Water is essential to life. An adequate, safe and accessible supply must be available to all. Improving access to safe drinking-water can result in significant benefits to health. Every effort should be made to achieve a drinking water quality as safe as possible [1].
Many people struggle to obtain access to safe water. A clean and treated water supply to each house may be the norm in Europe and North America, but in developing countries, access to both clean water and sanitation are not the rule, and waterborne infections are common. Two and a half billion people have no access to improved sanitation, and more than 1.5 million children die each year from diarrheal diseases [2]. According to the WHO, the mortality of water associated diseases exceeds 5 million people per year. From these, more that 50% are microbial intestinal infections, with cholera standing out in the first place.
In general terms, the greatest microbial risks are associated with ingestion of water that is contaminated with human or animal feces. Wastewater discharges in fresh waters and costal seawaters are the major source of fecal microorganisms, including pathogens [1-4].
Acute microbial diarrheal diseases are a major public health problem in developing countries. People affected by diarrheal diseases are those with the lowest financial resources and poorest hygienic facilities. Children under five, primarily in Asian and African countries, are the most affected by microbial diseases transmitted through water [5].
Microbial waterborne diseases also affect developed countries. In the USA, it has been estimated that each year 560,000 people suffer from severe waterborne diseases, and 7.1 million suffer from a mild to moderate infections, resulting in estimated 12,000 deaths a year [6]. The most important bacterial diseases transmitted through water are listed in Table 1.
The concept "one serovar-one species", in use for many years, is no longer acceptable. The taxonomy and nomenclature of the genus Salmonella has been subject of debate since Le Minor and Popoff proposed changes in a paper published in 1987. The issue was settled by a decision of the International Committee on the Systematics of Prokaryotes and published in 2005. The current taxonomy of the genus is presented in Table 4. According to the rules of bacterial nomenclature, the names of the serovars are not italicized and the first letter must be a capital [28-30].
Salmonellae pathogenic to humans can cause two types of salmonellosis: (1) typhoid and paratyphoid fever (do not confuse with typhus, a disease caused by a rickettsia); (2) gastroenteritis [28]. Low infective doses (less than 1,000 cells) are sufficient to cause clinical symptoms. Salmonellosis of newborns and infants presents diverse clinical symptoms, from a grave typhoid-like illness with septicemia to a mild or asymptomatic infection. In pediatric wards, the infection is usually transmitted by the hands of staff [29].
The most important bacterial gastrointestinal diseases transmitted through water are cholera, salmonellosis and shigellosis. These diseases are mainly transmitted through water (and food) contaminated with feces of patients. Drinking water can be contaminated with these pathogenic bacteria, and this is an issue of great concern. However, the presence of pathogenic bacteria in water is sporadic and erratic, levels are low, and the isolation and culture of these bacteria is not straightforward. For these reasons, routine water microbiological analysis does not include the detection of pathogenic bacteria. However, safe water demands that water is free from pathogenic bacteria [57].
The conciliation of the two needs was met by the discovery and testing of indicator bacteria. Water contaminated with pathogenic species also has the normal inhabitants of the human intestine. A good bacterial indicator of fecal pollution should fulfill the following criteria: (1) exist in high numbers in the human intestine and feces; (2) not be pathogenic to humans; (3) easily, reliably and cheaply detectable in environmental waters. Additionally, the following requisites should be met if possible: (4) does not multiply outside the enteric environment; (5) in environmental waters, the indicator should exist in greater numbers than eventual pathogenic bacteria; (6) the indicators should have a similar die-off behavior as the pathogens; (7) if human fecal pollution is to be separated from animal pollution, the indicator should not be very common in the intestine of farm and domestic animals [1,4,6,57,58]. The usefulness of indicator bacteria in predicting the presence of pathogens was well illustrated in many studies, namely by Wilkes et al. [59].
7.2. The Composition of Human and Animal Feces
Microbiological analysis of the human feces was important in order to structure and validate the use of fecal indicator bacteria in environmental waters. Bacteria present in feces are naturally derived from the microbiota of the human gastrointestinal tract.
Although bacteria are distributed throughout the human gastrointestinal tract, the major concentration of microbes and metabolic activity can be found in the large intestine. The upper bowel (stomach, duodenum, and jejunum) has a sparse microbiota with up to 105 CFU/ml of contents. From the ileum on, bacterial concentrations gradually increase reaching in the colon 1010 to 1011 CFU/g [60]. It has been estimated that at least 500–1,000 different microbial species exist in the human gastrointestinal microbiota, although on a quantitative basis 10–20 genera usually predominate (Table 6). The total number of microbial genes in the human gastrointestinal tract has been estimated as 2–4 million. This represents an enormous metabolic potential which is far greater than that possessed by the human host [60,64].
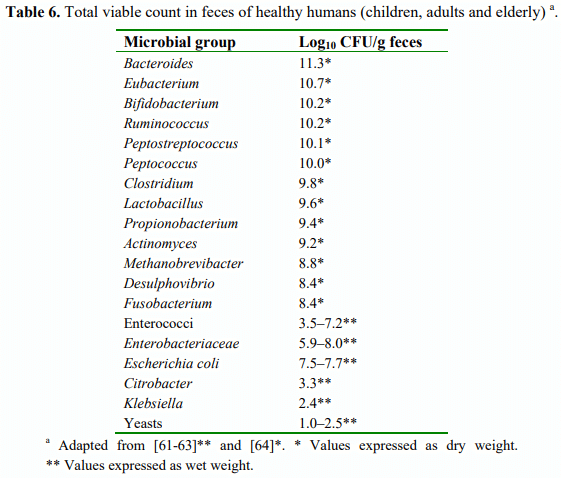
The composition of feces from an individual is stable at genus level, but the species composition can vary markedly from day to day. The relative proportion of intestinal bacterial groups can vary between individuals [60,64].
The microflora of the human gastrointestinal tract is dominated by obligate anaerobes, which are ca. 103 more abundant than facultative anaerobes. The main anaerobic genera are Bacteroides, Eubacterium and Bifidobacteria. These organisms account for ca. 90% of the cultivable human fecal bacteria. Bacteroides (mainly B. thetaiotaomicron and B. vulgatus) are the most abundant organism in the human feces and account for 20–30% of cultivable bacteria. The most abundant facultative anaerobes are Enterococci and Enterobacteriaceae. The main Enterobacteriaceae genera are Escherichia, Citrobacter, Klebsiella, Proteus and Enterobacter. Citrobacter and Klebsiella are present in most individuals although in low numbers. Proteus and Enterobacter are only present in a minority of humans [64].
A variety of molecular techniques have been used to study the microbial composition of the human gastrointestinal tract. Results yielded by these studies have shown that many microbes detected by molecular techniques are not isolable by conventional culture-based methods. The presence of high proportions of bifidobacteria detected by culture-based methods is not supported by the results of molecular-based studies. However, the results of molecular-based approaches support many of the findings derived from culture-based methods: the dominance of the obligate anaerobes over facultative anaerobes; the presence of high counts of Bacteroides, Clostridium and Eubacterium [64].
Anaerobic bacteria such as Bacteroides and Eubacterium are not easily cultured by conventional techniques since require incubation chambers with nitrogen atmosphere. Bifidobacterium and Lactobacillus tolerate some oxygen but are fastidious bacteria growing very slowly in culture media. Therefore, these four genera are not adequate to be used as indicators of fecal pollution (the introduction of molecular techniques may improve the situation). Citrobacter, Klebsiella and Enterobacter are present in low numbers in the human intestine and are widespread in environmental waters, and therefore are also not suitable as indicators of fecal pollution. Clostridium, Streptococcus and Escherichia do not suffer from these drawbacks. Therefore, their suitability as fecal indicators has been tested since several decades.
