INTRODUCTION
Cereal grains and vegetable protein sources contain between 10-75% of non-starch polysaccharides (NSP). NSP in cereals form part of the cell wall structure and in vegetable proteins, such as legumes, may also play a role as an energy storage material. As far as monogastric animal nutrition is concerned, NSP as the major part of fibre (fibre is the sum of NSP and lignin) are regarded as feed components that are either poorly digested or anti-nutritive. This is because (a) the soluble proportion of NSP elicits anti-nutritive activities, and (b) the utilisation of insoluble NSP by non-ruminant animals is very poor to zero. With the continuous increase in world's population and the decline in its food reserve, a more efficient conversion of by-products, including those rich in NSP, into high quality food will remain a top priority area of research in the future. Various biotechnologies, such as feed enzymes, will continually play a significant role in food production through improving the use of poorly digested nutrients for animal production. These technologies have important implications for combating climate change because improved feed efficiency means "producing more from less" as well as alleviating pollution problems arising from an excessive output of excreta containing large amounts of organic matter. The enzyme technology, for instance, has been applied in practice for more than two decades with outstanding results. However, the potential for the technology is far from exhausted. In fact, even enzymes, such as carbohydrases, which have been studied extensively, have a long way to go when it comes to increasing the utilisation of insoluble NSP as energy sources. The current paper presents data on the chemical characteristics of various NSP found in feed ingredients and focuses on carbohydrases used in monogastric diets.
FEED CARBOHYDRATES
Classification
Carbohydrates are the main components of animal feed, with starch being the major constituent. Starch is second only to cellulose in abundance in terms of polysaccharides synthesised by plants and represents the primary source of energy for many monogastric species, including humans. The other part of feed carbohydrates consists of NSP and free sugars (mon-, di- and oligosaccharides).
Polysaccharides are polymers of monosaccharides joined through glycosidic linkages and are defined and classified in terms of the following structural considerations: (a) identity of the monosaccharides present; (b) monosaccharide ring forms (6-membered pyranose or 5-membered furanose); (c) positions of the glycosidic linkages; (d) configurations (or) of the glycosidic linkages; (e) sequence of monosaccharide residues in the chain, and (f) presence or absence of non-carbohydrate substituents. Monosaccharides commonly present in plant carbohydrates are: (a) hexoses; D-glucose, D-galactose, D-mannose; (b) pentoses; L-arabinose, D-xylose, and (c) acidic sugars; D-galacturonic acid, D-glucuronic acid and its 4-O-methyl ether. NSP cover a large variety of polysaccharide molecules. The classification of NSP was based originally on the methodology used for extraction and isolation of polysaccharides. The residue remaining after a series of alkaline extractions of cell wall materials was called cellulose, and the fraction of this residue solubilised by alkali was called hemicellulose. The word hemicellulose was adopted because early researchers mistakenly regarded these polysaccharides as the precursors of cellulose. This is now known to be incorrect but the term is still commonly used. Some researchers used the terms hemicelluloses and pentosans interchangeably because the pentose-containing polysaccharides make up the bulk of hemicelluloses (Neukom et al., 1967; Neukom, 1976). Classification by differences in solubility lacks precision with respect to both chemical structures and biological functions. For example, the term crude fibre (CF) refers to the remnants of plant material after extraction with acid and alkali and includes variable portions of the insoluble NSP. Neutral detergent fibre (NDF) refers to the insoluble portion of the NSP plus lignin, and acid detergent fibre (ADF) refers to a portion of insoluble NSP comprised largely, but not exclusively, of cellulose and lignin. The nutritional relevance of values obtained using these methods in monogastric nutrition therefore is questionable. The complexity in the structure and confusion in the nomenclature have made it almost impossible to draw a clear-cut classification of NSP. However, from a chemistry point of view, NSP fall into three main groups as shown below, namely cellulose, non-cellulosic polymers and pectic polysaccharides (Bailey, 1973).
