INTRODUCTION
The intestinal epithelium constitutes the largest and most important barrier against external environmental agents and has two critical functions: to prevent the entry of harmful intraluminal microorganisms, antigens, and toxins, and to enable the selective translocation of dietary nutrients and electrolytes into circulation (Salminen and Isolauri, 2006; Salzman, 2011; Elson and Cong, 2012). One of the basic properties of gut-associated lymphoid tissue (GALT) is oral tolerance (unresponsiveness) to harmless components of microbiota and diet (Kau et al., 2011). Inappropriate immunological reactions against food compounds, such as lactose or gluten, can lead to the breakdown of oral tolerance and the development of intestinal immune disorders (Marsh, 1992; Stepniak and Koning, 2006). For example, Celiac disease (CD) is a chronic immune-mediated enteropathy of the small intestine that is triggered by dietary wheat gluten, or related rye and barley proteins in genetically susceptible individuals (Williamson and Marsh, 2002). The clinical and pathological spectrum of CD is heterogeneous and there is no current rodent model that reproduces all aspects of human CD (Schuppan et al., 2009; Kupfer and Jabri, 2012). Patients display intestinal barrier dysfunction and altered tight junction protein expression allowing abnormal penetration of gluten-related peptides and enteric microbes, which could stimulate any subsequent immune response (Silva et al., 2012; Ströhle et al., 2013). Clinical presentation of CD can vary from a classical malabsorption syndrome to more subtle atypical gastrointestinal manifestations (similar to irritable bowel syndrome) or extra intestinal presentations (infertility, osteoporosis, and iron-deficiency anemia; James, 2005; Bianchi and Bardella, 2008; Bianchi, 2010; Assimakopoulos et al., 2011; Lef- fler, 2011). Likewise, the composition of the diet, also has a tremendous impact in digestibility and gut health of chickens (Dunsford et al., 1989; Hrncir et al., 2008; Maslowski and Mackay, 2011). A specific example is rye-based diets versus traditional corn-based diets, where different cereals are used as the principal source of energy. The inclusion of rye in poultry diets has been fraught with problems, principally related to the production of sticky droppings, malabsorption syndrome, elevated feed conversion, and intestinal bacterial overgrowth (Campbell et al., 1983; Bedford and Schulze, 1998; Shirzadi et al., 2010). The purpose of the present study was to evaluate the utilization of rye as energy source on bacterial translocation (BT), intestinal viscosity, microbiota composition and bone mineralization when compared with a traditional cereal (corn) in broiler chickens.
MATERIALS AND METHODS
ANIMAL SOURCE AND EXPERIMENTAL DESIGN
In order to show that the same or similar results can be achieved independently, two experiments were conducted in the present study. In each experiment, forty broiler chickens were obtained from Cobb-Vantress (Siloam Springs, AR, USA); the number of animals used was based on published studies in which similar outcomes were measured (Campbell et al., 1983; Zhang and Coon, 1997; Higgins et al., 2010a,b, 2011). Chickens were randomly assigned to two groups (n = 20 chickens), and placed into isolator chambers in a controlled age-appropriate environment with unrestricted access to feed and water for 10 days. All animal handling procedures were in compliance with Institutional Animal Care and Use Committee at the University of Arkansas. At 10 days of age, in both experiments, 12 chickens in both treatment groups were randomly selected, and given an oral gavage dose of fluorescein isothiocyanate dextran (FITC-d; 2.2 mg/mL/bird; MW 3,000–5,000 Da; Sigma Aldrich Co., St. Louis, MO, USA). After 2.5 h they were humanely killed. Blood samples were collected from the femoral vein for measuring leakage of FITC-d. Liver was collected to evaluate BT. Duodenal, ileal, and cecum gut sections were collected to enumerate bacteria. For intestinal viscosity, five chickens from each group were humanely killed and intestinal digesta were individually collected. Additionally, tibias were collected for bone parameters as described below.
DIETS
The rye used in this research contained: 2710 Kcal of metabolizable energy, 12.6% of crude protein, 2.8% of crude fiber, 0.40% of Lysine, 0.16% of methionine, 0.08% of calcium, and 0.3% of phosphorus. In the case of corn it contained: 3350 Kcal of metabolizable energy, 8.27% of crude protein, 0.24% of Lysine, 0.15% of methionine, 0.01% of calcium, and 0.28% of phosphorus (Table 1). The differences in the nutritional composition of the two cereals used as principal sources of energy explain the variation between the experimental diets, which were formulated to approximate the nutritional requirements proposed by the National Research Council (1994). Due to a lower energetic contribution of rye, it was required to increase the percentage of inclusion of fat in this diet. On the contrary, in the case of crude protein in the corn diet, it was required to use a higher amount of soybeans in the feed formula to fulfill the nutritional requirements, because of the minor contribution of corn as a protein source. The small difference showed in the percentage of inclusion of lysine (L-Lysine HCL) and methionine (DL-Methionine) as purified amino acids in the diets is due to differences in amount and nutritional composition of soybean, corn, and rye in used in each diet (Table 1). However, both diets satisfy the amino acid requirements for maintenance and growth according to age, sex, and genetic line of the animals used in the present experiment. Broilers in the starter period (0–10 days of age) required between 21 and 22% of crude protein, 3035 Kcal of metabolizable energy, 1.32% of Lysine, 0.50% of Methionine, 0.90% of Calcium, and 0.45% of available phosphorus, all this specifications were taken into account to formulate and prepare the diets used in the present study.