7.3. Fecal Bacteria in Their Hosts and in the Environment
7.3.1. Bacteroides
The traditional genus Bacteroides included Gram-negative, non-sporeforming, anaerobic pleiomorphic rods. Many species have been transferred to other genera—Mitsuokella, Porphyromonas, Prevotella, Ruminobacter. Bacteroides are the most abundant bacteria in human feces. In animal feces, on the contrary, Bacteroides are present at low numbers. Although anaerobic, Bacteroides are among the most tolerant to oxygen of all anaerobic human gastrointestinal species. B. thetaiotaomicron is one of the most abundant species in the lower regions of the human gastrointestinal tract. Bacteroides have a high pathogenic potential and account for approximately two-thirds of all anaerobes isolated from clinical specimens. The most frequently isolated species has been B. fragilis. The survival of Bacteroides in environmental waters is usually much lower than the survival of coliforms [64,65].
7.3.2. Eubacterium
The traditional genus Eubacterium included anaerobic non-sporeforming Gram-positive rods. Some species have been transferred to other genera—Actinobaculum, Atopobium, Collinsella, Dorea, Eggerthella, Mogibacterium, Pseudoramibacter and Slackia. Cells are not very aerotolerant. Species isolated from the human gastrointestinal tract include: E. barkeri, E. biforme, E. contortum, E. cylindrioides, E. hadrum, E. limosum, E. moniliforme, E. rectal and E. ventricosum [64].
7.3.3. Bifidobacterium
Bifidobacteria are Gram-positive, non-sporeforming, pleiomorphic rods. Bifidobacteria are anaerobic (some species tolerate oxygen in the presence of carbon dioxide) or facultative anaerobic. The optimum growth temperature is 35–39 °C. The genus Bifidobacterium contains ca. 25 species, most of which have been detected in the human gastrointestinal tract [64-66].
Bifidobacteria are present in high numbers in the feces of humans and some animals. Several Bifidobacterium species are specific either for humans or for animals. B. cuniculi and B. magnum have only been found in rabbit fecal samples, B. gallinarum and B. pullorum only in the intestine of chickens and B. suis only in piglet feces. In human feces, the species composition changes with the age of the individual. In the intestine of infants B. breve and B. longum generally predominate. In the adult, B. adolescentis, B. catenulatum, B. pseudocatenulatum and B. longum are the dominant species. In both human and animal feces, bifibobacteria are always much more abundant than coliforms [64-66].
Bifidobacteria have been found in sewage and polluted environmental waters, but appears to be absent from unpolluted or pristine environments such as springs and unpolluted soil. This results from the fact that upon introduction into the environment, bifidobacteria decrease appreciably in numbers, probably due to their stringent growth requirements. Bifidobacteria grow poorly below 30 °C and have rigorous nutrient requirements. Reports on the survival of bifidobacteria in environmental waters indicate that their survival is lower than that of coliforms [64-66].
The presence of bifidobacteria in the environment is therefore considered an indicator of fecal contamination. Since some species are specific for humans and animals, the identification of Bifidobacterium species present in the polluted water could, in principle, provide information on the origin of fecal pollution [64-66].
A study carried out in a highly contaminated stream near Bologna, Italy, revealed that B. adolescentis, B. catenulatum, B. longum, B. pseudocatenulatum and B. thermophilum were the most representative species, whereas B. angulatum, B. animalis subsp. animalis (B. animalis), B. breve, B. choerinum, B. minimum, B. pseudolongum subsp. globosum (B. globosum) and B. subtile occurred only in low numbers [66].
Bifidobacteria are the less studied of all fecal bacteria, due to the technical difficulties in their isolation and cultivation. Other Gram-positive bacteria, such as Streptococcus and Lactobacillus, which may occur in higher numbers than bifidobacteria, can inhibit their growth. Although selective media has been designed for the isolation of bifidobacteria from environmental waters, the outcome is still unsatisfactory, with appreciable numbers of false positives and low recovery percentages [64-66].
7.3.4. Clostridia
The genus Clostridium is one of the largest genera of the prokaryotes containing 168 validly published species. From these, 77 (including C. perfringens) are considered to belong to a united group—Clostridium sensu stricto [64,67,68].
Clostridia are Gram-positive rods, forming endospores. Most of the clostridial species are motile with peritrichous flagellation. Cells are catalase-negative and do not carry out a dissimilatory sulphate reduction. Clostridia usually produce mixtures of organic acids and alcohols from carbohydrates and proteins. Many species are saccharolytic and proteolytic. Some species fix atmospheric dinitrogen [64,67,68].
The genus Clostridium includes psychrophilic, mesophilic, and thermophilic species. The major role of these organisms in nature is in the degradation of organic material to acids, alcohols, CO2, H2, and minerals. Frequently, a butyric acid smell is associated with the proliferation of clostridia. The ability to form spores that resist dryness, heat, and aerobic conditions makes the clostridia ubiquitous [64,67,68].
Most species are obligate anaerobic, although tolerance to oxygen occurs. Oxygen sensitivity restricts the habitat of the clostridia to anaerobic areas or areas with low oxygen tensions. Growing and dividing clostridia will, therefore, not be found in air saturated surface layers of lakes and rivers or on the surface of organic material and soil. Clostridial spores, however, are present with high probability in these environments, and will germinate when oxygen is exhausted and when appropriate nutrients are present [64,67,68].
C. perfringens ferment lactose, sucrose and inositol with the production of gas, produce a stormy clot fermentation with milk, reduce nitrate, hydrolyze gelatin and produce lecithinase and acid phosphatase. The species is divided into five types, A to E, on the basis of production of major lethal toxins [68,69].
C. perfringens appears to be a universal component of the human and animal intestine, since has been isolated from the intestinal contents of every animal that has been studied. Humans carry C. perfringens as part of the normal endogenous flora. The main site of carriage is the distal gastrointestinal tract. The principal habitats of type A are the soil and the intestines of humans, animals, and birds. Types B, C, D, and E appears to be obligate parasites of animals and occasionally are found in humans [68,69].
Clostridium perfringens is the most frequently isolated Clostridium in clinical microbiology laboratories, although it seldom causes serious infections. C. perfringens is isolated from infections in humans and the organism most commonly found in gas gangrene in humans. C. perfringens is most commonly isolated from infections derived from the colonic flora, namely peritonitis or abdominal abscess [68,69].
This organism is a common cause of food poisoning due to the formation of the enterotoxin in the intestine. C. perfringens food poisoning is seldom fatal, being marked by diarrhea and nausea, with no vomiting and no fever [68,69].
Sources yielding C. perfringens include soil and marine sediment samples worldwide, clothing, raw milk, cheese, semi-preserved meat products, and venison. Like E. coli, C. perfringens does not multiply in most water environments and is a highly specific indicator of fecal pollution. Berzirtzoglou et al. [70] reported a comparative study on the occurrence of vegetative cells and spores of Clostridium perfringens in a polluted station of the lake Pamvotis, in rural North-West Greece. The numbers of C. perfringens varied according to the water depth. Sporulated forms were found in all sampling sites with the exception of the surface sampling.
7.3.5. Lactobacillus
Lactobacilli are non-sporeforming Gram-positive long rods. There are more than thirty species in the genus. Most are microaerophillic, although some are obligate anaerobes. Cells are catalase-negative and obtain their energy by the fermentation of sugars, producing a variety of acids, alcohol and carbon dioxide. Lactobacilli have complex nutritional requirements and in agarized media may need the supplementation with aminoacids, peptides, fatty-acid esters, salts, nucleic acid derivatives and vitamins. Lactobacilli very rarely cause infections in humans [64].
7.3.6. Enterococci
Enterococci are Gram-positive, non-sporeforming, catalase-negative ovoid cells. Cells occur singly, in pairs or short chains. Optimal growth for most species is 35–37 °C. Some will grow at 42 –45 °C and at 10 °C. Growth requires complex nutrient s but is usually abundant on commonly used bacteriological media. Cells are resistant to 40% bile, 0.4% azide, 6.5% sodium chloride, have β-glucosidase and hydrolyze esculin. The enterococci are facultative anaerobic but prefer anaerobic conditions [64,71].