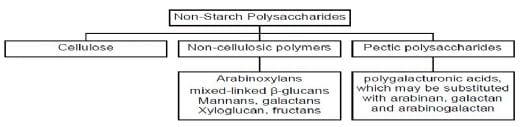
Physiochemical and Nutritional Properties
From the physical characteristics, NSP are classified according to their solubility in various solvents. In the context of feed ingredients, water solubility is often used as the measure because soluble and insoluble NSP behave very different in the gut, leading to contracting nutritional outcomes in monogastric animals. There have been numerous reports on the anti-nutritive effects of soluble NSP on nutrient digestibility and absorption in poultry (Choct and Annison, 1992; Choct 2006). These effects are due to the ability of soluble NSP to increase digesta viscosity, which has knock-on effects on gut development and microflora. The negative correlation between NSP content of the diet and its nutritive value has been clearly demonstrated in poultry (Choct and Annison, 1990; Annison, 1991), in pigs (King and Taverner, 1975) and in pets (dogs and cats) (Earle et al., 1998). It is understood that the viscous nature of NSP depends on the solubility and molecular weights of the NSP. Solubility of NSP, in turn, depends on their chemical structures and association with the rest of the cell wall components. Viscosity, however, is not specific to the sugar composition or linkage types present in the NSP. Furthermore, the physical effect of viscosity on nutrient digestion and absorption is similar regardless of the NSP sources. Generally, high gut viscosity decreases the rate of diffusion of substrates and digestive enzymes and hinders their effective interaction at the mucosal surface (Edwards et al., 1988; Ikegami et al., 1990). Soluble NSP interact with the glycocalyx of the intestinal brush border and thicken the rate-limiting unstirred water layer of the mucosa, which reduces the efficiency of nutrient absorption through the intestinal wall (Johnson and Gee 1981). As physiological and microbial changes occur, the efficiency of nutrition digestion, transport and absorption are altered. For example, since viscosity slows down digesta transit rate and reduces nutrient digestibility, more undigested nutrients remain in the gut for a longer period of time, more mucous is secreted due to stimulation of the mucosa by microbes (Chee et al., 2010), and more fermentation occurs in the gut due to a change in oxygen tension in the small intestine (Choct et al., 1996).
Insoluble NSP make up the bulk of the total fibre in diets, but they have little or no effect on nutrient utilisation in monogastric animals (Begin, 1961). The insoluble NSP, however, are not inert and their roles in monogastric nutrition cannot be neglected. One of the most important attributes of insoluble NSP is their ability to absorb large amounts of water and maintain normal motility of the gut (Stephen and Cummings, 1979). This is essential for the consistency of the excreta in monogastric animals. Elevated levels of insoluble fibre in diet shorten the residence time of digesta (Kirwan et al., 1974) and some argue that this may result in low nutrient digestibility. The rationale is that the longer the feed is exposed to the digestive processes in the gut, the more complete its digestion. This is proven incorrect as addition of insoluble fibre sources to low energy wheat increased its metabolisable energy value for poultry (Rogel, 1985). In recent years, there is increasing evidence to suggest that coarse, structural materials consisting largely of insoluble fibre enhances gut development in poultry (Choct, 2006; Hetland et al., 2007).
Chemical Composition and Structure
Cereal Grains
In regards to their use in non-ruminant animal feed, cereal grains are classified into two categories, i.e., viscous and non-viscous grains. This classification is based on characteristics of the soluble NSP present in the grain and their ability to form viscous digesta. Supplementation of diets containing high levels of viscous grains with appropriate carbohydrases has been proven much more effective than those containing non viscous grains. However, in both types of grains starch makes up 60-75% of the carbohydrates. The starches of cereal grains are highly digestible by the amylolytic enzymes of monogastric animals as they contain very little resistant starch. The properties of starch from normal cereals and high-amylose maize are compared in Table 1. The cereal starches have a smaller granular size than that of other sources and normally contain 20-35% amylase and 65-80% amylopectin. It is well-established that starches high in amylose are not effectively digested by amylolytic enzymes and consequently act like fibre in the small intestine and are not well utilised by monogastric animals (Annison and Topping, 1994).
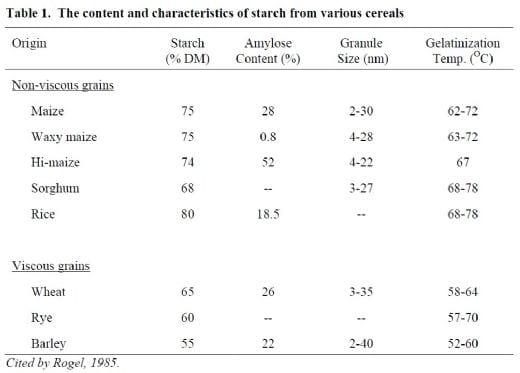
Apart from starch, cereal grains contain between 10-30% NSP (xylans, ß-glucans and cellulose). Only small amounts of pectic polysaccharides are found in the stems and leaves of cereals. Corn and sorghum contain very low levels of NSP, in particular the soluble fraction, whereas wheat, rye and triticale contain substantial amounts of both soluble and insoluble NSP. The main soluble NSP in these grains are arabinoxylans, whereas in barley and oats they are ß-glucans. The types and levels of NSP in cereals are shown in Table 2.
