INTRODUCTION
During the last decade, the decrease in sequencing costs coupled with innovations in computational technologies (1) has remarkably advanced our understanding of the composition and function of microbial communities residing in diverse environments (2). Accordingly, the roles of microbiota in health and diseases have been well-documented in wide range of animals, yet limited microbiota studies have been conducted so far in turkeys.
One study investigated the succession of intestinal microbiota in the ceca of male turkeys, where decrease in Clostridium species and increase in Bacteroides uniformis were reported over time (3). The cecal bacterial succession in relation to the Campylobacter jejuni and Campylobacter coli loads has also previously been reported (4). Similar with the previous findings, the cecal bacterial communities were changed in a time-dependent manner and Campylobacter loads were correlated with the acute microbial community transition. In another study, considerable divergence of the cecal bacterial genera was found in the domestic turkeys as compared to the wild ones, though bacterial compositions at higher taxonomic levels were similar (5). Although, these studies provide valuable insights regarding intestinal microbiota in turkeys, they are based on low-resolution molecular fingerprinting methods, such as terminal restriction fragment length polymorphism (T-RFLP) or automated ribosomal intergenic spacer analysis (ARISA) (3–5). These methods have certain limitations in terms of accurately depicting microbial diversity in samples, especially for those samples with higher level taxon richness (6).
Along with the advancement in sequencing technologies, the intestinal microbiota of turkey has been investigated using high-throughput next generation sequencing of 16S rRNA genes (7–10). These studies were conducted in turkeys to characterize the microbiota along the gastrointestinal tract (10), litter microbiotas (8), and their relation in terms of body weight gain (7), antibiotics treatment (8), and hemorrhagic enteritis virus (9). Mostly, these studies were conducted in experimental animal settings which might not properly reflects the turkey microbiotas in commercial farms, demanding the need of more comprehensive survey of turkey microbiota in commercial farms.
In this study, we characterized the litter microbiotas from different flocks of five different commercial farms at different time points of turkey production. We used the boot swab samples for better representation of microbiotas present at the farm level. A previous study demonstrated that the litter microbiotas in turkey were most closely related to the ileal microbiotas among other regions (8), suggesting the litter microbiota data in this study might reflect well the dynamic changes in the ileal microbial communities of turkeys in the respective farms.
MATERIALS AND METHODS
Collection of Samples
Samples were collected from five commercial turkey farms (H, M, V, K, and R) in the Northwest Arkansas at different time points including one farm (R) that had incidence of cellulitis at the time of the sampling. All these farms were individually owned and operated under the contract of Cargill, Inc during the period of the sampling. From all farms except for R farm, the samples were collected from each side of the barn’s quadrant by walking with a pair of boots with sponge swab attached at the bottom. Since each barn has four quadrants, a total of 8 (4 × 2 = 8) samples were collected from each barn. From R farm, samples were collected to be used as a cellulitis-positive samples. That is, four sponge swab samples directly from the birds with cellulitis (R farm Bird: RB) and four boot sponge swab samples from the litter surrounding the cellulitis-positive birds (R farm Litter: RL) were collected. Total 246 sponge swab samples were collected and used for 16S rRNA gene profiling analysis. The summary of the samples with the information on the farms, flocks, age of birds, and number of samples is shown in Table 1.