The genus was separated from Streptococcus in the 1980s. Enterococci form relatively distinct groups. Members of such groups exhibit similar phenotypic characteristics and species delimitation can be difficult. The E. faecalis group contains, among others, E. faecalis. The E. avium group contains, among others, E. avium. The E. faecium group contains, among others, E. faecium, E. durans and E. hirae. The E. gallinarum group contains, among others, E. gallinarum [64,71].
Most species are part of the intestinal flora of mammals, reptiles, birds, and other animals. In the human digestive tract, E. faecalis is the prevailing species, although in particular situations, E. faecium may predominate. In poultry, E. cecorum, E. durans, E. faecalis, E. faecium and E. hirae and dominate the intestinal flora [64,71].
Enterococci have been increasingly isolated from a variety of nosocomial and other infections, mainly from the urinary tract and wound infections, bacteremias, and endocarditis [64,71].
Although enterococci are considered only a temporary part of the microflora of plants, in optimal conditions, cells can proliferate on their surfaces. E. casseliflavus, E. faecalis, E. faecium, E. hirae, E. mundtii and E. sulfureus have been isolated from plants. They are generally isolated more often from flowers than from buds or leaves [64,71].
Enterococci are naturally present in many kinds of foods, especially those of animal origin such as milk and milk products, meat and fermented sausages. Enterococci are usually considered secondary contaminants of food, although they often play a positive role in ripening and aroma development of some types of cheeses [64,71]. Although soil is not a natural habitat for enterococci, cells can be found in this habitat due to the transport by rain [64,71].
Environmental waters are not a natural habitat for enterococci and their presence in this milieu is considered the result of fecal pollution. The most common species found in environmental waters are
E. durans, E. faecalis, E. faecium and E. hirae, and less commonly, E. avium, E. cecorum, E. columbae and E. gallinarum. However, pristine waters in Finland have been reported to contain E. casseliflavus [64,71].
In environmental samples (compost, sewage effluent, harbor sediments, brackish water and swimming pool water), Pinto et al. [72] reported the isolation of E. casseliflavus, E. durans, E. faecalis, E. faecium, E. gallinarum and E. hirae. E. durans, E. faecium and E. hirae were isolated from all sources except from harbor sediments. E. raffinosus was only isolated from compost and swimming pool water. E. faecalis and E. faecium accounted for the vast majority of enterococcal strains.
7.3.7. Escherichia
Escherichia, a member of Enterobacteriaceae, are oxidase-negative catalase-positive straight rods that ferment lactose. Cells are positive in the Methyl-Red test, but negative in the Voges-Proskauer assay. Cells do not use citrate, do not produce H2S or lipase, and do not hydrolyze urea [73]. E. coli is a natural and essential part of the bacterial flora in the gut of humans and animals. Most E. coli strains are nonpathogenic and reside harmlessly in the colon. However, certain serotypes do play a role in intestinal and extra-intestinal diseases, such as urinary tract infections [43]. In a study of the enteric bacteria present in the feces of Australian mammals, Gordon and FitzGibbon [74] reported that E. coli was the commonest species, being isolated from nearly half of the species studied.
7.3.8. Citrobacter
Citrobacter, a member of Enterobacteriaceae, are motile straight rods. Cells are oxidase-negative, catalase-positive and positive in the Methyl-Red test. Cells use citrate, are negative in the Voges-Proskauer test and do not decarboxylate lysine [73].
In a study of the enteric bacteria present in the feces of Australian mammals, Gordon and FitzGibbon [74] reported the isolation of C. amalonaticus, C. freundii and C. koseri (C. diversus). Citrobacter species can be isolated from different clinical sites. In particular, C. freundii is an intestinal inhabitant of humans that may sometimes have—or acquire—the ability to produce an enterotoxin and thus become an intestinal pathogen. Citrobacter is reported to occur in environments such as water, sewage, soil and food [75,76].
7.3.9. Klebsiella and Raoultella
Klebsiella and Raoultella are Enterobacteriaceae, oxidase-negative catalase-positive non-motile straight rods, surrounded by a capsule. Cells decarboxylate lysine, but are ornithine and arginine dihydrolase negative. Cells grow on KCN, do not produce H2S and ferment most carbohydrates [73].
In humans, K. pneumoniae is present as commensal in the nasopharynx and in the intestinal tract. Klebsiella spp. can cause human diseases, ranging from asymptomatic colonization of the intestinal, urinary, or respiratory tract to fatal septicemia. Klebsiella are mostly considered nosocomial pathogens. K. pneumoniae and Enterobacter aerogenes (K. mobilis) are most frequently involved, although K. oxytoca and R. planticola, and rarely R. terrigena, can be found. In the hospital, the principal reservoir of K. pneumoniae is the gastrointestinal tract of patients. The principal vectors are the hands of personnel [77,78]. In a study of the enteric bacteria present in the feces of Australian mammals, Gordon and FitzGibbon [74] reported the isolation of K. pneumoniae and K. oxytoca.
Klebsiellae are ubitiquous in the environment. They have been found in a variety of environmental situations, such as soil, vegetation, or water, and they influence many biochemical and geochemical processes. They have been recovered from aquatic environments receiving industrial wastewaters, plant products, fresh vegetables, food with a high content of sugars and acids, frozen orange juice concentrate, sugarcane wastes, living trees, and plants and plant byproducts. They are commonly associated with wood, sawdust, and waters receiving industrial effluents from pulp and paper mills and textile finishing plants (see below). Klebsiella have been isolated from the root surfaces of various plants. K. pneumoniae, K. oxytoca, and R. planticola are all capable of fixing dinitrogen [77,78].
7.3.10. Enterobacter
Enterobacter a member of Enterobacteriaceae, are motile straight rods. Cells are positive in the Voges-Proskauer test VP and in Simmons citrate agar. Cells do not decarboxylate lysine, but are ornithine positive. Malonate is usually utilized and gelatin is slowly liquefied. Cells do not produce H2S, deoxyribonuclease and lipase [73].
In a study of the enteric bacteria present in the feces of Australian mammals, Gordon and FitzGibbon [74] reported the isolation of Enterobacter cloacae subsp. cloacae (E. cloacae), E. cancerogenus (E. taylorae) and E. aerogenes (Klebsiella mobilis).
Before the widespread use of antibiotics, Enterobacter species were rarely found as pathogens, but these organisms are now increasingly encountered, causing nosocomial infections such as urinary tract infections and bacteremia. In addition, they occasionally cause community-acquired infections [79,80]. In the USA, the Surveillance and Control of Pathogens of Epidemiological Importance project analyzed 24,179 nosocomial bloodstream infections, from 1995–2002. Enterobacter species were the second most common gram-negative organism, behind Pseudomonas aeruginosa. Both bacteria were reported to each represent 4.7% of bloodstream infections in intensive care units. Enterobacter species represented 3.1% of bloodstream infections in non-intensive care units. Of nearly 75,000 gram-negative organisms collected from intensive care units‘ patients in the USA, between 1993 and 2004, Enterobacter species comprised 13.5% of the isolates. Multidrug resistance increased over time, especially in infections caused by E. cloacae [81].
In the USA, the National Healthcare Safety Network reported a study on healthcare-associated infections between 2006 and 2007. They found Enterobacter species to be the eighth most common cause of healthcare-associated infections (5% of all infections) and the fourth most common gram-negative cause of these infections [82].
Enterobacter cloacae subsp. cloacae (E. cloacae) occurs in the intestinal tracts of humans and animals, in hospital environments, the skin, in water, sewage, soil, meat. Nitrogen-fixing strains have been isolated from the roots of rice plants. E. amnigenus has been mostly isolated from water, but some strains were isolated from clinical specimens from the respiratory tract, wounds and feces.
E. asburiae strains were isolated from clinical specimens, mostly urine, respiratory tract, feces, wounds, and blood [79,80].
