:138-141.
ITO, T., SAITO, K., SUGAWARA, M., MOCHIDA, K. and NAKAKUKI, T. (1999) Effect of raw and heatmoisture- treated high-amylose corn starches on the process of digestion in the rat digestive tract. Journal of the Science of Food and Agriculture 79:1203-1207.
KLOPFENSTEIN, C.F. and HOSENEY, R.C. (1995) Sorghum and millets: chemistry and technology., in: DENDY, D. A. V. (Ed) American Association of Cereal Chemists, pp. 125-169, St. Paul, USA.
LEESON, S. and SUMMERS, D.J. (2005) Feed programs for broiler chickens. Commercial Poultry Nutrition, 3rd Edition, pp. 229-296, University Books, Guelph, Ontario, Canada. LESZCYNSKI, W. (2004) Resistant starch – classification, structure, production. Polish Journal of Food and Nutrition Sciences 13/54 (SI 1):37-50.
MIGNON-GRASTEAU, S., MULEY, N., BASTIANELLI, D., GOMEZ, J., PÉRON, A., SELLIER, N., MILLET, N., BESNARD, J., HALLOUIS, J-M. and CARRÉ, B. (2004) Heritability of digestiblities and divergent selection for digestion ability in growing chicks fed a wheat diet. Poultry Science 83:860-867.
LOPEZ, G. and LEESON, S. (2008) Review: energy partitioning in broiler chickens. Canadian, Journal of Animal Science 88:205-212.
LOPEZ, G., DE LANGE, K. and LEESON, S. (2007) Partitioning of retained energy in broilers and birds with intermediate growth rate. Poultry Science 86: 2162-2171.
MACLEOD, M.G. (1994) Dietary energy utilisation: prediction by computer simulation of energy metabolism. British Poultry Science 35:183-184.
MOORE, R.J., STANDLEY, D., KONSAK, B.M., HARING, V.R., HUGHES, R.J., GEIER, M.S. and CROWLEY, T.M. (2011) Correlations between variable broiler performance and gene expression and microflora in the gut. Australian Poultry Science Symposium 22: 9-16.
MORAN, E.T. (1982) Starch digestion in the fowl. Poultry Science 61:1257-1267. NEHRING, K. and HAENLEIN, G.F.W. (1973) Feed evaluation and ration calculationbased on net energy. Journal of Animal Science 36:949-964.
NOBLET, J., FORTUNE, H., SHI, X.S.A. and DUBOIS, S. (1994) Prediction of net energy value of feeds for growing pigs. Journal of Animal Science 72:344-354.
NOBLET, J., VAN MILGEN, J. and DUBOIS, S. (2010) Utilisation of metabolisable energy of feeds in pigs and poultry: Interest of net energy systems? Australian Poultry Science Symposium 21:26-43.
PIRGOZLIEV, V., BEDFORD, M.R., ACAMOVIC, T., MARES, P. and ALLYMEHR, M. (2011) The effects of supplementary bacterial phytase on dietary energy and total tract amino acid digestibility when fed to young chickens. British Poultry Science 52:245-254.
PIRZGOLIEV, V. and ROSE, S.P. (1999) Net energy systems for poultry feeds: a quantitative review. World’s Poultry Science Journal 55:23-36.
SCOTT, T.A. and BOLDAJI, F. (1997) Comparison of inert markers [chromic oxide or insoluble ash (Celite)] for determining apparent metabolizable energy of wheator barley-based broiler diets with or without enzymes. Poultry Science 76:594-598.
SCOTT, T.A. and HALL, J.W. (1998) Using acid insoluble ash marker ratios (diet:digesta) to predict digestibility of wheat and barley metabolizable energy and nitrogen retention in broiler chicks. Poultry Science 77:674-679.
SCOTT, T.A., SILVERDIES, F.G., CLASSEN, H.L., SWIFT, M.L. and BEDFORD, M.R. (1999) Prediction of the performance of broiler chicks from apparent metabolisable energy and protein digestibility values obtained using a broiler chick assay. Canadian Journal of Animal Science 79:59-64.
SELL, J.L., HORANI, F. and JOHNSON, R.L. (1976) The “extra caloric” effect of fat in laying hen rations. Foodstuffs 48:29-39.
SIBBALD, I.R. and WOLYNETZ, M.S. (1986) Cpmparison of three methods of excreta collection used in estimation of energy and nitrogen excretion. Poultry Science 65: 78-84.
SIBBALD, I.R. (1976) A bioassay for true metabolisable energy in feedingstuffs. Poultry Science 55:303-308.
SIBBALD, I.R. (1980) Metabolisable energy in poultry nutrition. BioScience 30:736-741. SIBBALD, I.R. (1985) The true metabolizable energy bioassay as a method for estimating bioavailable energy in poultry feedingstuffs. World’s Poultry Science Journal 41:179-187.
VALDES, E.V. and LEESON, S. (1992a) Measurement of metabolizable energy in poultry feeds by an in vitro system. Poultry Science 71:1493-1503.
VALDES, E.V. and LEESON, S. (1992b) Near infrared reflectance analysis as a method to measure metabolizable energy in complete poultry feeds. Poultry Science 71:1179-1187.
VAN DER KLIS, J.D. and FLEDDERUS, J. (2007) Evaluation of raw materials for poultry: what’s up? Proceedings of the 16th European Symposium on Poultry Nutrition. Strasburg, France, pp. 123-130.
VAN DER KLIS, J.D., KWAKERNAAK, C., JANSMAN, A. and BLOK, M. (2010) Energy in poultry diets: Adjusted AME of net energy? Australian Poultry Science Symposium 21:44-49.
VOHRA, P. (1972) Evaluation of metabolizable energy for poultry. World’s Poultry Science Journal 28:204-214.
WEURDING, R.E., ENTING, H. and VERSTEGEN, M.W.A. (2003) The relation between starch digestion rate and amino acid level for broiler chickens. Poultry Science 82:279-284.
WEURDING, R.E., VELDMAN, A., VEEN, W.A.G., VAN, D.E.R., AAR, P.J. and VERSTEGEN, M.W.A. (2001) Starch digestion rate in the small intestine of broiler chickens differs among feedstuffs. Journal of Nutrition 131:2329-2335.
ZHOU, Z., WAN, H.F., LI, Y., CHEN, W., QI, W.L., PENG, P. and PENG, J. (2010) The influence of the amylopectin/amylose ratio in samples of corn on the true metabolizable energy value for ducks. Animal Feed Science and Technology 157(1):99-103.