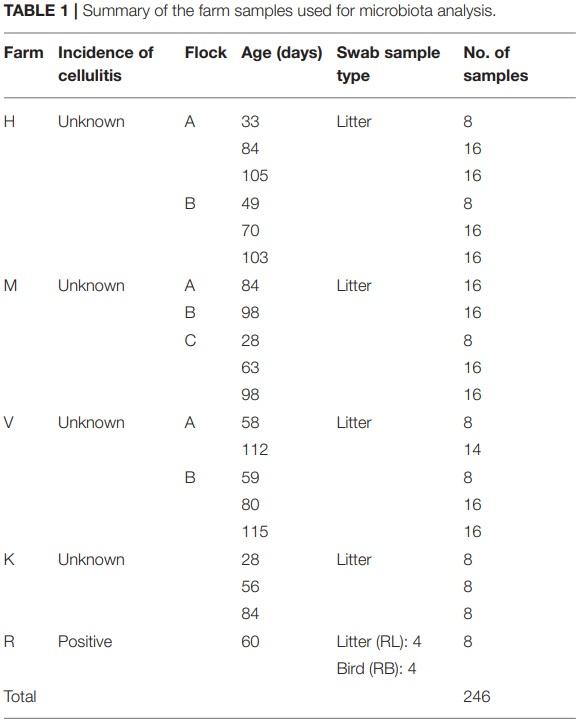
DNA Extraction
We developed the protocol for efficient extraction of metagenomic DNA in boot swab samples. For this purpose, each sponge swab sample was transferred to the sterile stomacher bag with filter (Seward). After adding 20 ml of sterile PBS buffer, the sponge swab samples were stomached for 2 min in a stomacher (Lab Blender 400 series). In order to obtain uniformity in sponge samples, litter debris attached to each samples were removed aseptically before transferring to stomacher bags. The filtered contents from each sample after stomaching were transferred to 15 ml sterile tube and centrifuged @8,000 rpm for 10 min to make cell pellets. The supernatant from each sample after centrifugation was removed, whereas pellets containing bacterial cells were retained and used for DNA extraction using QIAamp Fast DNA Stool Minikit (Qiagen). All the procedures for DNA extraction were followed according to the manufacturer’s instructions except for the incorporation of a bead beating step. Bead beating step was incorporated in the protocol because bead beating was reported to increase DNA yield and taxon abundances (11). For bead beating, pellet from each sample was resuspended in 1 ml Inhibit Ex Buffer provided with the kit, which was then transferred to 2 ml microcentrifuge tube with a screw cap (Thermofisher Scientific) containing 0.25 ml of sterile 0.1 mm glass leads (BioSpec). Bead beating was performed using Bead mill 24 (Fisher Scientific) for 6 cycles where 0.30 s run for each cycle and 0.11 s stopping time between the cycles. After bead beating, samples were incubated at 70°C for 10 min, followed by manufacturer’s protocol for downstream steps and finally DNA was eluted in 30 µl of elution buffer. Negative control sample was also included in the DNA extraction step.
PCR and Library Preparation for Sequencing
V4 region of 16S rRNA gene from genomic DNA of each farm sample and a negative control from DNA extraction step and a positive control using a mock community DNA sample (ZymoBIOMICS Microbial Community DNA Standard II, Zymo Research, Cat# D6311) was amplified using the primers 515F (12) and 806R (13). The library of amplicons for sequencing was prepared according to the 16S Illumina PCR protocol described in the Earth Microbiome project [http:// www.earthmicrobiome.org; (14)] with slight modifications. In brief, PlatinumTM II Hot-Start Green PCR Master Mix (2X) user guide protocol (Thermofisher Scientific, Catalog No. 14000013) was used to conduct PCR in a 25 µl final reaction volume and 35 amplification cycles. The thermocycling condition of PCR included an initial denaturation step at 94°C for 2 min, followed by 35 cycles of 0.5 min at 94°C, 0.5 min at 60°C, and 0.5 min at 68°C, and a final extension of 5 min at 68°C. The length of amplified product was confirmed with 1% agarose gel electrophoresis and equal amount (∼300 ng) of amplicons from each sample as measured by Qubit dsDNA BR Assay Kit (ThermoFisher Scientific, Catalog No. Q32850) were pooled together. The pooled amplicons were finally ran on 1% agarose gel electrophoresis, purified using Zymoclean Gel DNA Recovery Kit (Zymo Research, Catalog No. D4007), and sequenced using Illumina MiSeq with paired end 300 cycle options.