7.4. Origin of the Use of Fecal Indicator Bacteria
Historically, the design and use of indicators of fecal pollution comes from the end of the 19th to beginning of the 20th century. In 1880, von Fritsch described Klebsiella pneumoniae and K. pneumoniae subsp. rhinoscleromatis (Klebsiella rhinoscleromatis) as micro-organisms characteristically found in human feces [83]. In 1885, Escherich described several microorganisms in the feces of newborn and suckling babies. This included a motile, rod-shaped microorganism that caused milk to clot, which was named "Bacterium coli commune". He observed that within a few weeks after birth, this bacterium became the dominant organism in the infant colon [6]. Also in 1885, Percy and Grace Frankland started the first routine bacteriological examination of water in London, using Robert Koch‘s solid gelatin media to count bacteria [83]. In 1891, Percy and Grace Frankland came up with the concept that organisms characteristic of sewage must be identified to provide evidence of potentially dangerous pollution [83]. In 1892, Schardinger proposed that since "Bacterium coli" was a characteristic component of the fecal flora, its presence in water could be taken as an indication of the presence of fecal pollution and therefore of the potential presence of enteric pathogens [6]. Soon after the description of "Bacterium coli", other bacteria were isolated from stools and water—Klebsiella in 1882 and Enterobacter in 1890 [6]. By 1893, the "Wurtz method" of enumerating "Bacterium coli", by direct plating water samples on litmus lactose agar, was being used by sanitary bacteriologists. This was based on the concept of acid and gas production (detected by the Durham tube) from lactose as a diagnostic feature [6]. In 1905, MacConkey described his now famous MacConkey‘s broth, which was diagnostic for lactose-fermenting bacteria tolerant of bile salts. Coliforms were already considered to be a heterogeneous group of organisms, many of which were not of fecal origin. The origins of the critical observation that "Bacterium coli" was largely fecal in origin while other coliforms were not, could be claimed by Winslow and Walker in 1907 [83].
Various classification schemes for coliforms have emerged. The earliest were those of MacConkey in 1909, which recognized 128 different coliform types, while Bergey and Deehan in 1908, identified 256. By the early 1920s, differentiation of coliforms had come to a series of correlations that suggested that indole production, gelatin liquefaction, sucrose fermentation and the Voges–Proskauer reaction were among the more important tests for determining fecal contamination. These developments culminated in the IMViC (Indole, Methyl Red, Voges–Proskauer and Citrate) tests for the differentiation of so-called fecal coliforms, soil coliforms and intermediates [83].
7.5. Fecal Indicator Bacteria
7.5.1. Coliforms
Total coliforms are Gram-negative, oxidase-negative, non-sporeforming rods, that ferment lactose with gas production at 35–37 °C, after 48h, in a medium with b ile salts and detergents [1,4,6,57,84]. When the test of coliforms is carried out with environmental waters, several species of the four Enterobacteriaceae genera Escherichia, Klebsiella, Enterobacter and Citrobacter give positive results and therefore are coliforms according to this definition. However, the environmental significance of these four genera is very disparate as discussed in the present text. Therefore, total coliform counts are not necessarily a measure of fecal pollution and indeed can have no relation with this cause [1,4,6,84].
Fecal coliforms (or thermotolerant coliforms) are traditionally defined as coliforms that ferment lactose at 44.5 °C in a medium with bile salts [1,4,57,84]. The range of species detected by the experimental procedure is much lower than that of total coliforms. With environmental polluted waters, only E. coli, and K. oxytoca and K. pneumoniae gave positive results in the test [85].
Traditional tests for total and fecal coliforms are carried out either by the multiple-tube fermentation technique or by filtration through membrane. The multiple-tube fermentation technique is used for medium or highly contaminated waters, and the filtration through membrane for low or very low contaminated waters. Filtration through membrane is a very sensitive technique since can detect one (culturable) cell in 500 or even 1,000 mL of water. However, both methods take several days to complete and do not detect viable but non-culturable bacteria [3,57,86]. These limitations stimulate the discovery of alternative methods, faster and, if possible, less prone to false negative results such as those caused by the viable but non-culturable bacteria.
The detection of β-D-galactosidase activity (at 37 °C) is usually a good marker for total coliform s in environmental waters, since most of these bacteria display this enzymatic activity [1,3,57,87-91]. Most Escherichia, Citrobacter, Enterobacter, Klebsiella and Raoultella strains have galactosidase. Hafnia, Serratia and Yersinia also possess this enzymatic activity. Most Proteus, Salmonella and Edwardsiella strains do not display β-galactosidase [92-95]. Ca. 10% of the coliform strains isolated from the environment do not have an active formic hydrogenolyase (cleaves formate with the formation of CO2) and therefore do not produce gas being undetected by the traditional techniques but are detected by the assay of β-galactosidase activity [57,96,97].
β-galactosidase cleaves lactose in glucose and galactose, and can be detected by using colored or fluorescent markers that change color after enzyme action, such as XGAL (5-bromo-4-chloro-3-indol- β-galactopyranoside) and ONPG (O-nitrophenyl-β-D-galactopyranoside) or MUGAL (4-methylumbelliferyl-β-D-galactopyranoside), respectively [57,96,97].
In environmental waters, the presence of Aeromonas or Vibrio cholerae can be a source of false positives in the β-D-galactosidase assay, since these bacteria have galactosidase, but are not coliforms [93,95,96,98]. Additionally, in particular environments, such as estuaries, β-galactosidase activity can overestimate total coliform count due to UV-stimulated enzymatic activity in certain bacteria such as E. coli [86].
The detection of β-D-glucuronidase activity (at 44.5 °C) is, generally, a good marker for fecal coliforms in environmental polluted waters and very specific for E. coli [13,85,87-91,97,99-101]. In Gram-negative bacteria, this enzymatic activity if found in most E. coli strains and in some Salmonella and Shigella strains [92-95,97,102]. Aeromonas, Citrobacter, Enterobacter, non-coli Escherichia, Hafnia, Klebsiella, Proteus, Serratia, Vibrio, Yersinia, and most Salmonella strains do not display β-glucuronidase activity [93-95,101,102].
β-D-glucuronidase activity can be detected by using colored or fluorescent markers that change color after enzyme action, such as XGLUC (5-bromo-4-chloro-3-indoxyl-β-D-glucuronide), IBDG (indoxil-β-glucuronide), and MUGLU (4-methylumbelliferyl-β-D-glucuronide), respectively [57,97,100].
The presence of this enzyme in some strains of Bacteroides, Flavobacterium, Staphylococcus, Streptococcus, in anaerobic corynebacteria and Clostridium, has also been reported [93,95-97,102]. β-D-glucuronidase activity in fecal bacteria other then E. coli (Bacteroides, bifidobacteria, clostridia, enterococci and Lactobacillus) is very limited [61]. Although all these glucuronidase positive bacteria could lead to false positive detections in the fecal coliform test, experimental results for environmental polluted waters indicate a significant correlation between fecal coliform detection using conventional techniques and the glucuronidase assay, suggesting that false positives are not significant [85,96].
The detection of total coliforms and fecal coliforms by enzymatic methods are much less time consuming than traditional techniques. With fluorescent markers and the use of a spectrofluorimeter the detection of coliforms can be performed in minutes [57,101]. However, in very low contaminated waters, enzymatic methods might not be able to detect coliform cells. Moreover, on-line monitoring of glucuronidase activity is currently too insensitive to replace culture based detection of E. coli. Nevertheless, on-line enzymatic methods can be a valuable complementary tool for high temporal resolution monitoring. More research is needed in order to enhance sensitive and lower detection limits of available on-line glucuronidase techniques.