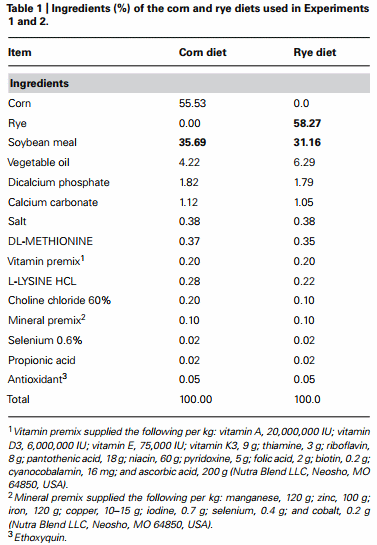
VISCOSITY
Total intestinal digesta contents were collected from Meckel’s diverticulum to the ileocecocolonic junction. For viscosity analysis, approximately 1.5 g (wet weight) of the fresh digesta was immediately placed in a microcentrifuge tube and centrifuged at 12,000×g at 4°C for 5 min. The supernatant fluid was collected and stored on ice until viscosity measurement was determined using a LVDV-I Brookfield digital cone-plate viscometer fitted with a CP-40 spindle (Brookfield Engineering, Middleboro, MA, USA). The analyzed samples and the viscometer cup were maintained at 40°C during viscosity measurement. Viscosity was measured in centipoise (cP = 1/100 dyne s/cm2) and the results were reported as log10 cP.
BACTERIAL TRANSLOCATION
Briefly, the right half of the liver was removed from each chicken, collected in sterile bags, homogenized, weighed and 1:4 wt/vol dilutions were made with sterile 0.9% saline. Ten-fold dilutions of each sample, from each group were made in a sterile 96 well Bacti flat bottom plate and the diluted samples were plated on MacConkey Agar (VWR Cat. No. 89429-342 Suwanee, GA 30024, USA). Biochemical evaluation tests, as well as identification of isolated colonies, were carried out using a bioMerieux API 50 CH test kit (catalog no. 50430, bioMerieux, Marcy l’Etoile, France).
SERUM DETERMINATION OF FITC-d
Blood was kept at room temperature for 3 h and centrifuged (1,000× g for 15 min) to separate the serum from the red blood cells. FITC-d levels of undiluted serum were measured at excitation wavelength of 485 nm and emission wavelength of 528 nm (Synergy HT, Multi-mode microplate reader, BioTek Instruments, Inc., Vermont, USA). Fluoroscence measured was then compared to a standard curve with known FITC-d concentrations. Gut leakage for each bird was reported as μg of FITC-d/mL of serum.
ENUMERATION OF BACTERIA
Whole duodenum, ileum, and both ceca were aseptically removed, separated into sterile bags, and homogenized. Samples were weighed and 1:4 wt/vol dilutions were made with sterile 0.9% saline. Ten-fold dilutions of each sample, from each group were made in a sterile 96 well Bacti flat bottom plate and the diluted samples were plated on three different culture media; to evaluate total number of lactic acid bacteria (LAB) in Man Rogosa Sharpe (Difco Lactobacilli MRS Agar VWR Cat. No. 90004-084 Suwanee, GA 30024, USA); total Enterobacteriaceae in MacConkey; and total anaerobes in tryptic soy agar with sodium thioglycolate plates (Tryptic Soy Agar, catalog no. 211822, Becton Dickinson, Sparks, MD, USA).
BONE PARAMETERS
Bone parameters were measured according to the methods as described by Zhang and Coon (1997). Tibias from each chicken were cleaned of attached tissues. Bones from the left leg were subjected to conventional bone assays and tibia from the right leg was used to determine breaking strength. The bones from left tibia were dried at 100°C for 24 h and weighed again. The samples were then incinerated in a muffle furnace (Isotemp muffle furnace, Fisher Scientific, Pittsburgh, PA, USA) at 600°C for 24 h in crucibles. Finally, the content of calcium and phosphorus in the tibia was determined using standard methods (AOAC International, 2000) and were reported as percentage of dry matter. The right tibial diaphyses from individual birds were cleaned of adherent tissues, the periosteum was removed, and the biomechanical strength of each bone was measured using an Instron 4502 (Norwood, MA, USA) material testing machine with a 100 kg Load Cell. The bones were held in identical positions and the mid-diaphyseal diameter of the bone at the site of impact was measured using a dial caliper. The maximum load at failure was determined using a three-point flexural bend fixture with a total distance of 30 mm between the two lower supporting ends. The load, defined as force in kilograms per square millimeter of cross-sectional area (kg/mm2), represents bone strength. The rate of loading was kept constant at 20 mm/min collecting 10 data points per second. The data were automatically calculated using Instron’s Series IX Software (Norwood, MA, USA).