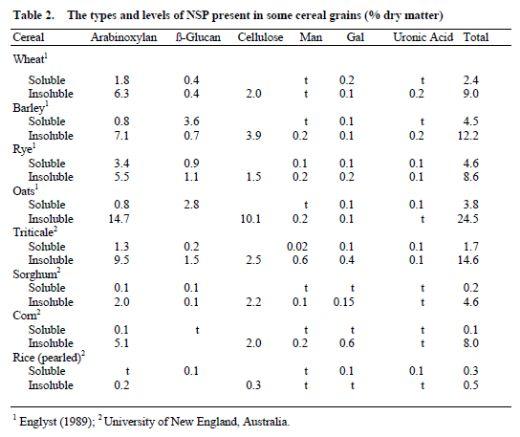
Cereal By-Products
As shown in Table 2, both viscous and non-viscous grains contain considerable amounts of insoluble NSP. These NSP often end up by-products, such as brans and Distillers Dried Grains with Solubles (DDGS). For instance, wheat bran contains approximately 40% NSP which are predominantly arabinoxylans (Englyst, 1989). Wheat pollard, on the other hand, has slightly lower (34%) of NSP and still lower for wheat germ (17%). In a good quality rice bran, the NSP content rarely exceeds 25%, which consist of approximately equal amounts of arabinoxylans and cellulose (Saunders, 1986). The NSP in cereal by-products are largely insoluble, although wheat bran contains up to 3% of soluble NSP. It appears that the NSP in cereal by-products are also of low molecular weight and even when they are solublised, will not form highly viscous gel (Annison et al., 1996). The quality and quantity of NSP in wheat bran, wheat pollard and rice bran are shown in Table 3.
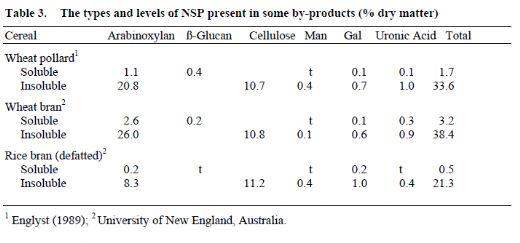
In addition to arabinoxylans and cellulose, rice bran also contains an unusual group of NSP, xyloglucans (Shibuya and Misaki, 1978). The structure of xyloglucans is a ß 1-4-linked glucan backbone with single units of α-xylose attached to the O6 atoms of the main chain. The physicochemical properties and nutritional activities of these NSP are yet to be established. In recent years, a by-product from cereals, namely the DDGS, has become widely available due to the use of various cereal grains for ethanol production. Choct and Petersen (2009) presented the chemical composition of six corn DDGS samples obtained from the northern part of the USA. In terms of the carbohydrate composition, the samples contained, on as is basis, between 15.5% to 22.4% starch, 14.6% to 19.3% NSP and 1.4% to 8.3% free sugars (sugars extractable in 70% ethanol). There is variation in sorghum DDGS produced in Australia (Choct et al., unpublished data). Cellulose and mannans have no clear anti-nutritive effects on nutrient digestion and absorption except that it reduces nutrient density of the diet.
Vegetable protein sources
Most vegetable proteins are usually high in NSP, with leguminous sources containing NSP dominated by pectic polysaccharides. The term pectic polysaccharides refers to galacturonans or, more commonly, rhamnogalacturonans, in which (1-4)-α-D-galacturonan chains are interrupted at intervals by insertion of (1-2)-α-L-rhamnose residues. Other constituent sugars attached as side chains include D-galactose, L-arabinose, D-xylose, and less frequently L-fucose and D-glucuronic acid. Most of these sugars occur in short side chains, although D-galactose and L-arabinose are often found in multiple units. Extremely complicated side chains containing neutral pectic polymers such as galactans and arabinans, xyloglucans and galactomannans have been reported. The molecular weights of the pectins have been reported to range from 30,000 to 300,000 (Pilnik and Voragen, 1970). In soybean, the L-rhamnose residues in the rhamnogalacturonan backbone have been shown to be joined consecutively and others alternatively with galacturonic acid residues (Aspinall et al., 1967). The carboxyl groups of the galacturonic acid residues of the pectic polymers can be highly methyl esterified (Aspinal and Jiang, 1974), depending on their sources. A detailed breakdown of carbohydrates present in soybean and soybean meal products is given by Choct et al. (2010). The soluble pectins from mung beans, for instance, are highly methylated (Goldberg et al., 1994). Pectins with high degree of esterification are not susceptible to endo-polygalacturonase which requires at least two free carboxyl groups adjacent to each other (Jansen and MacDonnell, 1945). The structure of a pectic polymer from lupins by Cheethan et al. (1993) is shown below:
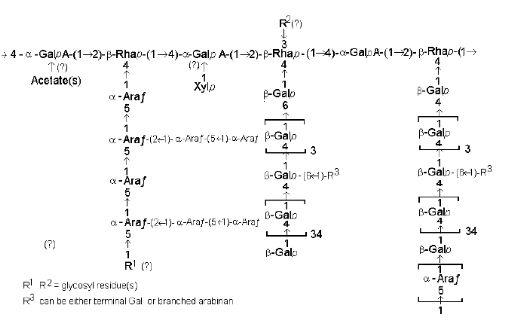
CARBOHYDRATE-DEGRADING ENZYMES
Enzymes Targeting Cereal Grains
The fact that the viscous property of NSP is a major factor in the anti-nutritive effect of NSP in monogastric diets is supported by the wide-spread use of enzymes in monogastric diets. The enzymes cleave the large molecules of NSP into smaller polymers, thereby reducing the thickness of the gut content and increasing the nutritive value of the feed (Bedford et al., 1991; Choct and Annison, 1992).