The seminal work of Leclerc et al. [103] clarified the diversified roles that coliforms have in the environment and the real meanings of the tests on total coliforms and fecal coliforms. It was shown that Enterobacteriaceae encompass three groups of bacteria with very different roles in the environment. Group I harbored only E. coli. Since this species usually do not survive for long periods outside this environment (but see topic 10), it was considered a good and reliable indicator of fecal pollution (both animal and human). Group II, the "ubiquitary" group, encompassed several species of Klebsiella (K. pneumoniae and K. oxytoca), Enterobacter (Enterobacter cloacae subsp. cloacae, E. aerogenes) and Citrobacter (C. amalonaticus, C. koseri and C. freundii). These bacteria live in the animal and human gut, but also in the environment, and are easily isolated from the soil, polluted water and plants. Their presence in polluted waters does not necessarily indicate fecal pollution. Finally, Group III was composed of Raoultella planticola, R. terrigena, Enterobacter amnigenus and Kluyvera intermedia (Enterobacter intermedius), Serratia fonticola, and the genera Budvicia, Buttiauxella, Leclercia, Rahnella, Yersinia, and most species of Erwinia and Pantoea. These bacteria live in fresh waters, plants and small animals. They grow at 4 °C, but not at 41 °C. They are not indicators of fecal pollution, although can be detected in the total coliform test. Leclerc et al. concluded that: (1) in the enterobacteria, E. coli is the only true and reliable indicator of fecal pollution in environmental waters; (2) the traditional total coliform test should be abandoned, since it can detect bacteria that have no connection with fecal pollution; (3) the detection of fecal coliforms must be carried out at 44.5 °C, and positive results confirmed by identification to species levels in order to exclude false positives such as K. pneumoniae.
7.5.2. Streptococci and Enterococci
Fecal streptococci also belong to the traditional indicators of fecal pollution. Fecal streptococci are Gram-positive, catalase-negative, non-sporeforming cocci that grow at 35 °C in a medium containing bile salts and sodium azide. Cells hydrolyze esculin [1,4,57]. Azide is a strong inhibitor of the respiratory chain. Since streptococci are one of the very few bacteria that have no respiratory chain, the test is very specific for this group, and false positives are rarely found [104,105].
Fecal enterococci (E. faecalis, E. faecium, E. avium and E. gallinarum) are fecal streptococci that grow in the presence of 6.5% NaCl at 45 °C. Selective media use these particular characteristics in order to separate enterococci from the other streptococci [104,105].
Several studies [104,106] have reported on the microbiological composition of human and animal (cattle, chicken, deer, dog, fowl, goose, and swine) feces. E. faecalis and E. faecium were present in human and animal feces. However, whereas human feces almost have only these two enterococci, in the animals others species co-occur, like E. avium, E. cecorum, E. durans, E. gallinarum and E. hirae. It was concluded that in urban areas where contamination with dog and chicken feces is not likely, the best marker for human fecal pollution was E. faecalis.
The intestinal enterococci group has been used as an index of fecal pollution. In human feces, the numbers of intestinal enterococci are generally about an order of magnitude lower than those of E. coli (Table 6). Most species do not grow in environmental waters. In this milieu, fecal enterococci are able to survive longer, are more resistant to drying and chlorination, than E. coli [1,84].
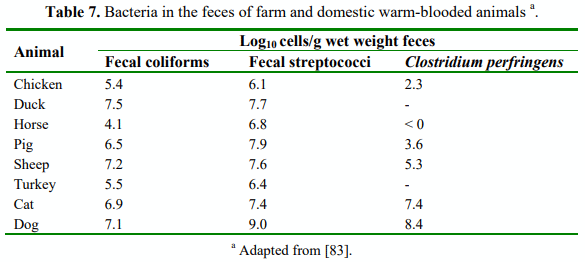
However, caution should be taken with interpreting the results obtained by the enterococci procedure in water analysis. Enterococci and other group D-streptococci are present in many foods, especially those of animal origin. The isolation of E. faecalis and E. faecium was used to indicate fecal contamination of food. However, enterococci are now also considered as normal parts of the food microflora and not only as indicators for poor hygiene [104]. In addition, agricultural soils and crops with added with manure also harbor enterococci [105].
7.5.3. The use of ratios between indicator counts
The ratio of counts of fecal coliforms to fecal streptococci has been proposed as a means to differentiating between contamination from human and animal sources. Ratios greater than 4 have been suggested to indicate a human source whereas ratios less than 0.7 suggest an animal source. This results from the fact that streptococcal concentrations in human feces are generally less than coliforms (Table 6). In contrast, in animal feces fecal streptococci generally outnumber fecal coliforms (Table 7). In urban sewage, fecal streptococci tend to be present in concentrations 10–100 times less than fecal coliforms [65].
Geldreich [107] summarized the information available on the fecal coliforms to fecal streptococci ratios in the feces of warm-blooded animals, and reported the following values: human feces, 4.3; cattle, sheep, and poultry, from 0.104 to 0.421; and wild animals (including rabbits, field mice, chipmunks, and birds), 0.0008 to 0.043. Fecal coliforms to fecal streptococci ratios for the feces of wild animals appear to be at least 10-fold lower than those of domestic livestock.
Doran and Linn [108] reported a study of the runoff from a cow-calf pasture in eastern Nebraska (USA), monitored during a three-year period. It was concluded that the fecal coliforms to fecal streptococci ratio in pasture runoff was useful in identifying the relative contributions of cattle and wildlife and in evaluating the effects of cattle management and distribution on runoff water quality.
Ratios below 0.05 were indicative of wildlife sources and ratios above 0.1 were characteristic of grazing cattle. Fecal coliforms to fecal streptococci ratios of diluted cattle waste in excess of 1 were interpreted as the result of differential aftergrowth and die-off between fecal coliforms and fecal streptococci. Ratios between 0.7 and 4.0 may indicate situations where cattle are localized close to sampling or outflow points.
However, the interpretation of this ratio should be cautious. It has been observed a shift in the ratio with time and distance from the fecal pollution source. This resulted from the fact that both in surface and groundwaters, fecal streptococci are more persistent than fecal coliforms. Therefore increasing the distance from the pollution point and with passing time, the ratio tends to decrease without a change in the nature of the pollution source [65]. For these reasons, this ratio has been considered by some authors as too unreliable to be useful in characterizing pollution sources [65,84].
The ratio of fecal enterococci to fecal streptococci differs among vertebrate species. Humans have a predominance of enterococci, whereas animals contain appreciable amounts of streptococci. However, since enterococci are also present in animals and are more persistent in the environment than other fecal streptococci, the identification of the enterococci and streptococci species present in polluted waters, and the concomitant calculation of this ratio is generally considered unreliable as an indicator of the source of fecal pollution [65].
7.5.4. Limitations of coliform and enterococcus counts as indicator of fecal pollution
An extreme case of uselessness of the determination of total and fecal coliforms and enterococci in the assessment of fecal pollution has been demonstrated by several authors studying the microbiology of pulp and paper mill effluents.
Caplenas and Kanarek [109] reported a study of pulp and paper mills located in Wisconsin (USA). Fresh water supplies, re-cycled water within mills, treated effluent wastewater and waters receiving effluent wastes downstream, were assessed for the presence of fecal coliforms and Klebsiella. Wastewaters prior to treatment contained fecal coliforms and Klebsiella. Up to 84% of the fecal coliforms (detected by the standard test procedure) were indeed Klebsiella. In treated effluent wastewaters this value reached 90%. Treatment of the wastewater lowered the concentration of ?true? fecal bacterial contamination, but since Klebsiella grew rapidly in the wastewaters, fecal coliform counts were high, although no true fecal contamination was involved. The source of Klebsiella was traced to the early pulping stages in the mills. Klebsiella maintains a wood, bark or soil reservoir. It was concluded that: (1) Klebsiella are ubiquitous in the pulp and paper mill industry processing stages; (2) the standard procedure for fecal coliform estimation is useless to assess the microbiological quality of the effluents of these industries; (3) The assay of E. coli should replace the fecal coliform detection procedure.