STATISTICAL ANALYSIS
All data were subjected to one-way analysis of variance as a completely randomized design using the General Linear Models procedure of SAS (SAS Institute, 2002). Data are expressed as mean ± standard error. Significant differences among the means were determined by using Duncan’s multiple-range test at p<0.05.
RESULTS
The evaluation of body weight, intestinal viscosity, serum FITC-d, and liver BT in broiler chickens fed with a corn diet or a rye diet of Experiment 1 and 2 are summarized in Table 2. A significant (p < 0.05) reduction in body weight was observed in chickens fed with rye as compared with corn in both experiments. However, chickens fed with rye showed a increase in intestinal viscosity which was associated with elevated (p < 0.05) serum FITC-d, and an increase in BT to the liver (Table 2). Identification of the Gram-negative lactose positive bacteria in the liver was confirmed to be Escherichia coli.
Total bacterial counts in duodenum, ileum, and ceca of chickens fed with a corn or rye diet in Experiments 1 and 2 are summarized in Table 3. In both trials, chickens that were fed with rye had a significant increase in the number of total LAB that were observed in the duodenum, in the ileum, and in the ceca when compared with chickens fed with corn. In these chickens, a significant increase in the total number of coliforms was also observed in duodenum and ileum but not in cecum, whereas, an increase in total number of anaerobes was observed only in the duodenum.
The results of the evaluation of bone breaking strength and bone parameters in chickens fed with corn or rye in Experiments 1 and 2 are summarized in Table 4. Significant increases in tibia diameter, tibia breaking strength, tibia ash, and calcium and phosphorus percentages were observed in chickens that fed corn when compared with chickens that fed the rye diet (Table 4).
DISCUSSION
In recent years, nutrition research has moved from classical epidemiology and physiology to molecular biology and genetics. Modern nutritional research is aimed at health promotion, at disease prevention, and on performance improvement (Kussmann et al., 2008). Hence, nutritional sciences are discovering the application of the so-called “omics” sciences (Ghosh and Poisson, 2009; Dimitrov, 2011). Nutritional genomics is a recent off-shoot of this genetic revolution. As a consequence of these ambitious objectives, the disciplines “nutrigenetics” and “nutrigenomics” have evolved (Afman and Müller, 2006). in particular, nutrigenomics, is the junction between health, diet, and genomics, it can be seen as the combination of molecular nutrition and genomics, addressing the inverse relationship, which is how diet influences gene transcription, protein expression, and metabolism. (Davis and Milner, 2004; Trujillo et al., 2006; Wishart, 2008; García-Cañas et al., 2010). With emerging “omics” technologies, scientists are now better able to investigate how dietary food components can affect physiological functions and the underlying cellular and molecular mechanisms implicated in the digestive process (Ghosh and Poisson, 2009; Dimitrov, 2011). Nutrition-related genomics technology has revolutionized the field of nutrition providing unprecedented opportunities for increasing our understanding of how nutrients modulate gene and protein expression to influence cellular metabolism (Davis and Milner, 2004; Trujillo et al., 2006; Subbiah, 2007; Wishart, 2008; García-Cañas et al., 2010).
When integrated with other “omics” technologies in a biological system approach, novel nutrition-based intervention strategies are expected to provide an effective alternative for disease control.
Several studies in poultry have been conducted to investigate the effects of probiotics and phytonutrients on the translational regulation of genes associated with immunology, pathogen control, physiology, and metabolism using high-throughput microarray analysis and in vivo disease challenge models (Tellez et al., 1993; Higgins et al., 2007, 2008, 2010a,b, 2011; Timbermont et al., 2010; Lillehoj et al., 2011; Jerzsele et al., 2012; Kiarie et al., 2013; Layton et al., 2013). Chicken has been an important experimental system for developmental biology, immunology, and microbiology for more than 2 millennia (Stern, 2004) having led to many fundamental discoveries (Burt, 2007). With the evolution of sequencing of the genome and the development of high-throughput tools for the exploration of functional elements of the genome, chicken has attained a model organism status (Stern, 2005; Dodgson, 2007). The increase in genomic resources, easy access to the embryo, and the application of RNA interference showed that it will be easy and quick to use chicken embryos to screen the function of many genes during embryonic development (Stern, 2004). So, it seems likely that chicken will increasingly be the system of choice for many vertebrate biologists, especially in the field of human sciences, who are interested in gene function (Aggarwal and Lee, 2003; Hillier et al., 2004; Cogburn et al., 2007).