Feed Enzymes have come a long way since the very first use of enzymes in feed by Clickner and Follwell in 1925. The two key groups of enzymes are carbohydrases and phytases, with the former consisting mainly of β-glucanase and xylanase that target soluble NSP, whereas the latter is used to release phosphorus from phytate. Both types of enzymes function by countering the negative impact of anti-nutrients, in the meantime releasing nutrients from cell wall matrices. As phytase is not within the scope of this paper, the use of carbohydrases in monogastric diets will be detailed based on the grain type.
It is well accepted that the positive effect of carbohydrases in viscous grains is not due to a total depolymerisation of the NSP into simple sugars, but rather is due to the ability of these enzymes to degrade them into smaller polymers, rendering them unable to form viscous digesta. Since viscosity is not a unique characteristic of any specific molecule, it is essential that carbohydrases target their substrates in order to degrade the specific viscosity-inducing molecules originating from different ingredients.
Barley and Oats
Barley and oats contain a high level of mixed-linked β-glucan (3-4%). The poor nutritive value of barley in chickens is related to its β-glucan level (Burnett, 1966). Since this significant finding, there have been numerous studies on the use of enzymes, in particular β-glucanases in barley and oat based poultry diets (Hesselman and Åman, 1986; Campbell et al., 1989), with outstanding success. Barley also contains an appreciable amount of soluble NSP other than β-glucans and thus the majority of enzymes for barley diets have both ß-glucanase and xylanase activities. ß-Glucans are glucose polymers containing a mixture of ß1-3 and ß1-4 linkages that make their physicochemical properties totally different from cellulose that is a straight-chain glucose polymer with only ß1-4 linkages. A typical structure of ß-glucans is shown below:
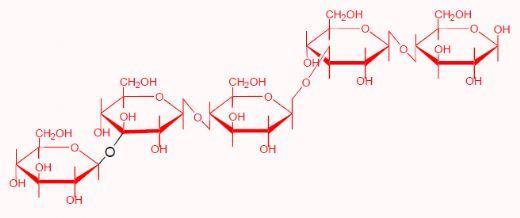
Wheat, rye and triticale
The common NSP in rye, wheat and triticale that exhibit anti-nutritive activities in monogastric animal diets are arabinoxylans. Cereal arabinoxylans are composed predominantly of two pentoses, arabinose and xylose, and their molecular structure consists of a linear (1-4)-ß-xylan backbone to which substituents are attached through O2 and O3 atoms of the xylosyl residues (Perlin, 1951). Most of the arabinoxylans in cereal grains are insoluble but the arabinoxylans not bound to the cell walls can form highly viscous solutions and can absorb about ten times their weight of water. A likely structure of the arabinoxylan is presented below:
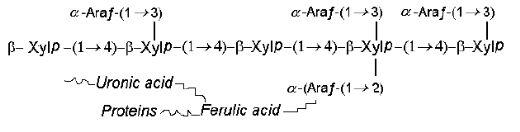
Thus, the addition of xylanases to rye-based broiler diets significantly improves the growth performance and feed conversion efficiency (Bedford et al., 1991; Bedford and Classen, 1992). Supplementation with increasing levels (0.11, 0.22, 0.44, and 0.88 g/kg) of an enzyme preparation having pentosanase and ß-glucanase activities to a rye-wheat based diet improved the weight gain of broilers up to 27% and FCE up to 10% (Pettersson and Åman, 1988;1989). Although enzymes always substantially improve the performance of birds fed rye diets, they do not seem to have as large an effect on the litter quality. In wheat, the level of NSP change due to environment is inversely related to the metabolisable energy (ME) value in poultry (Annison, 1991). Variation of up to 4 MJ in ME has been reported for wheat (Choct et al., 1995). Supplementation of a low-ME wheat diet with a commercial xylanase preparation increased the ME by 24% and the feed to gain ratio by 25% in 3-4 week old broiler chickens. There are fewer data on use of enzymes in triticale-based diets. Tran (1996) reported a significant improvement in ME of broiler chicken diets containing 20-40% triticale with use of a xylanase. Triticale contains almost equal amounts of arabinoxylans and ß-glucans and it is expected that an enzyme cocktail containing xylanase and ß-glucanase activities will be more efficacious in triticale based rations.
Corn and sorghum
Corn and sorghum together with millet are "non-viscous" grains, reflecting the fact that these grains contain negligible amounts of soluble NSP and therefore do not produce viscous digesta when fed to pigs and poultry. Enzymes targeting diets based on these cereals have been less effective compared with those based on viscous grains.
Enzymes Targeting Cereal By-Products
Cereal by-products are used extensively in animal feed although their inclusion levels in monogastric diets are limited below 10% due to their high NSP content. The major cereal by-products used as monogastric feed are millrun (wheat bran + pollard) and rice bran in most countries around the world, with the availability of one or the other depending on the geographical location. For example, rice bran is not always available in North America, Australia and New Zealand, but it is available in large quantities in Asia throughout the year.