Amplicons Sequence Analysis
Nebula cloud computing platform at the University of Arkansas was used to process raw sequencing reads in QIIME 2 version 2018.8 (15) utilizing the pipelines developed for paired-end data types. In sum, “demux emp-paired” method of q2-demux plugin was used to demultiplex sequencing reads followed by quality filtering and denoising with “dada2 denoise-paired” method of q2-dada2 (16) plugin available at QIIME 2. The truncation length of forward and reverse reads was set at 240 and 200 bp, respectively, which is based on the quality score criteria (≥30). Taxonomic assignment was performed using a Naive Bayes classifier (17) pre trained with SILVA (Version 132) 99% OTUs (18, 19) and q2-feature-classifier plugin, where the sequences have been trimmed to include only the V4 region of the 16S rRNA gene defined by the 515F/806R primer pair. The coremetrics-phylogenetic method at a sampling depth of 17,000 was used to analyze Alpha and Beta diversity. Alpha diversity calculated by Shannon’s diversity index (20) and Observed OTUs metric, while beta diversity calculated by unweighted UniFrac distance metric (21) and Bray Curtis (22) are presented. All figures except Emperor plots were created using ggplot2 packages of R (23). The significant differences in alpha diversity were calculated using alpha-group-significance command of QIIME2 which uses Kruskal-Wallis test. In contrary, statistical differences in beta diversity among groups were calculated by PERMANOVA (24) test using beta-group-significance command of QIIME2 with pairwise option. For both diversities analysis, the corrected P-values for multiple comparisons (q) were used to report significant difference between two groups, where the level of significance was set at adjusted P < 0.05.
RESULTS
Summary of DNA Sequencing Analysis
The summarized feature table resulted in total 10,863,650 sequence reads from the 246 samples that ranged from 17,134 to 82,383 reads per sample. The median and mean ± SE reads per sample were 42,949.5 and 44,161.2 ± 787.9, respectively. In addition, there were altogether 3,057 unique features (ASV) from all 246 samples.
Phylum Level Compositions of Litter Bacterial Communities
At phylum level, 11 major bacterial phyla and one phylum (Euryarchaeota; 0.08%) that belongs to the domain Archaea were detected from four farm samples, excluding the samples from R farm (cellulitis-positive farm). These phyla constituted 99.96% of the total sequence reads. Among the major bacterial phyla, Firmicutes was the predominant phylum (51.10%), followed by Actinobacteria (31.69%), Proteobacteria (8.30%), and Bacteroidetes (8.18%). Other minor phyla included Cyanobacteria, Synergistetes, Epsilonobacteraeota, Kiritimatiellaeota, Tenericutes, Fusobacteria, and Verrucomicrobia whose relative abundance ranged from 0.01 to 0.24% and constituted < 1% in total. The relative abundance of the major phyla across four different farms is shown in Figure 1A. Irrespective of farms, the Firmicutes was the predominant phylum which was found the highest in H farm (55.47%), while it was the lowest in K farm (34.49%) as shown in Figure 1A. On the contrary, Proteobacteria was found the highest in K farm (26.92%), whereas the Actinobacteria was found the highest in V farm (41.51%). The phylum Bacteroidetes was found the highest in M farm (12.04%) as shown in Figure 1A.
In addition, the microbial compositions at phylum level were also different among the flocks within the same farm as illustrated in Figure 1B. The variations in the relative abundance of major phyla among the flocks of same farm was further achieved due to differences in the ages of birds as illustrated in Figure 1C. Generally, Firmicutes was found higher in each flock of the farms rearing younger birds, while the Actinobacteria and Bacteroidetes were found higher in the flocks of the older birds (Figure 1C). However, their relative abundance varied depending upon the farms and flocks within the same farm, but does not show apparent patterns of change over the ages. Similarly, the Proteobacteria was highly enriched (47.97%) especially in K farm housing 56 days old turkeys as shown in Figure 1C. In case of Bacteroidetes, this phylum was found the highest in the flock C of M farm housing 63 days old turkeys (M-C-63; 22.38%) followed by K farm having turkeys at 84 days old (K-84; 17.26%).