Thousands of years of evolution shaped the digestive system of the jungle fowl and wild pig to deal with the dietary ingredients they encounter in an efficient manner. More recently, throw intensive genetic manipulation, nutrition, and health programs we have altered the biology and growth potential of chickens and pigs among other productive animals (Muramatsu et al., 1990; Fuller et al., 1995). In the wild, the diets of these animals would be made up of many different ingredients, few of which would ever reach>30% of total intake on a lifetime basis. The range in types and relative quantities of ingredients that can be presented to the modem commercial monogastric animals, while complex, tend to result in a diet in which two or three ingredients may constitute >75% of intake (National Research Council, 1994). Such change is driven by least cost formulation processes, and endeavors to provide maximum nutrient density for minimum cost (Bedford and Schulze, 1998). Corn is usually the main source of energy in poultry diets, but at times it is difficult to formulate least-cost diets using corn and unconventional grains have to be used. When chickens are fed alternative grains such wheat or rye that are high in non-starch polysaccharides (NSPs), poor performance and unmanageable litter conditions caused by sticky droppings are reported (Campbell et al., 1983; Fengler and Marquardt, 1988; Choct et al., 1995). Wheat or rye contains high concentrations of NSPs, leading to reduced digestibility. In addition, high NSPs diets have also been associated with Necrotic Enteritis (NE), a multi-factorial disease caused by Clostridium perfringens (CP) that is probably the most important bacterial disease in terms of economic implications in broiler chickens (Hofacre, 2001; Annett et al., 2002; Timbermont et al., 2011). NSPs, are comprised mainly of highly branched arabinoxylans, increasing digesta viscosity responsible for poor digestibility through interference with the movement of particles and solutes across the intestinal lumen, preventing the access of digestive enzymes to the endosperm contents and reducing intestinal absorption of sodium and calcium (Fengler and Marquardt, 1988). In addition, increased digesta viscosity reduce conjugated bile acid, affecting fat emulsification and fat digestibility (Langhout et al., 1997). In the present study, the significant reduction in bone strength and mineralization (Table 4) confirmed previous studies that have shown that high NSPs diets in poultry or gluten intolerance in humans, are also associated with malabsorption of minerals and fat-soluble vitamins (MacAuliffe and McGinnis, 1971; Campbell et al., 1983; Rennie et al., 1993; Bianchi and Bardella, 2008; Capriles et al., 2009; Kotake et al., 2009; Wideman and Prisby, 2011). In our two experiments, the viscosity of the gut in chickens fed with rye was so extreme, the supernatant being more semi-solid than fluid, that it alone could be directly responsible for the poor performance (Table 2). Condensed feed passage rate increases the time available for digesta associated bacteria to multiply prior to evacuation in the feces, and provides more substrate availability in the distal parts of the small intestine for microbial fermentation (Choct et al., 1995; Murphy et al., 2009; Kiarie et al., 2013). In the present study, the increase intestinal viscosity observed in chickens fed with rye was also associated with elevated BT, increased serum FITC-d and bacterial overgrowth when compared with chickens fed with corn (Tables 2 and 3). Variations in the composition of the microbiome within different segments of the alimentary tract are influenced by the environment, by the diet, and by the host (Ouwehand et al., 2002; Xu and Gordon, 2003; Hooper, 2004; Mazmanian et al., 2008). Alterations in gut permeability are connected with BT in the portal and/or systemic circulation in several types of leaky gut syndromes leading to systemic bacterial infections (Ilan, 2012; Seki and Schnabl, 2012). Similarly, FITC-d is a large molecule (3–5 kDa) which does not usually leak through the intact gastrointestinal tract barrier. However, when conditions disrupt the tight junctions between epithelial cells, the FITC-d molecule can enter circulation as demonstrated by an increase in trans-mucosal permeability associated with chemically induced disruption of tight junctions by elevated serum levels of FITC-d after oral administration (Yan et al., 2009). Since poultry has little or no intrinsic enzymes capable of hydrolyzing these NSPs, exogenous xylanases as additives are used in an attempt to reduce this antinutritive factors (Bedford et al., 1991; Bedford and Classen, 1993; Bedford and Schulze, 1998). Previously, we have evaluated the inclusion of selected direct-fed microbial (DFM) candidates that produce exogenous enzymes (protease, phytase, lipase, xylanase, and cellulose) in high NSPs diets (rye, wheat, barley, and oat). In those studies, a significant reduction in both viscosity and C. perfringens proliferation was observed between high NSPs control non-treated diets or the same diets supplemented with DFM in vitro (Latorre et al., 2014; Tellez et al., 2014). The results of the present study have confirmed in some extent our earlier in vitro findings. Together, they represent a step toward the application of nutrigenomics in the context of a chicken model. The incorporation of one or more “omics” techniques (in particular, assessment of the microbiome) will provide a better understanding of how dietary food components can affect physiological functions and the fundamental cellular and molecular mechanisms implicated in the digestive process of high NSPs diets in chickens.
In conclusion, the use of rye as an energy source increased intestinal viscosity, increased BT, and leakage of FITC-d, altered the microbiota composition as well as bone mineralization in chickens. Studies to evaluate dietary inclusion of selected DFM candidates that produce exogenous enzymes in rye fed chickens are currently being evaluated.