Various enzymes, including xylanase, ß-glucanase and cellulose, have been used to enhance the nutritive value of cereal by-products for pigs and poultry (Choct, 2006). Although it appears that some improvement in the nutritive value of diets rich in cereal by-product can be attained through the use of enzymes, there is no conclusive evidence that supplemental carbohydrases are capable of depolymerising insoluble NSP, such as cellulose and mannans, into their monomeric constituents within the food transit time of monogastric animals.
There have been numerous reports on the nutritive value of DDGS (Shurson et al., 2004; Ward et al., 2008). There is tremendous variability in nutrient and energy utilisation of DDGS, as summarised by Péron and Partridge (2009). As presented earlier, corn DDGS contains up to 40% carbohydrates which are equally divided into NSP and starch (Choct and Petersen, 2009). Recent studies reflect the fact that the use of enzyme cocktails, such as those consisting of xylanase, amylase, protease and phytase, in diets containing high levels of DDGS can enhance nutrient digestibility and performance in broilers (Ledoux et al., 2009) and in pigs (Emiola et al., 2009). Again, the stumbling block in finding highly effective enzymes for DDGS will be the ability of the enzymes to degrade insoluble NSP, such as cellulose and insoluble arabinoxylans, within the food transit time of the animal.
Enzymes Targeting Vegetable Proteins
Despite a number of studies reporting positive effects of carbohydrases on the vegetable protein components of monogastric diets (Kocher et al. 2000, 2002), there appear to be no commercial enzyme products available to date for effective depolymerisation of the complex polysaccharides in vegetable protein sources, such as legumes, within the digesta transit time of the monogastric animals. Similarly, a considerable number of studies on the use of oligosaccharide-degrading enzymes (α-galactosidases) have been reported (Brenes et al., 1993; Bryden et al., 1994; Irish et al., 1995), but the results are ambiguous.
Enzymes Targeting Non-Conventional Ingredients
The by-products of roots and tubers, of vegetable oil sources such as coconut, sunflower, safflower, groundnut (peanut), palm kernel, and of rubber processing are used in various countries as feed resources. Despite the obvious advantages of using cheaper, alternative feedstuffs in monogastric diets when available, such raw materials may have some negative effects on performance. The quality of these materials may be highly variable, especially in their nutritional composition and some raw materials differ widely in their amino acid composition even when protein levels are the same. New non-conventional feedstuffs can be and are included in formulated feed, although the necessary information of nutritional composition and deleterious factors may not be clearly understood. The use of these materials may therefore produce unpredictable, often negative effects, on performance either in terms of nutrient digestion or in terms of anti-nutritive effects.
The quality of by-products is influenced by processing. Two of the amino acids most adversely affected by processing conditions are lysine and cystine. Too high or prolonged heat during the extraction process will destroy essential amino acids in protein meals. Miller (1976) indicated that the proteins in oilseed meals are particularly susceptible to damage by heat treatments involved in the oil extraction because of the high carbohydrate concentration of the oilseeds. However, the correct heat processing of oilseeds makes nutrients highly available and destroys anti-nutritional factors (Göhl, 1981; Kalinowski, 1993). Anti-nutritional factors contained in alternative protein meals will impart negative effects on the performance of animals, even though some efforts have been made to reduce or eliminate these factors (Cheeke and Shull, 1985). Most alternative ingredients contain high levels of NSP, which are the most important limitation to their effective use in monogastric diets. Many other factors, including mould contamination and associated toxins, can impair the nutritional value of alternative protein sources, interfering with the utilisation of feed ingredients for animals, but this is not within the scope of this paper.
Copra meal
Copra meal is the residue of coconut oil production. Despite its 20% crude protein, the use of copra meal in monogastric diets is limited due to a very high level of NSP (Purwadaria et al., 1995). The NSP make up between 45-60% of the dry matter and consist predominantly of mannans (galactomannans and mannans), just over 10% cellulose and trace amounts of other polymers (arabinoxylogalactans, arabinomannogalactan and galactoglucomannans) (Saittagaroon et al., 1983; Zamora et al., 1989). Saittagaroon et al. (1983) reported that approximately 30% of the copra NSP is soluble in hot water, but their nutritional properties are yet to be defined. The glucomannans are comprised of (1-4)-ß-linked glucose and mannose units, whilst the galactomannans consist of a (1-4)-ß-mannan backbone substituted with single units of (1-6)-α-galactose. Information on use of enzymes in diets containing copra meal is scarce. Purwadaria et al. (1995) reported an increased fibre digestibility in vitro after incubation of copra meal with Aspergillus niger.