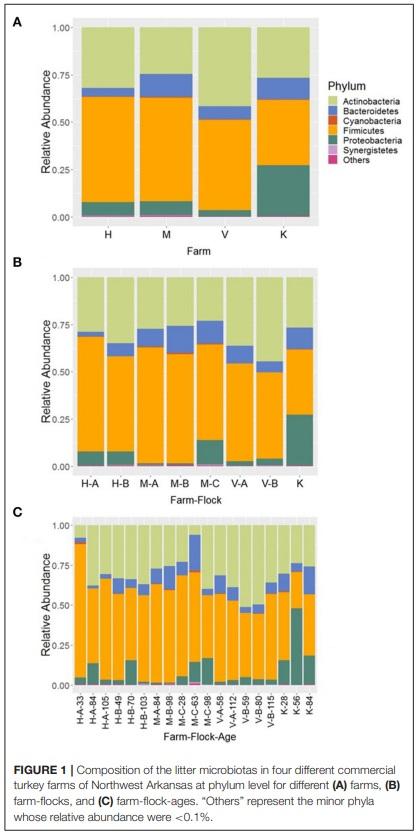
In the samples from cellulitis-positive farm (R farm), Firmicutes was detected as the predominant phylum (66.06%) followed by Proteobacteria (17.77%), Actinobacteria (14.44%), and Bacteroidetes (1.47%), which constituted 99.97% of the total sequence reads. Although no direct comparisons can be made, the relative abundance of phyla Firmicutes and Proteobacteria were increased, while the relative abundance of phyla Actinobacteria and Bacteroidetes decreased in cellulitis-positive farm samples in comparison to the rest of the farm samples. The distribution of the relative abundance of major four phyla across different samples from R farm is shown in Figure 2A. The phylum Bacteroidetes was significantly reduced in bird swab samples (RB; 0.19%) as compared to the litter swab samples (RL; 2.75%) at P < 0.05 (Kruskal-Wallis test). In addition, Proteobacteria was numerically enriched in RB (26.22 vs. 9.31%), whereas Firmicutes (72.15 vs. 59.98%) and Actinobacteria (15.60 vs. 13.28%) were numerically abundant in RL.
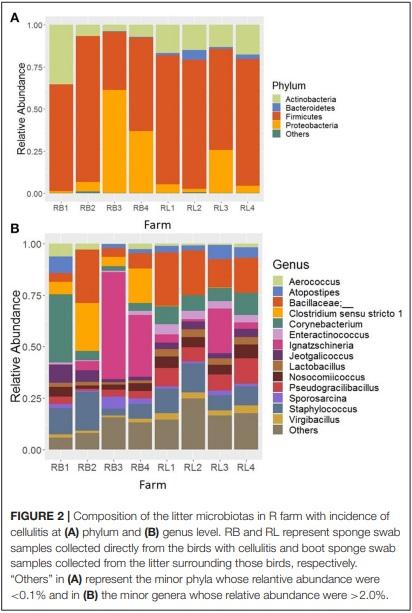
Genus Level Compositions of Litter Bacterial Communities
At genus level, 13 major bacterial genera were identified whose average relative abundance were > 2% when summed across all four farm samples excluding R farm. Among these genera, the relative abundance of the genus Corynebacterium (16.66%) was found the highest, followed by Staphylococcus (11.03%), Brevibacterium (6.01%), Megamonas (5.13%), Brachybacterium (4.83%), Jeotgalicoccus (4.76%), Lactobacillus (3.72%), Bacteroides (3.66%), Escherichia-Shigella (3.33%), Aerococcus (2.62%), Prevotellaceae UCG-001 (2.27%), Pseudogracibacilibacillus (2.24%), and Oceanisphaera (2.04%).
The relative abundance of the major genera across four different farms is shown in Figure 3A. The genus Corynebacterium was the predominant genus in H (21.78%) and V (17.30%) farm, however, the genera Megamonas (12.39%) and EscherichiaShigella (17.79%) were significantly higher in the M and K farm, respectively, at P < 0.05. Moreover, the composition of bacterial genera varied not only across the different flocks of the same farm (Figure 3B), but was also affected by ages of birds within the same flock (Figure 3C). For instance, the genus Megamonas was highly enriched in flock C of the M Farm rearing turkeys of 28 (M-C-28; 19.02%) and 63 days old (M-C-63; 27.60%), but very lower level of Megamonas was detected at the same flock rearing 98 days old (M-C-98; 1.95%) turkeys. Similarly, the genus Escherichia-Shigella was highly abundant in K farm having the turkeys of 56 days old (42.83%) (Figure 3C). Similarly, the genus Bacteroides was the highest from the flock C of M Farm rearing turkeys of 63 days old (M-C-63; 13.70%). Regarding Corynebacterium and Staphylococcus, they were present at significant amount throughout all ages and flocks of the farms (Figure 3C), except in the flock C of M Farm rearing turkeys of 63 days old (M-C-63) where they were found at 0.97 and 1.07%, respectively.
