I. INTRODUCTION
Infectious laryngotracheitis (ILT) is an important respiratory disease of chickens caused by ILT virus (ILTV, Gallid herpesvirus 1) that infects the upper respiratory tract and conjunctiva resulting in high morbidity and sometimes mortality (García et al., 2013). Vaccination with live attenuated vaccines administered by eye drop is generally effective: however for commercial meat chickens mass vaccination in drinking water via nipple drinkers is generally practiced. This method increases the risk of vaccination failure due to the lack of vaccine contact with the upper respiratory tract and conjunctival tissues (Robertson and Egerton, 1981; Menendez et al., 2014). Due to the lack of practical and economically viable methods, no evaluation of vaccination success is undertaken following mass vaccination of commercial meat chickens. A method for monitoring of vaccinal or wild type strains of a related herpesvirus, Marek’s disease virus, in poultry dust by real-time quantitative PCR (qPCR) has been successfully implemented by the poultry industry (Walkden-Brown et al., 2013; Kennedy et al., 2017). The feasibility of using this method to determine vaccination success for other poultry vaccines needs to be determined. A previous study has shown that high ILTV genomic copies (GC) are detected in faeces and dust after ILT vaccination by oral inoculation (Roy et al., 2015). This study aims to determine under commercial conditions: 1. the level and duration of ILTV detection in dust at various times following water based ILT vaccination, and 2. if sampling location within a shed influences vILTV GC detection.
II. METHODS
During a natural outbreak of ILT in the greater Sydney region, preventive mass vaccination with the Serva strain of ILTV vaccine (Nobilis ILT, MSD) in meat chickens was implemented. Flocks in four conventional and four tunnel ventilated meat chicken sheds on 4 farms were included in the study. Water vaccination was conducted by the farmers at 7 - 14 days of age. Two to six settle plates were installed in each shed on the day of vaccination. The settle plates comprised an L-shaped piece of flat metal with a plastic plate bolted to the horizontal section. The vertical portion was attached to vertical wires in the shed using a clamp mechanism. A second plate sat in the first plate and could be easily removed for dust collection into preweighed in plastic zip-lock bags. The surface area of the settle plate was 520 cm2. Dust samples were collected from each plate at 4, 7-8, 12-13 and 25 days post vaccination. Samples were weighed in the bags and stored at -20°C until needed. DNA was extracted from 5 mg of dust from each sample using the ISOLATE II Genomic DNA kit (Roy et al., 2015). Extracted DNA was tested for ILTV by qPCR targeting the gC gene (Callison et al., 2007; Roy et al., 2015). Results were reported as ILTV log10 genomic copies (GC) per mg of dust based on a plasmid preparation of the target sequence (Roy et al., 2015).
Statistical analyses were performed using JMP v.14 software (SAS Institute, Cary, NC, USA). Analysis of variance was used with viral GC fitted as the variable effect and settle plate location, shed type, and day post vaccination (dpv) fitted as fixed effects. Data are presented as least-squares means (LSM±SEM). Chi-square test was used for effect of location on ILTV GC detection rate. P < 0.05 was considered statistically significant.
III. RESULTS
The proportion of positive samples varied between flocks and day post vaccination (Table 1) (P < 0.001). Overall, two ILTV detection patterns were observed, flocks in which positive samples were first detected at 7-8 dpv and positive thereafter (Flocks 2, 3, 4, 5 and 7) and flocks in which samples were first consistently positive at or later than 12-13 dpv (Flocks 1, 6 and 8). Unusually, in Flock 1, 33% of dust samples were positive for ILTV GC at 4 dpv but then negative until 25 dpv. Of the 32 combinations of sample time × flock, 27 (84%) all dust samples were uniformly positive or negative. A mixture of positive and negative samples was mostly observed when ILTV GC load was low or decreasing (Table 1, Figure 1).
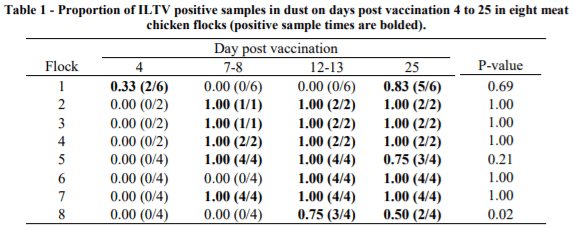
Overall, settle plate location had no impact in the ILTV GC detection rates (P = 0.90) except for Flock 1 on 4 dpv and 25dpv; Flock 5 on 25dpv; and Flock 8 on 13dpv and 25dpv (Table 1). All dust samples collected from Flocks 2 to 4 and Flocks 6 and 7 at a given dpv were consistently positive or negative for the duration of the study. For the remaining flocks, the detection rates for a given dpv were between 33% and 83% (Table 1).
Likewise, ILTV GC varied between flocks and dpv (P < 0.001) (Figure 1). At 7-8 dpv, five flocks (2 - 5 and 7) exhibited ILTV log10 GC between 3.11 and 7.8 with high values persisting until the end of the study at 25 dpv. The remaining three flocks (1, 6 and 8) had low ILTV log10 GC (0 - 0.09) before 12-13 dpv, with rapid increase afterwards.
IV. DISCUSSION
ILTV vaccine GC were detected in dust samples from 4 to 25 dpv. At 4 dpv, most samples were negative for ILTV but the number of positive samples increased at variable rates over time. The results are consistent with Roy et al. (2015), who reported ILTV GC detection in dust samples from 7 to 28 dpv in birds orally vaccinated with ILTV SA2 and A20 strains.
A rapid increase of ILTV GC was observed shortly after vaccination with the ILTV Serva strain in five of eight flocks which is suggestive of vaccination success, whereas absence of detection of ILTV at 7-8 dpi or detection of very low levels in the other 3 flocks is an indication of a low level of early vaccination take. This difference between the flocks reflected real differences in vaccine take in these flocks detected by tracheal swab measurements of individual birds (Groves et al., 2018). However, the variation in vaccination take observed in the present studies highlights risk of vaccination failure with water application through nipple drinkers due to the lack of vaccine contact with the upper respiratory tract and conjunctival tissues (Robertson and Egerton, 1981; Menendez et al., 2014). Taken together, these results suggest that the best point of differentiation among flocks with adequate or poor vaccine take is at 7-8 dpv. Peak of ILTV GC detection at later time points would indicate secondary bird to bird transmission resulting from non-uniform initial vaccination. It should be noted that the tracheal swab data of Groves et al., (2018) revealed that in only one flock were more than 50% of chickens ILTV positive at 4 dpv so even the best vaccination events in this experiment may have been sub-optimal. Very low initial vaccination take could explain the low ILTV GC detection rates at 4 dpv on Flock 1 followed by negative results until 25 dpv.
Regarding sampling location, no significant variation was observed in the detection rates for ILTV GC; therefore, a sample from a location in the shed was as good as from any other. Kennedy et al. (2017) suggested that collection of dust sample from poultry sheds using settle plates could be biased as distribution of virus in a shed may not uniform. Although location had no significant effect in this study, as not all samples collected from at a given day were positive, pooling samples from two or more dust plates per flock would maximise ILTV GC detection and overcome possible differences in virus distribution in a shed, particularly at low levels of GC.
In this study, collection of dust samples using polypropylene plastic settle plates provided a convenient sampling method for vaccine and pathogen monitoring thus would be suitable for use under commercial conditions. Similar but relatively expensive metal settle plates have been used by Skóra et al. (2016) for evaluating microbial contaminations in poultry dust. The design optimisation of the settle plates described in this study provides a device for dust collection that is inexpensive, durable, easy to manufacture and convenient to handle in commercial poultry settings.
In conclusion, ILTV is detectable in commercial meat chicken dust most readily from 7-8 dpv and the presence of significant GC load at 7-8 dpv appears to be a reasonable basis for differentiating adequate and poor initial vaccine take. It is possible that earlier sampling may be required to differentiate poor from adequate take and this is worthy of further investigation. The lack of spatial variation within a shed suggests that choosing a random sampling location within a shed will not affect virus detection but pooling samples from two to four settle plates would be ideal for maximising sensitivity.
ACKNOWLEDGEMENTS: We are thankful to the farm owners for providing access to the farms and flocks information to conduct this study. Thanks to Yugal Raj Bindari for support during sample collection, and to Rural Industry Research and Development Corporation (RIRDC) project PRJ-010639 for funding.
Abstract presented at the 30th Annual Australian Poultry Science Symposium 2019. For information on the latest edition and future events, check out https://www.apss2021.com.au/.