Gauthier et al. [110] and Gauthier and Archibald [58] reported two studies of seven pulp and paper mills in Ontario and Quebec, Canada. Total and fecal coliforms and enterococci were detected in nearly all the biotreatment, biosolids (sludges), and in-mill water system samples. In the mill samples, the majority of the fecal coliforms (detected by the standard test procedure) were K. pneumoniae, Raoultella terrigena and Raoultella planticola, with E. coli in minority. E. faecalis and E. faecium were detected in relatively large numbers in most samples from all of the seven mills examined. Other coliforms such as Enterobacter spp. and Citrobacter freundii were occasionally recovered from total and fecal coliform tubes. Biofilms established in the piping, tanks, and machinery where thermal and pH conditions permit were the most likely source of these bacteria. Analyses using two independent Salmonella detection/enumeration methods showed no detectable Salmonella cells in the sludges and final effluents of the five mills tested. It was concluded that for these particular systems, the determination of total and fecal coliforms and enterococci is useless and have no relationship with real fecal pollution. These studies also demonstrated the importance of checking the identities of bacteria causing the positive results in the tests. Both Escherichia and Klebsiella can give positive results in the fecal coliform test, but their ecological meaning is opposite.
Another important case of failure of the use of coliforms to detect fecal pollution was the 1993
Cryptosporidium outbreak in Milwaukee (USA).
Cryptosporidium parvum, a protozoan parasite that causes gastrointestinal illness, is transmitted by ingestion of oocysts excreted in human or animal feces. Typical modes of transmission include person to person, animal to person, by exposure to contaminated food or water [111,112].
From 1990 to 2000, at least 10 cryptosporidiosis outbreaks associated with contaminated drinking water were reported in the USA. In 1993, an estimated 403,000 residents of the greater Milwaukee area (Wisconsin, population, ca. 1.61 million) became ill when an ineffective filtration process led to the inadequate removal of Cryptosporidium oocysts in one of two municipal water-treatment plants [111,112]. It was the largest waterborne disease outbreak in documented USA history. Over the span of approximately two weeks, people became ill with stomach cramps, fever, diarrhea and dehydration caused by the pathogen. More than half the people who received residential drinking water from the southern water-treatment plant became ill, which was twice the rate of illness among people whose residential drinking water came mainly from the northern water-treatment plant. Over 54 deaths were attributed to this outbreak, mostly among the elderly and immunocompromised people, such as AIDS patients [111,112].
Standard microbiological water analysis was ineffective in detecting this parasite. Indeed, throughout the period from February to April, samples of treated water from both plants were negative for coliforms. The origin of the contamination was determined as water from Lake Michigan. No specific source of the Cryptosporidium was ever identified but runoff from abnormally heavy spring rains most likely carried the parasite to the lake [111,112].
7.5.5. Clostridium perfringens
Sulphite-reducing clostridia, namely Clostridium perfringens, are spore-forming Gram-positive, non-motile, anaerobic, sulfite-reducing rods. C. perfringens is present in higher numbers in the feces of some animals, such as dogs, than in the feces of humans and less often in the feces of many other warm-blooded animals. The numbers excreted in feces are normally substantially lower than those of E. coli.
Clostridium spores are exceptionally resistant to unfavorable conditions in water environments, including UV irradiation, temperature and pH extremes, and disinfection processes, such as chlorination. Although clostridia probably do not growth in surface waters, the high resistance of their spores makes their presence ubiquitous in environmental waters [1,4,84,113].
The presence of chlorine in water rapidly inactivates indicator bacteria such as E. coli and coliforms, but it leaves the most resistant pathogens almost unaffected for several hours. This creates a false sense of security by providing negative coliform and negative E. coli results to authorities responsible for water testing. Giardia cysts, Crystosporidium oocysts, and human enteric viruses all have higher resistance to disinfectants and constitute a major public health risk if distribution system integrity is breached. C. perfringens spores are less affected by the residual concentrations of chlorine. Testing for the spores of this bacterium can probably provide an added margin of safety in the evaluation of treatment [114].
7.5.6. Correlations between parameters used to assess fecal pollution
In environmental waters, several studies have reported significant correlations between indicators of fecal pollution and between indicators and pathogenic gastrointestinal bacteria.
Charriere et al. [115] reported a study of deep aquifer waters (raw waters and piped chlorinated waters) in Normandy, France. In heavily contaminated raw waters and in slightly contaminated treated waters, fecal coliforms and enterococci were correlated.
Martins et al. [116] reported a study of 60 public outdoor swimming pools in Sao Paulo city, Brazil. Total coliforms, fecal coliforms and fecal streptococci levels increased with number of bathers and water temperature, and decreased with chlorine levels. All these indicators were significantly correlated with each other.
Ferguson et al. [117] reported a study carried out in Georges River, in the Sydney region, Australia. In the water column, concentrations of fecal coliforms, fecal streptococci, C. perfringens spores were all positively correlated with each other. Isolation of Salmonella spp. were most frequent during rainfall and sewage overflow events. In the water column, 55% of samples contained Salmonella when fecal coliform densities exceeded 2,000 CFU/100 mL.
Medema et al. [118] reported a study of seven different fresh water sites normally used for triathlon competitions. Sites were small rivers, channels, lakes and harbors, and were influenced by sewage effluents and agricultural run-off. When data from all triathlons were pooled, geometric mean densities of fecal coliforms and E. coli, of E. coli and fecal enterococci and of fecal coliforms and fecal enterococci, were significantly correlated.
Polo et al. [119] reported a study of water samples obtained from 213 beaches, eight rivers and 14 freshwaters in north-eastern Spain. In freshwaters and heavily contaminated seawaters, Salmonella and fecal coliforms were correlated, while in less contaminated seawaters, the highest correlation was with between Salmonella and fecal streptococci.
Byamukama et al. [99] reported a study of the microbiology of Nakivubo channel, Uganda. This channel receives raw sewage from slums, industrial effluents, and discharges from a sewage treatment plant and from a complex of slaughterhouses. Water from eight sampling sites was assessed for the presence of total and fecal coliforms, E. coli and sulphite-reducing clostridia. All microbiological parameters were significantly correlated.
Noble et al. [120] reported a comparative determination of total coliforms, fecal coliforms and enterococci in 108 sites along the southern California coastline, USA. Results by traditional and enzymatic methods and from all three parameters were correlated.
Harwood et al. [121] reported a study on indicator and pathogenic microorganisms carried out in six wastewater reclamation facilities in the USA. Data from disinfected effluent (reclaimed water) samples were analyzed separately (by facility) and as a pooled data set (all facilities). Significant correlations between indicator organism concentrations were observed in the pooled data sets, namely for total and fecal coliforms.
Cabral and Marques [85] reported a microbiological study of a polluted river (Febros) in the Great Oporto area, northwest Portugal. Total and fecal coliforms, fecal streptococci and enterococci were all significantly correlated with each other.
Touron et al. [122] reported a study carried out in the Seine estuary, France. Water was sampled at nine stations (along an upstream/downstream transect of 156 km), during nine years, for fecal coliforms, E. coli, enterococci and Clostridium perfringens spores. At the upstream part of the estuary (at Poses), Salmonella and fecal coliforms, and E. coli and enterococci counts were correlated. At the mouth of the estuary (at Honfleur), significant correlation was found for Salmonella and enterococci counts. No significant correlation between concentrations of any combination of indicator organism and pathogen was observed.
Wilkes et al. [59] reported a comparative study on the presence and concentration of several pathogenic and indicator bacteria in the surface water of a Canadian river. Surface water was collected within the South Nation River basin in eastern Ontario, from the river proper, and from several lower stream order tributaries. Using data aggregated during the entire multi-year study, significant correlations were found among all indicator bacteria - total and fecal coliforms, E. coli, Enterococcus, and C. perfringens.
However, in others studies no correlation was found between the different fecal indicator bacteria. Garrido-Pérez et al. [123] reported a study of the bathing seawater quality in 18 Spanish beaches near the Strait of Gibraltar. Sample locations were selected as a single point located in the area of highest bather density of each beach. No significant correlation was found between fecal coliforms and Clostridium perfringens counts in the bathing seawater.