The top 14 major genera whose relative abundances were on average > 2% when summed across all samples recovered from R farm are shown in Figure 2B. On the contrary to the other farm samples, the samples from this cellulitis-positive farm consisted of unknown genera of the family Bacillaceae (15.05%) and Ignatzschineria (14.58%), which were only 1.67 and 0.035% in other farm samples, respectively. Other important genera included Staphylococcus (10.60%), Corynebacterium (9.65%), Clostridium sensu stricto 1 (6.34%), Pseudogracilibacillus (5.95%), Nosocomiicoccus (4.28%), Jeotgalicoccus (3.88%), Atopostipes (3.69%), Lactobacillus (2.55%), Enteractinococcus (2.54%), Virgibacillus (2.20%), Sporosarcina (2.09%), and Aerococcus (2.06%). Although direct comparisons cannot be made, it seems that different genera were differentially abundant between the cellulitis-positive farm samples (Figure 2B) and the rest of the farm samples (Figure 3A). Moreover, as seen in Figure 2B, there existed differences in the relative abundance of major bacterial genera between RL (litter swabs) and RB (bird swabs) groups. For instance, the genera Enteractinococcus, Pseudogracilibacillus, Virgibacillus, Nosocomiicoccus, and Lactobacillus were significantly higher in RL group, while the Clostridium sensu stricto 1 was significantly higher in RB group (Kruskal-Wallis test, P < 0.05).
When all ASVs that belong to the Clostridium sensu stricto 1 were compared with Clostridium septicum 16S rRNA gene sequence, they showed > 97% similarity. Thus, we believed that the sequence reads of Clostridium sensu stricto 1 belong to C. septicum as C. septicum is considered as the primary etiological agent of cellulitis in turkeys (25) and these sequence reads were detected exclusively in the samples from R farm.
Core Bacterial Genera
The number of core bacterial genera that were present in the 50–100% of all litter swab samples except those from R farm are shown in Supplementary Figure 1. There were 90 core bacterial genera found in 50% of the samples, while only 4 genera (Staphylococcus, Brevibacterium, Brachybacterium, and Lactobacillus) were found in all samples (Supplementary Figure 1). In addition, 20 core bacterial genera were identified in 95% of the samples, which include Corynebacterium, Staphylococcus, Jeotgalicoccus, Brevibacterium, Brachybacterium, Lactobacillus, Bacteroides, Pseudogracilibacillus, Aerococcus, Atopostipes, Virgibacillus, an unknown genus of Lachnospiraceae, Facklamia, Weissella, Escherichia-Shigella, Bifidobacterium, Enterococcus, Phascolarctobacterium, Sellimonas, and Subdoligranulum.
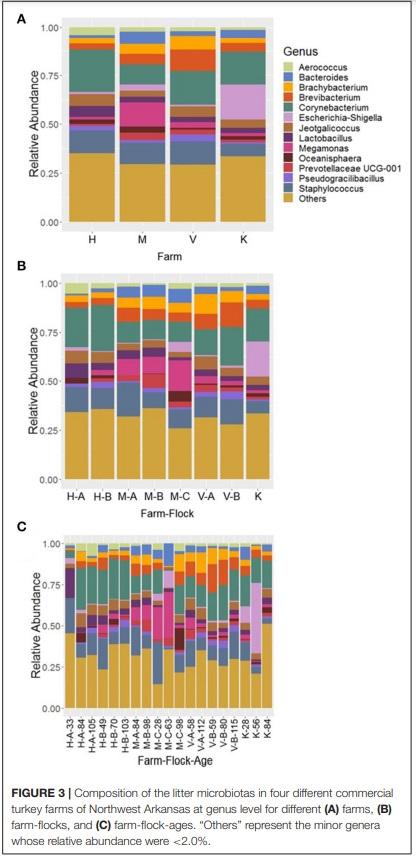
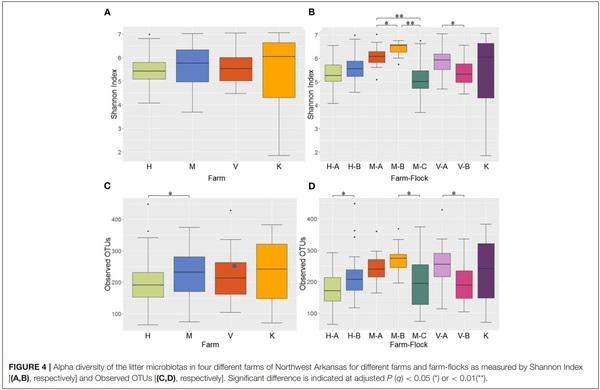
On the contrary, the number of core bacterial genera that were present in the 50–100% of the samples from R farm (with the incidence of cellulitis) are shown in Supplementary Figure 2. As shown in Supplementary Figure 2, 73 core bacterial genera were detected in 50% of samples, whereas 24 genera were present in all 100% samples. These genera include unknown genus of Bacillaceae, Staphylococcus, Corynebacterium, Pseudogracilibacillus, Nosocomiicoccus, Ignatzschineria, Jeotgalicoccus, Atopostipes, Enteractinococcus, Lactobacillus, Virgibacillus, Sporosarcina, Aerococcus, Weissella, Brevibacterium, an uncultured genus of Bacillaceae, Bifidobacterium, Brachybacterium, an unknown genus of Lachnospiraceae, Salinicoccus, Subdoligranulum, Blautia, Sellimonas, and Romboutsia.