ACKNOWLEDGMENTS
The authors gratefully acknowledge the excellent technical assistance of Scott Zornes (Poultry Production and Product Safety Research Unit, United States Department of Agriculture, Agricultural Research Service, Poultry Science Center, University of Arkansas, Fayetteville).
This article was originally published in Frontiers in Genetics, September 2014, Volume 5, Article 339, doi: 10.3389/fgene.2014.00339. This is an Open Access article distributed under the terms of the Creative Commons Attribution License (CC BY).
REFERENCES
Afman, L., and Müller, M. (2006). Nutrigenomics: from molecular nutrition to prevention of disease. J. Am. Diet. Assoc. 106, 569–576. doi: 10.1016/j.jada.2006.01.001
Aggarwal, K., and Lee, K. H. (2003). Functional genomics and proteomics as a foundation for systems biology. Brief. Funct. Genomic. Proteomic. 2, 175–184. doi: 10.1093/bfgp/2.3.175
Annett, C. B., Viste, J. R., Chirino-Trejo, M., Classen, H. L., Middleton, D. M., and Simko, E. (2002). Necrotic enteritis: effect of barley, wheat and corn diets on proliferation of Clostridium perfringens type A. Avian Pathol. 31, 598–601. doi: 10.1080/0307945021000024544
AOAC International. (2000). “Animal feeds,” in Official Methods of Analysis of AOAC International, 17th Edn., Vol. 1. ed. W. Horwaitz. (Gaithersburg, MD: AOAC International), 1–54.
Assimakopoulos, S. F., Papageorgiou, I., and Charonis, A. (2011). Enterocytes’ tight junctions: from molecules to diseases. World J. Gastrointest. Pathophysiol. 2, 123–137. doi: 10.4291/wjgp.v2.i6.123
Bedford, M. R., and Classen, H. L. (1993). An in vitro assay for prediction of broiler intestinal viscosity and growth when fed rye-based diets in the presence of exogenous enzymes. Poult. Sci. 72, 137–143. doi: 10.3382/ps.0720137
Bedford, M. R., Classen, H. L., and Campbell, G. L. (1991). The effect of pelleting, salt, and pentosanase on the viscosity of intestinal contents and the performance of broilers fed rye. Poult. Sci. 70, 1571–1577. doi: 10.3382/ps.0701571
Bedford, M. R., and Schulze, H. (1998). Exogenous enzymes for pigs and poultry. Nutr. Res. Rev. 11, 91–114. doi: 10.1079/NRR19980007
Bianchi, M. L. (2010). Inflammatory bowel diseases, celiac disease, and bone. Arch. Biochem. Biophys. 503, 54–65. doi: 10.1016/j.abb.2010.06.026
Bianchi, M. L., and Bardella, M. T. (2008). Bone in celiac disease. Osteoporos. Int. 19, 1705–1716. doi: 10.1007/s00198-008-0624-0
Burt, D. W. (2007). Emergence of the chicken as a model organism: implications for agriculture and biology. Poult. Sci. 86, 1460–1471. doi: 10.1093/ps/86.7.1460
Campbell, G. L., Campbell, L. D., and Classen, H. L. (1983). Utilisation of rye by chickens: effect of microbial status, diet gamma irradiation and sodium taurocholate supplementation. Br. Poult. Sci. 24, 191–203. doi: 10.1080/00071668308416730
Capriles, V. D., Martini, L. A., and Arêas, J. A. (2009). Metabolic osteopathy in celiac disease: importance of a gluten-free diet. Nutr. Rev. 67, 599–606. doi: 10.1111/j.1753-4887.2009.00232.x
Choct, M., Hughes, R. J., Trimble, R. P., Angkanaporn, K., and Annison, G. (1995). Non-starch polysaccharide-degrading enzymes increase the performance of broiler chickens fed wheat of low apparent metabolizable energy. J. Nutr. 125, 485–492.
Cogburn, L. A., Porter, T. E., Duclos, M. J., Simon, J., Burgess, S. C., Zhu, J. J., et al. (2007). Functional genomics of the chicken—a model organism. Poult. Sci. 86, 2059–2094. doi: 10.1093/ps/86.10.2059
Davis, C. D., and Milner, J. (2004). Frontiers in nutrigenomics, proteomics, metabolomics and cancer prevention. Mutat. Res. 551, 51–64. doi: 10.1016/j.mrfmmm.2004.01.012
Dimitrov, D. V. (2011). The human gutome: nutrigenomics of the host-microbiome interactions. OMICS 15, 419–430. doi: 10.1089/omi.2010.0109 Dodgson, J. B. (2007). The chicken genome: some good news and some bad news. Poult. Sci. 86, 1453–1459. doi: 10.1093/ps/86.7.1453
Dunsford, B. R., Knabe, D. A., and Haensly, W. E. (1989). Effect of dietary soybean meal on the microscopic anatomy of the small intestine in the early-weaned pig. J. Anim. Sci. 67, 1855–1863.