Palm kernels
Palm kernel meal is a common feed ingredient in Asian feed mills. The kernel contains approximately 50% oil (Omar and Hamdan, 1998) and around 70-80% of the oil is removed by extraction. The by-product of oil extraction is known either as palm kernel cake or as palm kernel meal, depending on the processing method. Expeller extraction method produces the former, whereas solvent extraction produces the latter. The two types of products have different chemical composition, such as calcium, oil and fibre. Omar and Hamdan (1998) reported oil and crude fibre levels of 12.3% and 9.8% for the cake and 16.6% and 5.2% for the meal, respectively. The commercial palm kernel meal contains about 16% of crude protein and at least 60% of NSP. An NSP content as high as 74.3% has been reported for palm kernel cake (Omar and Hamdan, 1998). The NSP are believed to be insoluble linear mannans (78% of total NSP) with very low galactose substitution, followed by cellulose (12%) and a small amount of 4-O-methyl-glucuronoxylans (3%) and arabinoxylans (3%) (Düsterhöft et al., 1992). Supplementation of commercially available glycanases to palm kernel containing diets yielded inconsistent results (Daud et al., 1997). A typical structure of a 1-4- ß-mannan is shown below:
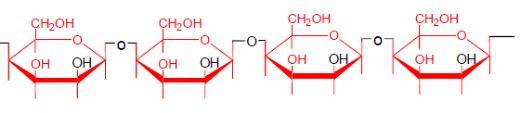
Peanut Meal
The peanut (Arachis hpogaea) is a tropical legume that is also known as the groundnut, earthnut, monkey nut, Manilla nut, Chinese nut, pindar or goober pea (Göhl 1981; Evans, 1985). Peanut is grown for the confectionery trade and, periodically, peanuts are available for stockfeed (Evans, 1985). Peanuts are also crushed for oil and the subsequent by-product meal is then available as a stockfeed, as are the shells. A large increase in peanut production is expected for China, India and Indonesia over the next five years.
Peanut meal is the by-product of the oil-extraction process and is a source of highly concentrated protein for animal feed. Peanut meal contains 42-47% protein which is deficient in sulphur amino acids and lysine (Miller, 1976; Evans, 1985). The quality of peanut meal is influenced by the quantity of hulls in the meal and by the type and level of heat during processing. Processing factors, such as heat treatment, moisture level and extraction method, are also involved. Impaired digestibility and availability of protein meals may result from the presence of high fibre content, anti-nutritional factors or from damage during processing. The high NSP content of peanut meal makes it particularly susceptible to damage by the heat treatments involved in the oil extraction (Miller, 1976). The carbohydrate composition of peanut meal is not known. Evans (1985) reported a crude fibre content of 8.7% for peanut meal, which could represent up to 30% of NSP. There are also not data on use of enzymes in diets containing peanut meal.
Sunflower meal
Irish and Balnave (1993) reported 27.6% NSP in sunflower seeds, of which 4.5% was soluble. The soluble NSP were mainly the uronic acids. Assuming the rate of oil extraction at 40%, the amount of NSP in the meal could be as high as 46%. The NSP consist of 42% cellulose, 24% pectic polysaccharides, 24% 4-O-methyl-glucuronoxylans, 5% (gluco)-mannans and 4.5% fucoxyloglucans (Düsterhöft et al., 1992). Supplementation of diets containing high levels of sunflower meal with a number of commercial enzymes had little or no effects on performance of broiler chickens (Gerendai et al., 1997; El Sherif et al., 1997).
Roots and tubers
The term roots and tubers refers to potatoes, sweet potatoes, cassava, yams, taro (cocoyam) and other root plants. These are not only important human foods; they are also very significant feed sources for animals. Most of the roots and tubers are used as alternative energy sources for pigs and poultry. Cassava, also known as tapioca, manioc and mandioca contains approximately 87% starch and 10-15% fibre. Sometimes a by-product of cassava starch extraction is also available for use as a feed ingredient. It is called onggok in Indonesia and contains 15-20% starch and a very high level of NSP. The nature of the NSP is not known but it is likely that the NSP consist of cellulose, arabinoxylans and some pectic polysaccharides.
The quality of root and tuber starch products varies widely, with some products containing up to 20% NSP. Occasional occurrence of increased concentration of cyanide in some products is also known, although detrimental levels are not reported. The starches from potatoes and cassava contain 20% and 16.7% amylose and have gelatinisation temperatures between 50-68oC and 49-65oC, respectively (Rogel, 1985). Our recent in-house (at the University of New England, Australia) analysis of several cassava samples showed that a typical sample contains around 75% starch, which is highly digestible by amylase together with amyloglucosidase, and has an amylase to amylopectin ratio of 23:77. The level of NSP is around 5%, with only 10% soluble but not viscosity forming. It contains about 2% free sugars, consisting mainly of glucose, galactose and rhamnose.
CONCLUSION
Starch and NSP are the main carbohydrates in conventional and non-conventional feeds, although small amounts of free sugars (oligosaccharides and monosaccharides) are also present. These carbohydrates are the sources of most of the dietary energy as well as the fibre.