8. Fecal Indicator Chemical Compounds
Several chemical substances have been used as markers of fecal pollution in environmental waters. Caffeine is present in several beverages and in many pharmaceutical products. It is excreted in the urine of individuals who have ingested the substance. The main source of caffeine in domestic wastewaters is excretion following consumption of coffee, tea, soft drinks, or medication. Levels of caffeine in domestic wastewater have been measured to be between 20 and 300 µg/L. Levels in receiving waters are much lower due to significant dilution. Due to its high solubility, low octanol-water partition coefficient, insignificant volatility and clear anthropogenic origin, the presence of caffeine in environmental waters can be a good marker for human fecal pollution [124-129].
However, relationships between fecal indicator bacteria and caffeine are variable. Wu et al. [129] reported a study carried out in the Rochor Canal and Marina Bay, Singapore. In Rochor Canal, the highest concentration of caffeine (1.35 ng/mL) was found at downstream, and the lowest (0.68 and 0.37 ng/mL) were determined at middle and upstream points. At Marina Bay, the concentration of caffeine was in the range of 0.41–0.96 ng/mL. Fecal coliform concentrations were very high, exceeding 5,000 CFU/100 mL. Caffeine and fecal coliform concentrations were significantly correlated in Rochor Canal samples, but no significant correlation was observed in Martina Bay water samples.
The use of caffeine as marker of fecal pollution has additional important limitations. Caffeine is often present in the urban environment from numerous plant species debris as well as from human "dumping" of coffee wastes. In addition, the current analytical methods used are relatively complex and expensive [124].
Coprostanol (5β-cholestan-3β-ol) is a fecal stanol that is formed by indigenous bacteria present in the gut of humans and higher animals, during catabolism of cholesterol. It is the main stanol present in human feces (24 to 89% of total steroids) and in domestic wastewater. Based on these facts, it has been proposed as a chemical indicator of human fecal pollution. Feces from pigs and cats also contain coprostanol, but at much lower levels. Additional fecal stanols, such as 24-ethylcoprostanol, were found to be predominant in herbivores, such as cows, horses, and sheep, suggesting potential use of this chemical as an indicator of fecal pollution from these sources. Reported half-lives of coprostanol in aerobic conditions are generally lower than 10 days at 20 °C. Thus, the presence of coprostanol in an aerobic environment can be considered an indication of recent fecal input to the waters. [124,128,130].
Isobe et al. [130] reported a study carried out in the Mekong Delta (Vietnam) and in the Tokyo metropolitan area. During the wet season in the Mekong Delta, higher bacterial densities were observed in rivers, probably due to the higher bacterial inputs from soil particles with runoff. In Tokyo, higher bacterial densities were usually observed during summer, followed by those in the typhoon aftermath and winter. Significant correlations between the concentrations of E. coli and coprostanol (log scale) were found in all surveys. It was concluded that the determination of coprostanol can improve standard microbiological assays of fecal pollution.
Reports from several regions throughout the world indicate variable quantitative relationships between fecal coliform densities and coprostanol concentrations. In the Derwent Estuary and Sydney region (Australia), coprostanol concentration of 400 ng/L corresponded to 1,000 CFU of fecal coliforms/100 mL. In the Mekong Delta, during the wet season, this coliform density corresponded to 30 ng coprostanol/L, and in the dry seasons, 100 ng/L. In Tokyo metropolitan area, these values were 30 ng coprostanol/L, in summer, and 100 ng coprostanol/L, in a typhoon aftermath. These differences were interpreted as a result of differences in water temperature and soil particle concentration [130].
Fecal sterol analysis, although expensive and complex, has resolved problems of source attribution in urban and rural environments not possible with use of traditional fecal indicator bacteria [124]. These chemical indicators are especially useful in environments which allow survival and growth of fecal bacteria. For instance, in tropical regions, characterized by high temperatures and frequent rainstorms that facilitate erosion of soils, fecal bacteria can proliferate in environmental waters reaching densities that are not representative of real sewage inputs in the environment [130].
9. Sources of Fecal Bacterial Pollution of Enviromental Waters
9.1. Sources of Surface and Groundwater Contamination
Determinations carried out in the sewage systems of urbanized areas have confirmed the presence of high numbers of intestinal bacteria. Treatment of sewage reduces the concentration of these bacteria by 1–2 logs, but effluent still contains high levels of intestinal bacteria (Table 8). Effluents from sewage treatment plants can be a source of contamination of surface waters with fecal bacteria.
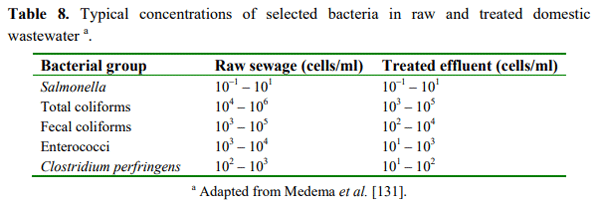
Septic tanks, cesspools, latrines and other on-site systems are widely used for wastewater storage and treatment. The water percolating from these facilities contains bacteria that may contaminate groundwater supplies.
Many farmers use cellars, tanks or landfills to store manure. Water leaching from these storage sites may also contaminate groundwater, especially during periods of rainfall. The application of animal manure to agricultural lands as fertilizer is common practice throughout the world. Bacteria present in the manure may leach into the groundwater.
The potential for bacteria present in human and animal wastes to contaminate water in nearby wells needs special attention [131]. An important source of contamination of surface and ground waters is runoff water from agricultural and pasture lands, and urban areas.
Fecal bacteria enter surface water by direct deposit of feces and by overland runoff. The movement of animal wastes into surface waters can be a major factor contributing to the pollution of available water in many regions. Over one-third of the land area of USA is used for grazing livestock and receives 50% of all livestock wastes.
In a study reported by Doran and Linn [108], runoff from a cow-calf pasture in eastern Nebraska was monitored during a three-year period. Rainfall runoff from the grazed area contained 5 to 10 times more fecal coliforms than runoff from the fenced, ungrazed area. However, fecal streptococci counts were higher in runoff from the ungrazed area and reflected the contributions from wildlife.
Urban and suburban areas are dominated by impervious cover. During storms, rainwater flows across these impervious surfaces, mobilizing contaminants. The pollutants carried in runoff originate from a variety of urban and suburban nonpoint sources. Contaminants commonly found in stormwater runoff include fecal and pathogenic bacteria. Stormwater transports pollutants to water bodies such as lakes and streams [132].
Enterococci and E. coli can be found in high numbers in most storm drains and creeks. In Southern California (USA), Ferguson et al. [133] found high levels of enterococci (Enterococcus faecalis, Enterococcus faecium, Enterococcus hirae, Enterococcus casseliflavus and Enterococcus mundtii) in intertidal sediments in a seasonal river, and near a storm drain outlet.
9.2. Survival in Surface Water
Most intestinal bacteria that contaminate environmental waters are not able to survive and multiply in this environment. Survival rates vary widely among fecal bacteria introduced in environmental waters. Pathogenic enteric bacteria and E. coli display low survival rates (Table 9).
The ability of fecal bacteria to survive in environmental waters generally increases as the temperature decreases. Others factors that influence survival include dissolved organic carbon concentration, sunlight intensity and the ability to enter the viable but non-culturable state [131].
In a comparative study on the survival of 10 different coliform species (E. coli, Citrobacter freundii, Citrobacter youngae, Klebsiella pneumoniae, K. oxytoca, Enterobacter amnigenus, Enterobacter cloacae subsp. cloacae, and Pantoea agglomerans (Enterobacter agglomerans)) inoculated in sterilized river water with different concentrations of dissolved organic carbon, Boualam et al. [134] found that only C. freundii, K. pneumoniae and E. cloacae subsp. cloacae remained cultivable after 96 hours of incubation. In a posterior study, using the same bacteria and medium, Boualam et al. [135] found that after 28 days, only C. freundii and E. cloacae subsp. cloacae survived.
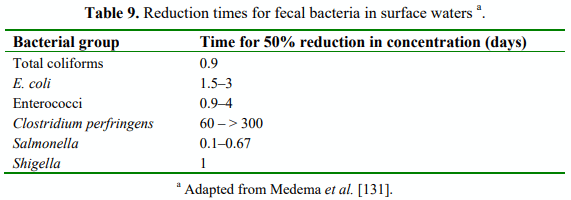
Baudišová [136] reported a comparative study on the survival of total coliforms, fecal coliforms and E. coli, in sterile and non-sterile river water. In sterile water, all bacteria survived for many months. However, in non-sterile conditions (closer to true environmental conditions), the elimination rate of all bacteria was considerably faster. Total coliforms survived the longest and E. coli the shortest.
9.3. Survival in Groundwater
Survival of bacteria in groundwater is influenced by several factors, namely the survival in soil, since in order to reach the groundwater bacteria have to percolate through the soil. Generally, survival in soil (and concomitantly in groundwater) is enhanced by low temperatures, high soil humidity, neutral or alkaline soil pH and the presence of organic carbon [131].
10. Which Indicators of Fecal Pollution Should be Used?
Several fecal indicator bacteria in environmental waters are in current use. From these stand out fecal coliforms, E. coli and enterococci [1,6,137]. In environmental waters, most fecal coliform strains are E. coli.
In particular situations, the presence of E. coli is definitively not associated with fecal pollution. These situations were firstly detected in some African countries, namely Nigeria, Ivory Coast and New Guinea (although not in others, such as Uganda) [42,99].
Recent studies carried out in temperate zones indicated that E. coli can persist in secondary, nonhost habitats, outside the hot tropical areas, and become naturalized in these habitats. Byappanahalli et al. [138] reported that E. coli could be isolated from coastal temperate forest soils in Indiana (USA). The aquatic alga Cladophora glomerata (L.) from several Lake Michigan beaches was shown to harbor high densities of E. coli [139].
Ishii et al. [140] reported a study on the survival of E. coli in temperate riverine soils of northern Minnesota (USA). Viable E. coli populations were repeatedly isolated from northern temperate soils in three Lake Superior watersheds. Seasonal variation in the population density of soilborne E. coli was observed; the greatest cell densities were found in the summer to fall, and the lowest numbers, occurred during the winter to spring months. Horizontal, fluorophore-enhanced repetitive extragenic palindromic PCR (HFERP) DNA fingerprint analyses indicated that identical soilborne E. coli genotypes, overwintered in frozen soil and were present over time, and that these strains were different from E. coli strains obtained from wildlife commonly found in the studied habitats or river water. Soilborne E. coli strains had HFERP DNA fingerprints that were unique to specific soils and locations. In laboratory studies, naturalized E. coli strains had the ability to grow and replicate to high cell densities, in nonsterile soils when incubated at 30 or 37 °C and survived longer than 1 month when soil temperatures were lower than 25 °C. It was concluded that these E. coli strains became naturalized, autochthonous members of the soil microbial community.
In a latter paper, Ksoll et al. [141] studied epilithic periphyton communities at three sites on the Minnesota shoreline of Lake Superior (USA). Fecal coliform densities increased up to 4 orders of magnitude in early summer, and decreased during autumn. HFERP DNA fingerprint analyses indicated that waterfowl (geese, terns, and gulls) were the major primary source of periphyton E. coli strains that could be identified. Periphyton and sewage effluent were also major potential sources. Several periphyton E. coli isolates were genotypically identical, repeatedly isolated over time. Inoculated E. coli rapidly colonized natural periphyton in laboratory microcosms and persisted for several weeks, and some cells were released to the overlying water. It was concluded that E. coli had became a naturalized member of the bacterial periphyton communities.
The presence, persistence, and possible naturalization of E. coli in these habitats can confound the use of fecal coliforms as a reliable indicator of recent fecal contamination of environmental waters. Future studies should consider other nonhost habitats as potential sources of fecal coliform bacteria in aquatic environments.
Considering these limitations, it appears to be advisable, in order to check the microbiological quality of drinking water, to complement the determination of Escherichia coli with the assay of enterococci. This rationale that has been followed, for many years, in the making of the drinking-water legislation in the European Union.
However, for many developing countries, where limited financial resources are the norm and reality, the routine determination of these two parameters can be difficult to implement. In these circumstances, it appears common sense that is better to determine a (good) parameter, such as Escherichia coli, than have no analysis done.
In this context, USA legislation emerges as a pragmatic approach to the problem. According to the American legislation, total coliforms are the routine parameter to be determined. Only when these determinations are repeatedly positive, it is mandatory to assess fecal coliforms [142,143]. Although total coliforms are not necessarily fecal bacteria, the rationale behind this system is correct, since: (1) a positive test in fecal coliforms (which is our target) is necessarily positive in the total coliform procedure; (2) the inverse is not necessarily true; (3) total coliforms are easily and cheaply assayed in waters.
As an alternative to the determination of both E. coli and enterococci, the assay of ammonia in environmental waters can be useful and complement the determination of fecal coliforms.
Ammonia is one of the key molecules in the nitrogen cycle. The presence of ammonia in surface waters can be due to direct contamination by agricultural fertilizers, and/or to microbial degradation of proteins, nucleic acids and urea, implying, therefore, the presence of a considerable concentration of organic matter in the water. Ammonia is rapidly oxidized in the environment and is typically found in natural waters at concentrations less than 0.1 mg/L. Concentrations significantly above this indicate gross contamination by fresh sanitary waste, where ammonia levels are typically very high (tens or hundreds of mg/L) [84].
Espigares et al. [144] reported a comparative study of chemical and microbiological indicators (total and fecal coliforms, fecal streptococci and sulphite-reducing clostridia) in a stretch of the Guadalquivir River (Spain) and its affluents. Total coliforms were correlated with fecal coliforms, but were not correlated with fecal streptococci and clostridia. Fecal coliforms were correlated with the other indicators. Fecal streptococci and sulphite-reducing clostridia were correlated with the other indicators except for total coliforms. All these microbiological indicators were correlated with dissolved oxygen (negatively), dissolved organic carbon and ammonia (positively). Cabral and Marques [85] in a study of a polluted river (Febros) in the Great Oporto area (northwest Portugal) found that ammonia was significantly correlated with all the microbiological assayed parameters—total and fecal coliforms, fecal streptococci and enterococci. These correlations are most probably due to the carry-over of organic matter in wastewaters, and to a high microbial ammonification activity [85].
Simple and rapid in-field tests and automated and continuous systems are available for the assay of ammonia in environmental waters. More studies are needed in order to confirm the use of ammonia as a reliable parameter in a preliminary screening for emergency fecal pollution outbreaks.
11. Conclusions
- Safe drinking water for all is one of the major challenges of the 21st century.
- Microbiological control of drinking water should be the norm everywhere.
- Routine basic microbiological analysis of drinking water should be carried out by assaying the presence of Escherichia coli by the culture methods. On-line monitoring of glucuronidase activity is currently too insensitive to replace culture based detection of E. coli but is a valuable complementary tool for high temporal resolution monitoring. Whenever financial resources are available, coliform determinations should be complemented with the quantification of enterococci.
- More studies are needed in order to check if ammonia is reliable for a preliminary screening for emergency fecal pollution outbreaks.
- Financial resources should be devoted to a better understanding of the ecology and behavior of human and animal fecal bacteria in environmental waters.
This article was originally published in International Journal of Environmental Research and Public Health 2010, 7, 3657-3703; doi:10.3390/ijerph7103657. This is an Open Access article distributed under the terms and conditions of the Creative Commons Attribution license (http://creativecommons.org/licenses/by/3.0/).