Alpha Diversity
Alpha diversity of the microbial communities was measured using Shannon and observed OTUs indices. When the Shannon index was compared among the 4 different farms, no significant difference was observed in alpha diversity (Figure 4A). However, when the Shannon index was compared across different flocks within the same farms, all pairwise comparisons among the 3 flocks (A, B, and C) in M Farm showed significant differences (P < 0.05) (Figure 4B). Similarly, the two flocks (A and B) in V Farm showed significant difference in the Shannon index (Figure 4B; adjusted P < 0.05). Similar, yet slightly different results were observed with observed OTU index. There was significant difference in alpha diversity between H and M Farms (Figure 4C) (P < 0.05). When the flocks within the same farms were compared, significant difference (P < 0.05) was observed between the flock A and B in H Farm, between the flock B and C in M Farm, and between the flock A and B in V Farm (Figure 4D).
Beta Diversity
Beta diversity of the microbial communities was measured by unweighted distance and Bray-Curtis metrics. All pairwise combinations of various flocks from four turkey farms showed significant difference in microbial communities among the groups as indicated by both unweighted distance metric (Figure 5A; adjusted P < 0.001) and Bray-Curtis distance metric (Figure 5B; adjusted P < 0.01). In addition, within H farm, all possible pairwise comparisons of flocks and ages combinations showed significantly different microbial community structure in terms of both unweighted distance metrics (Figure 6A) and Bray-Curtis (Figure 6B) at adjusted P < 0.001.
DISCUSSION
In the current study, we characterized the microbiotas associated with the litter from five different commercial farms of the Northwest Arkansas, including a farm with positive incidence of cellulitis. To our knowledge, this is the first study that used boot swab samples for comprehensive survey of litter microbiotas in commercial turkey farms. Previously, boot swab was used for detection of Mycobacterium avium subsp. paratuberculosis (MAP) in cattle herds (26). By the culture of boot swab samples, they were able to isolate MAP from 90.6% of MAP confirmed cattle herds. We also noticed significant enrichment of Clostridium sensu stricto 1 in farm samples with positive incidence of cellulitis (R farm). When the sequences of all ASVs identified as Clostridium sensu stricto 1 were compared with C. septicum 16S rRNA gene sequence, they shared > 97% sequence identity. Furthermore, the results of a nested qPCR assay targetting alpha toxin gene (csa) of C. septicum gave strong amplification signals from the farm samples with incidence of cellulitis (data not shown). Thus, we believe that the sequences that were classified as Clostridium sensu stricto 1 belong to C. septicum, since cellulitis in turkey is considered to be primarily caused by C. septicum (25). This further suggests that the boot swab samples can serve as an easy and cost-effective technique for the collection of environmental samples for detection of various pathogens as well as characterization of litter microbiotas in poultry farms. Moreover, studies on litter microbiota can reflect the changes in the microbial communities of the poultry as the litter microbial communities correlate with those residing in the poultry hosts (8), which are further affected by the litter types (27).
It was found different flocks with in the same farm contributed to differences in the composition and structures of litter microbial communities, which are further affected by the ages of turkeys. Age as a major driving factor of turkey microbiota was also reported previously (7, 8). Differences in environmental conditions can play a vital role in the initial maturation of turkey microbiota, in addition to the flock types (7). Although the trend is not linear, we noticed the higher abundance of Firmicutes from the flocks rearing younger age of birds, while Actinobacteria and Bacteroidetes were reported higher from the flocks rearing older birds.
Interestingly, the phyla Proteobacteria and Bacteroidetes were highly enriched in the flock C of the M farm with 63 days old turkeys (M-C-63) and K farm housing 56 days old turkeys (K-56), respectively. This was reflected at the genus level by increased abundance of Escherichia-Shigella and Bacteroides in the respective farms. The Proteobacteria is the phylum that contains several pathogenic Increase in relative abundance of Gram-negative genera such as Escherichia and Shigella is generally considered as the signature of gut dysbiosis (28). Therefore, increase in the relative abundance of the phylum Proteobacteria and the subsequent increase of genera Escherichia-Shigella in the K farm (K-56) might be indicative of gut dysbiosis of turkeys, though we are lacking any data to support our hypothesis. Another important observation was that the genera Bacteroides and Megamonas were present the most in the M-C-63 group. The increase in the relative abundance of Bacteroides in the particular farm was explained by the highest abundance of the phylum Bacteroidetes in that farm. In addition, the genus Staphylococcus was highly reduced in M-C-63 as compared to the other groups. The Bacteroides is a genus of Gram- negative bacteria that are well-known for its ability to degrade complex plant carbohydrates and host derived glycan. This group of bacteria can exert beneficial effects toward the hosts’ health, maintaining gut homeostasis. However, such effects were found to vary among the studies and due to the strains of Bacteroides (29, 30). The increase in the abundance of the genera Bacteroides and Megamonas might be associated with the reduction of Staphylococcus in MC-63 group. Although C. septicum is considered as primary etiological agent, Staphylococcus aureus was also reported to be associated with cellulitis in turkeys (31). This was further supported by our results from the farm with positive incidence of cellulitis, where the Staphylococcus was detected in all samples, suggesting the possible association of Staphylococcus in cellulitis of turkeys.
Moreover, only 4 core genera (Staphylococcus, Brevibacterium, Brachybacterium, and Lactobacillus) were found in all samples of 4 farms excluding those from R farm, whereas 24 core genera were present in all samples from R farm that had cellulitis. The important core genera in cellulitis-positive samples were Corynebacterium, an unknown genus of family Bacillaceae, Clostridium sensu stricto 1 (> 97% similarity with C. septicum), and Ignatzschineria among others. These genera should be considered while describing the etiopathogenesis of cellulitis in turkeys. The genus Ignatzschineria was noticeably enriched in some of the positive samples especially in RB3 (51.97%), RB4 (29.91%), and RL3 (21.70%) as shown in Figure 2B. Ignatzschineria is a genus of Gram-negative bacteria that has been associated with necrotizing wounds colonized by maggots (32–34). This group of bacteria are common isolates from the larvae of the parasitic flesh fly (Wohlfahrtia magnifica) and two species, I. indica (32, 34) and I. ureiclastica (33) were isolated from the bacteremia following maggot’s infestation of the wounds in humans. This suggests that if the cellulitis is not properly treated in a timely manner, it might create further complications including septicemia.
In sum, boot swab samples were successfully used to investigate the litter microbial communities of the commercial turkey farms of the Northwest Arkansas. Majority of the microbial taxa identified using boot swabs belong to the microbiota residing in the gut of the poultry, which suggests that the litter microbiota might be used to reflect the microbial changes in the hosts. The composition and diversities of litter microbial communities varied even among the flocks of the same farm, which were further affected by the age of turkeys. The core bacterial genera from samples with cellulitis differed from those for the rest of the farm samples. In addition, several bacterial genera such as Corynebacterium, Staphylococcus, Ignatzschineria, and unknown genus of family Bacillaceae that were identified as core members in the cellulitis-positive samples might be correlated with incidence of cellulitis in addition to C. septicum.
This article was originally published in Frontiers in Veterinary Science 7:596933. doi: 10.3389/fvets.2020.596933. This is an Open Access article distributed under the terms of the Creative Commons Attribution License (CC BY).