NSP of various ingredients vary widely in terms of chemical structures and physiological characteristics, and for many non-conventional ingredients, basic quantitative information on the type of sugars making up the NSP is lacking. For cereal grains and their by-products, not only is quantitative information available on levels of soluble and insoluble NSP, the chemical structures of these polymers are also well defined. The anti-nutritive activities of soluble NSP in monogastric animals are well elucidated and microbial enzymes are routinely used to alleviate their effects on nutrient digestion and absorption. However, whilst the current enzyme technology is quite effective against soluble NSP with well-characterised chemical structures, it is not nearly as effective against insoluble NSP or NSP with highly complex structures, such as pectins. As more and more knowledge is gathered on the detailed chemical structures and the physiological activities of NSP in various ingredients, more sophisticated enzymes will be developed to target these polymers in a precise manner. The future challenge for the feed industry is, therefore, to produce highly efficacious enzymes which will lead to the utilisation of both soluble and insoluble NSP as an energy source for monogastric animals.
REFERENCES
Annison, G. (1991). J. Agric. Food. Chem. 39: 1252.
Annison, G. and Topping, D.L. (1994). Ann. Rev. Nutr. 14: 297.
Annison, G., Hughes, R.J. and Choct, M. (1996). Br. Poult. Sci. 37: 157.
Aspinall, G.O. and Jiang, K.-S. (1974). Carbohydr. Res. 38: 247.
Aspinall, G.O., Cottrell, I.W., Egan, S.V., Morrison, I.M. and Whyte, J.N.C. (1967). J. Chem. Soc. C: 1071.
Bailey, R.W. (1973). In "Chemistry and Biochemistry of Herbage", Vol.1, P.157.
Bedford, M. R. and Classen, H. L. (1992). J. Nutr. 122: 560.
Bedford, M.R. and Morgan, A.J. (1995). Proc. 2nd Euro. Symp. Feed Enzymes. Eds: W. van Hartingsveldt, M. Hessing, J.P. van der Lugt and W.A.C. Somers. P.125, Zeist, the Netherlands.
Bedford, M.R., Classen, H.L. and Campbell, G.L. (1991). Poult.Sci. 70: 1571.
Begin, J.J. (1961). Poult.Sci. 40: 892.
Brenes, A., Marquardt, R. R., Guenter, W. and Rotter, B. A. (1993). Poult. Sci. 72: 2281.
Broz, J. and Firgg, M. (1986). Archiv Für Geflügelkunde 50: 41.
Bryden, W. L., Gill, R. J. and Balnave, D. (1994). Aust. Poult. Sci. Symp. 6: 115.
Burger, I. (1993). The Waltham Book of Companion Animal Nutrition. Ed.: I. Burger, Pergamon Press.
Burnett, G. S. (1966). Br. Poult. Sci. 7: 55.
Campbell, G. L., Rossnagel, B. F., Classen, H. L. and Thacker, P. A. (1989) Anim.l Feed Sci. Technol. 26: 221.
Chee, S.H., Iji, P.A., Choct, M., Mikkelsen, L.L. and Kocher A. (2010). Br. Poult. Sci. 51:368.
Cheeke, P.R. and Shull, L.R. (1985). In: "Natural Toxicants in Feeds and Poisonous Plants", AVI Publishing Co., Inc., Connecticut.
Cheetham, N. W. H., Cheung, P. C. K. and Evans, A. J. (1993). Carbohydr. Polym. 22: 37.
Choct, M. (2006). World's Poult. Sci. J. 62: 2.
Choct, M. and Annison, G. (1992). Br. Poult. Sci. 33: 821.
Choct, M. and Annison, G. (1990). Br. Poult. Sci. 31:811.
Choct, M. and Petersen, S.T. (2009). Aust. Poult. Sci. Symp. 20: 117.
Choct, M., Hughes, R.J., Trimble, R.P., Angkanaporn, K. and Annison, G. (1995). J. Nutr. 125: 485.
Choct, M., Hughes, R.J., Wang, J., Bedford, M.R., Morgan, A.J. and Annison, G. (1996). Br. Poult. Sci. 37: 609.
Choct, M. Dersjant-Li, Y., McLeish, J. and Peisker, M. (2010). Asian-Aust. J. Anim. Sci. 23: 1386.
Clicker, F.H. and Follwell, E.H. (1925). Poult. Sci. 5: 241.
Daud, M.J., Samad, N. and Rasool, S. (1997). Proc. 19th MSAP Conf., Johor Bharu, Malaysia.
Düsterhöft, E.-M., Posthumus, M. A. and Voragen, A. G. J. (1992). J. Sci. Food Agric. 59: 151.
Earle, K.E., Opitz, B., Kienzle, E., Smith, P.M. and Maskell, I.E. (1998). www.waltham.com/vets/pubs/vp02_14.htm
Edwards, C. A., Johnson, I. T. and Read, N. W. (1988). Euro. J. Clin. Nutr. 42: 306.
El Sherif, Kh., Gerendai, D. and Gippert, T. (1997). Aust. Poult. Sci. Symp. 9: 195.
Emiola, I.A.,Opapeju, F.O., Slominski, B.A. and Nyachoti, C.M. (2009). J. Anim. Sci. 87: 2315.
Englyst, H. (1989). Anim. Feed Sci. Technol. 23: 27.