Elson, C. O., and Cong, Y. (2012). Host-microbiota interactions in inflammatory bowel disease. Gut Microbes 3, 332–344. doi: 10.4161/gmic.20228 Fengler, A. I., and Marquardt, R. R. (1988). Water soluble pentosans from rye. I. Isolation, partial purification, and characterization. Cereal Chem. 65, 291–297.
Fuller, M. F., Franklin, M. F., McWilliam, R., and Pennie, K. (1995). The responses of growing pigs, of different sex and genotype, to dietary energy and protein. Anim. Sci. 60, 291–298. doi: 10.1017/S1357729800008456
García-Cañas, V., Simó, C., León, C., and Cifuentes, A. (2010). Advances in Nutrigenomics research: novel and future analytical approaches to investigate the biological activity of natural compounds and food functions. J. Pharm. Biomed. Anal.51, 290–304. doi: 10.1016/j.jpba.2009.04.019
Ghosh, D., and Poisson, L. M. (2009). “Omics” data and levels of evidence for biomarker discovery. Genomics 93, 13–16. doi: 10.1016/j.ygeno.2008.07.006
Higgins, J., Higgins, S., Vicente, J., Wolfenden, A., Tellez, G., and Hargis, B. (2007). Temporal effects of lactic acid bacteria probiotic culture on Salmonella in neonatal broilers. Poult. Sci. 86, 1662–1666. doi: 10.1093/ps/86.8.1662
Higgins, J., Higgins, S.,Wolfenden, A., Henderson, S., Torres-Rodriguez, A., Vicente, J., et al. (2010a). Effect of lactic acid bacteria probiotic culture treatment timing on Salmonella enteritidis in neonatal broilers. Poult. Sci. 89, 243–247. doi: 10.3382/ps.2009-00436
Higgins, S. E., Ellestad, L. E., Trakooljul, N., McCarthy, F., Saliba, J., Cogburn, L. A., et al. (2010b). Transcriptional and pathway analysis in the hypothalamus of newly hatched chicks during fasting and delayed feeding. BMC Genomics 11:162. doi: 10.1186/1471-2164-11-162
Higgins, J. P., Andreatti Filho, R. L., Higgins, S. E., Wolfenden, A. D., Téllez, G., and Hargis, B. M. (2008). Evaluation of Salmonella-lytic properties of bacteriophages isolated from commercial broiler houses. Avian Dis. 52, 139–142. doi: 10.1637/8017-050807-ResNote
Higgins, S., Wolfenden, A., Tellez, G., Hargis, B., and Porter, T. (2011). Transcriptional profiling of cecal gene expression in probiotic-and Salmonella-challenged neonatal chicks. Poult. Sci. 90, 901–913. doi: 10.3382/ps.2010-00907
Hillier, L. W., Miller, W., Birney, E., Warren, W., Hardison, R. C., Ponting, C. P., et al. (2004). Sequence and comparative analysis of the chicken genome provide unique perspectives on vertebrate evolution. Nature 432, 695–716.
Hofacre, C. L. (2001). “Necrotic enteritis, currently a billion dollar disease: is there anything new on the horizon,” in Proceedings of Alltech’s 17th Annual Symposium Science and Technology in the Feed Industry, eds T. P. Lyons and K. A. Jacques (Nottingham: Nottingham University Press), 79–86.
Hooper, L. V. (2004). Bacterial contributions to mammalian gut development. Trends Microbiol. 12, 129–134. doi: 10.1016/j.tim.2004.01.001
Hrncir, T., Stepankova, R., Kozakova, H., Hudcovic, T., and Tlaskalova-Hogenova, H. (2008). Gut microbiota and lipopolysaccharide content of the diet influence development of regulatory T cells: studies in germ-free mice. BMC Immunol. 9:65. doi: 10.1186/1471-2172-9-65
Ilan, Y. (2012). Leaky gut and the liver: a role for bacterial translocation in nonalcoholic steatohepatitis. World J. Gastroenterol. 18, 2609–2618. doi: 10.3748/wjg.v18.i21.2609
James, S. P. (2005). Prototypic disorders of gastrointestinal mucosal immune function: Celiac disease and Crohn’s disease. J. Allergy Clin. Immunol. 115, 25–30. doi: 10.1016/j.jaci.2004.11.011
Jerzsele, A., Szeker, K., Csizinszky, R., Gere, E., Jakab, C., Mallo, J. J., et al. (2012). Efficacy of protected sodium butyrate, a protected blend of essential oils, their combination, and Bacillus amyloliquefaciens spore suspension against artificially induced necrotic enteritis in broilers. Poult. Sci. 91, 837–843. doi: 10.3382/ps.2011-01853
Kau, A. L., Ahern, P. P., Griffin, N. W., Goodman, A. L., and Gordon, J. I. (2011). Human nutrition, the gut microbiome and the immune system. Nature 474, 327–336. doi: 10.1038/nature10213 Kiarie, E., Romero, L. F., and Nyachoti, C. M. (2013). The role of added feed enzymes in promoting gut health in swine and poultry. Nutr. Res. Rev. 26, 71–88. doi: 10.1017/S0954422413000048
Kotake, S., Nanke, Y., Yago, T., Kawamoto, M., and Yamanaka, H. (2009). Human osteoclastogenic T cells and human osteoclastology. Arthritis Rheum. 60, 3158– 3163. doi: 10.1002/art.24886
Kussmann, M., Rezzi, S., and Daniel, H. (2008). Profiling techniques in nutrition and health research. Curr. Opin. Biotechnol. 19, 83–99. doi: 10.1016/j.copbio.2008.02.003
Kupfer, S. S., and Jabri, B. (2012). Pathophysiology of celiac disease. Gastrointest. Endosc. Clin. N. Am. 22, 639–660. doi: 10.1016/j.giec.2012.07.003
Langhout, D. J., Schutte, J. B., Geerse, C., Kies, A. E., De Jong, J., and Verstegen, M. W. (1997). Effects on chick performance and nutrient digestibility of an endoxylanase added to a wheatand rye-based diet in relation to fat source. Br. Poult. Sci. 38, 557–563. doi: 10.1080/00071669708418036
Latorre, J. D., Wolfenden, R., Vicente, J. L., Menconi, A., Wolfenden, A., Bielke, L. R., et al. (2014). “Evaluation of enzyme production, biofilm synthesis, viscosity, and germination/sporulation rate of a Bacillus spp. based commercial DFM product in different poultry diets using an in vitro digestive model,” in Proceedings of the International Poultry Scientific Forum, Atlanta.
Layton, S. L., Hernandez-Velasco, X., Chaitanya, S., Xavier, J., Menconi, A., Latorre, J. D., et al. (2013). The effect of a Lactobacillus-based probiotic for the control of necrotic enteritis in broilers. Food Nutr. Sci. 4, 1–7. doi: 10.4236/fns.2013.411A001
Leffler, D. (2011). Celiac disease diagnosis and management: a 46-year-old woman with anemia. JAMA 306, 1582–1592. doi: 10.1001/jama.2011.1312
Lillehoj, H. S., Kim, D. K., Bravo, D.M., and Lee, S. H. (2011). Effects of dietary plantderived phytonutrients on the genome-wide profiles and coccidiosis resistance in the broiler chickens. BMC Proc. 5(Suppl. 4):S34. doi: 10.1186/1753-6561- 5-S4-S34
MacAuliffe, T., and McGinnis, J. (1971). Effect of antibiotic supplements to diets containing rye on chick growth. Poult. Sci. 50, 1130–1134. doi: 10.3382/ps.0501130
Marsh, M. N. (1992). Gluten, major histocompatibility complex, and the small intestine. A molecular and immunobiologic approach to the spectrum of gluten sensitivity (“celiac sprue.”) Gastroenterology 102, 330–354.
Maslowski, K. M., and Mackay, C. R. (2011). Diet, gut microbiota and immune responses. Nat. Immunol. 12, 5–9. doi: 10.1038/ni0111-5
Mazmanian, S. K., Round, J. L., and Kasper, D. L. (2008). A microbial symbiosis factor prevents intestinal inflammatory disease. Nature 453, 620–625. doi: 10.1038/nature07008
Muramatsu, T., Hiratnoto, K., and Okumura, J. (1990). Strain differences in wholebody protein turnover in the chicken embryo. Br. Poult. Sci. 31, 91–99. doi: 10.1080/00071669008417234
Murphy, T. C., McCracken, J. K., McCann, M. E., George, J., and Bedford, M. R. (2009). Broiler performance and in vivo viscosity as influenced by a range of xylanases, varying in ability to effect wheat in vitro viscosity. Br. Poult. Sci. 50, 716–724. doi: 10.1080/00071660903389950
National Research Council. (1994). Nutrient Requirements of Poultry, 9th Revision Edn. Washington, DC: National Academic Press.
Ouwehand, A., Isolauri, E., and Salminen, S. (2002). The role of the intestinal microflora for the development of the immune system in early childhood. Eur. J. Nutr. 41, 132–137. doi: 10.1007/s00394-002-1105-4
Rennie, J. S., Whitehead, C. C., and Thorp, B. H. (1993). The effect of dietary 1, 25-dihydroxycholecalciferol in preventing tibial dyschondroplasia in broilers fed on diets imbalanced in calcium and phosphorus. Br. J. Nutr. 69, 809–816. doi: 10.1079/BJN19930081
Salminen, S., and Isolauri, E. (2006). Intestinal colonization, microbiota, and probiotics. J. Pediatr. 149, S115–S120. doi: 10.1016/j.mib.2010.09.018
Salzman, N. H. (2011). Microbiota-immune system interaction: an uneasy alliance. Curr. Opin. Microbiol. 14, 99–105. doi: 10.1016/j.mib.2010.09.018 SAS Institute. (2002). SAS User Guide. Version 9.1. Cary, NC:
SAS Institute Inc. Schuppan, D., Junker, Y., and Barisani, D. (2009). Celiac disease: from pathogenesis to novel therapies. Gastroenterology 137, 1912–1933. doi: 10.1053/j.gastro.2009.09.008
Seki, E., and Schnabl, B. (2012). Role of innate immunity and the microbiota in liver fibrosis: crosstalk between the liver and gut. J. Physiol. 590, 447–458. doi: 10.1113/jphysiol.2011.219691
Shirzadi, H., Moravej, H., and Shivazad, M. (2010). Influence of non starch polysaccharide-degrading enzymes on the meat yield and viscosity of jejunal digesta in broilers fed wheat/barley-based diet. Afr. J. Biotechnol. 9, 1517–1522.
Silva, M. A., Jury, J., Sanz, Y., Wiepjes, M., Huang, X., Murray, J. A., et al. (2012). Increased bacterial translocation in gluten-sensitive mice is independent of small intestinal paracellular permeability defect. Dig. Dis. Sci. 57, 38–47. doi: 10.1007/s10620-011-1847-z
Stepniak, D., and Koning, F. (2006). Celiac disease—sandwiched between innate and adaptive immunity. Hum. Immunol. 67, 460–468. doi: 10.1016/j.humimm.2006.03.011
Stern, C. D. (2004). The chick embryo-past, present and future as a model system in developmental biology. Mech. Dev. 121, 1011–1013. doi: 10.1016/j.mod.2004.06.009
Stern, C. D. (2005). The chick: a great model system becomes even greater. Dev. Cell 8, 9–17. doi: 10.1016/j.devcel.2004.11.018
Ströhle, A., Wolters, M., and Hahn, A. (2013). Celiac disease-the chameleon among the food intolerances. Med. Monatsschr. Pharm. 36, 369–80.
Subbiah, M. T. (2007). Nutrigenetics and nutraceuticals: the next wave riding on personalized medicine. Transl. Res. 149, 55–61. doi: 10.1016/j.trsl.2006.09.003
Tellez, G., Jaeger, L., Dean, C., Corrier, D., DeLoach, J., Williams, J., et al. (1993). Effect of prolonged administration of dietary capsaicin on Salmonella enteritidis infection in leghorn chicks. Avian Dis. 37, 143–148. doi: 10.2307/ 1591467
Tellez, G., Latorre, J. D., Wolfenden, R., Vicente, J. L., Menconi, A., Wolfenden, A., et al. (2014). “Screening of bacteriocin-like compound synthesis (BLC) from Bacillus spp: relation of diet composition, viscosity and proliferation of Clostridium perfringens in an in vitro digestive,” in Proceedings of the International Poultry Scientific Forum, Atlanta.
Timbermont, L., Haesebrouck, F., Ducatelle, R., and Van Immerseel, F. (2011). Necrotic enteritis in broilers: an updated review on the pathogenesis. Avian Pathol. 40, 341–347. doi: 10.1080/03079457.2011.590967
Timbermont, L., Lanckriet, A., Dewulf, J., Nollet, N., Schwarzer, K., Haesebrouck, F., et al. (2010). Control of Clostridium perfringens-induced necrotic enteritis in broilers by target-released butyric acid, fatty acids and essential oils. Avian Pathol. 39, 117–121. doi: 10.1080/03079451003610586
Trujillo, E., Davis, C., and Milner, J. (2006). Nutrigenomics, proteomics, metabolomics, and the practice of dietetics. J. Am. Diet. Assoc. 106, 403–413. doi: 10.1016/j.jada.2005.12.002
Wideman, R. F., and Prisby, R. D. (2011). Bone circulatory disturbances in the development of spontaneous bacterial chondronecrosis with osteomyelitis: a translational model for the pathogenesis of femoral head necrosis. Front. Endocrinol. 3:183. doi: 10.3389/fendo.2012.00183
Williamson, D., and Marsh, M. N. (2002). Celiac disease. Mol. Biotechnol. 22, 293–299. doi: 10.1385/MB:22:3:293
Wishart, D. S. (2008). Metabolomics: applications to food science and nutrition research. Trends Food Sci. Technol. 19, 482–493. doi: 10.1016/j.tifs.2008.03.003
Xu, J., and Gordon, J. I. (2003). Honor thy symbionts. Proc. Natl. Acad. Sci. U.S.A. 100, 10452–10459. doi: 10.1073/pnas.1734063100
Yan, Y., Kolachala, V., Dalmasso, G., Nguyen, H., Laroui, H., Sitaraman, S. V., et al. (2009). Temporal and spatial analysis of clinical and molecular parameters in dextran sodium sulfate induced colitis. PLoS ONE 4:e6073. doi: 10.1371/journal.pone.0006073
Zhang, B., and Coon, C. N. (1997). The relationship of various tibia bone measurements in hens. Poult. Sci. 76, 1698–1701. doi: 10.1093/ps/76.12.1698