Evans, M. (1985). Nutrient Composition of Feedstuffs for Pigs and Poultry, Queensland Department of Primary Industries, Brisbane.
Gerendai, D., El Sherif, Kh. and Gippert, T. (1997). Aust. Poult. Sci. Symp. 9: 211.
Göhl, B. (1981). In: "FAO Animal Production and Health Series", No. 12, Food and Agriculture Organization of the United Nations, Rome.
Goldberg, R., Prat, R. and Morvan, C. (1994). Carbohydr. Polym. 23: 203.
Hesselman, K. and Åman, P. (1986). Anim.l Feed Sci. Technol. 15: 83.
Hetland, H,, Uhlen, A.K., Viken, K.H., Krekling, T. and Svihus, B. (2007). Br. Poult. Sci. 48: 12.
Ikegami, S., Tsuchihashi, F., Harada, H., Tsuchihashi, N., Nishide, E. and Innami, S. (1990). J. Nutr. 120: 353.
Irish, G. G., Barbour, G. W., Classen, H. L., Tyler, R. T. and Bedford, M. R. (1995) Poult. Sci. 74: 1484.
Irish, G.G. and Balnave, D. (1993). Aust. J. Agric. Res. 44: 1483.
Jansen, E.F. and MacDonell, L.R. (1945). rch. Biochem. 8: 97.
Johnson, I.T. and Gee, J.M. (1981). Gut, 22: 398.
Kalinowski, P. (1993). Proc. Queensland Poult. Sci. Symp., University of Queensland, Brisbane.
King, R.H. and Taverner, M.R. (2975). Anim. Prod. 21: 275.
Kirwan, W.O., Smith, A.N., McConnel, A.A., Mitchell, W.D. and Eastwood, M.A. (1974). Br. Med. J. 4: 187.
Kocher, A., Choct, M., Porter, M.D. and Broz, J. (2000). Poult. Sci.79: 1767.
Kocher, A., Choct, M., Porter, M.D. and Broz, J. (2002). Br. Poult. Sci. 43: 54.
Ledoux, D.R., Kutz, R.E., Murraroti, R. and Plumstead, P. (2009). Proc. Intern. Poult. Forum, Altanta, P.36.
Miller, E.L. (1976). In: From Plant to Animal Protein-Reviews in Rural Science II, eds. T.M. Sutherland, J.R. McWilliam and R.A. Leng, University of New England, Armidale, p. 47.
Neukom, H. (1976). ebensm.Wiss.Technol. 9: 143.
Neukom, H., Providoli, L., Gremli, H. and Hui, P.A. (1967). Cereal Chem. 44: 238.
Newman, R. K. and Newman, C. W. (1987). Nutr. Rep. Intern. 36: 693.
Omar, M.A. and Hamdan, A.M. (1998). Proc. 8th World Congress on Anim. Prod. P.306. Eds.: Seoul, Korea.
Perlin, A.S. (1951). Cereal Chem. 28: 370.
Péron, A. and Partridge, G.G. (2009). Rec. Adv. Anim. Nutr. 17: 9.
Petterson, D. and Åman, P. (1989). Br. J. Nutr. 62: 139.
Pettersson, D. and Åman, P. (1988). Anim. Feed Sci. Technol. 20: 313.
Pilnik, W. and Voragen, A.G.J. (1970). In: "The Biochemistry of Fruits and Their Products". Hulme,A.C. (ed.). Vol. 1, p53. (Academic Press, NY).
Purwadaria, T., Haryati, T., Darma, J. and Munazat, O.I. (1995). Bull. Anim. Sci. Special Edition, p.375.
Rogel, A.M. (1985). Ph.D Thesis, University of Sydney, Camden, Australia.
Sattagaroon, S., Kawakishi, S. and Namiki, M. (1983). J. Sc. Food Agric. 34: 855.
Saunders, R.M. (1986). Food Rev. Intern. 1: 465.
Shibuya, N and Misaki, A. (1978). Agric.Biol.Chem. 42: 2267.
Shurson, G., Piehs, M. and Whitney, M. (2004). Pig News Info. 25: 75N.
Stephen, A.M. and Cummings, J.H. (1979). Gut 20: 722.
Tran, S.T. (1996). The use of triticale in broiler chicken diets: its nutritive value and the effects of enzyme supplementation. Master's thesis. University of New England, Armidale, Australia.
Ward, N.E., Zijltra, R.T., Parsons, C. and Starkey, C. (2008). Proc. Intern. Poultry. Sci. Forum, Intern. Poultry Expo.
Zamora, A.F., Calapardo, M.R., Rosano, K.P., Luis, E.S. and Dalmacio, I.F. (1989). Proc. FAO/UNDP Workshop on Biotechnology in Animal Production and Health in Asia and Latin America. p.312.
This presentation was given at the IV CLANA CBNA/AMENA, São Pedro, SP, Brazil in november 2010. Engormix.com thanks the author and the organizing committee for this huge contribution.
To know more about this topic, watch this video: