INTRODUCTION
Cereal grains such as wheat, sorghum, barley, and corn are commonly used in poultry diets as major sources of energy. Knowledge of the ME content of cereal grains is critical for their efficient and sustainable use and precise poultry feed formulation. Despite several limitations (Mateos et al., 2019; Wu et al., 2020), the AME is the globally accepted system for describing the available energy for poultry (Hill and Anderson, 1958; Sibbald, 1982). The simplicity, relatively easy measurements, and the fact that it accounts for most of the energy losses after digestion and metabolism are the major reasons for its worldwide acceptance.
When formulating commercial poultry diets, the current practice in the feed industry is to use the AME or AMEn values of ingredients from equations or reference tables which have been determined using older birds (NRC, 1994; Rostagno et al., 2005). Most published data on the AMEn in feed ingredients have been generated with older broilers (typically 5-wk-old) and used in feed formulations for all the phases of broiler growth. However, this practice overlooks the potential effect of bird age on the AMEn content of feed ingredients. Bird age has been shown to influence the digestion and absorption of energy-yielding nutrients (Batal and Parsons, 2002; Tancharoenrat et al., 2013). Birds of different ages show variable capacity to digest and metabolize nutrients especially in feed ingredients containing antinutritive substances such as soluble non-starch polysaccharides, tannins, and phytic acid (del Alamo et al., 2008; Kiarie et al., 2014). Moreover, the capacity of digestive tract to digest and absorb nutrients is limited during the early life of broilers and there is consensus that the nutrient digestibility generally increases with advancing age (Brumano et al., 2006; Olukosi et al., 2007). Several studies have reported higher dietary AME values in older broilers than in younger birds (Zelenka, 1968; Scott et al., 1998; Batal and Parsons, 2002). A positive linear correlation between age and AME of a wheat-based diet has also been reported in broilers from 8 to 16 d (Scott et al., 1998). In contrast, some studies have shown a negative or no age effect on the AME. Fonolla et al. (1981) revealed that the AME value of a corn-based diet did not differ between 21 and 52 d of age. Bartov (1995) showed that the AMEn of corn- and sorghum-based diets reduced with advancing age from 11 to 22 d of age. Despite the importance of age effect, a scan of the literature reveals that there is a dearth of published data on age-related values for the AMEn of common cereal grains over the growth cycle of broilers. The relevance of using a single value of AMEn obtained with older birds to all growth phases, especially the early life, of broilers is questionable and highlights the need for age-dependent estimates for use in feed formulations.
All previous studies on age-related responses have been conducted with grain-based complete diets (Olukosi and Bedford, 2019; Yang et al., 2020). To our knowledge, no published data are available on age effects for the AMEn of individual cereal grains. Therefore, the aim of the present study was to investigate whether the age of broiler chickens has any effect on the AMEn of commonly used cereal grains (wheat, sorghum, barley, and corn).
MATERIALS AND METHODS
The experiment was conducted according to the New Zealand Revised Code of Ethical Conduct for the use of live animals for research, testing and teaching and approved by the Massey University Animal Ethics Committee.
Ingredients
Four cereal grains (wheat, sorghum, barley, and corn) were obtained from a local commercial supplier. The proximate and nutrient composition of the cereal grains are presented in Table 1. The wheat and sorghum samples were sourced from Australia, and corn and barley were of New Zealand origin. All grains were ground in a hammer mill to pass through a screen size of 3.0 mm.
Diets, Birds, and Housing
The AME was determined using the direct method. In this method, four experimental diets were formulated to contain the same inclusion level (962 g/kg) of each grain, and metabolism are the major reasons for its worldwide acceptance.
When formulating commercial poultry diets, the current practice in the feed industry is to use the AME or AMEn values of ingredients from equations or reference tables which have been determined using older birds (NRC, 1994; Rostagno et al., 2005). Most published data on the AMEn in feed ingredients have been generated with older broilers (typically 5-wk-old) and used in feed formulations for all the phases of broiler growth. However, this practice overlooks the potential effect of bird age on the AMEn content of feed ingredients. Bird age has been shown to influence the digestion and absorption of energy-yielding nutrients (Batal and Parsons, 2002; Tancharoenrat et al., 2013). Birds of different ages show variable capacity to digest and metabolize nutrients especially in feed ingredients containing antinutritive substances such as soluble non-starch polysaccharides, tannins, and phytic acid (del Alamo et al., 2008; Kiarie et al., 2014). Moreover, the capacity of and fortified with macro minerals and, vitamin and trace mineral premixes. Diets were mixed in a single-screw paddle mixer (Bonser Engineering Co. Pty. Ltd., Merrylands, Australia), then pelleted using a pellet mill (Model Orbit 15; Richard Sizer, Kingston-upon-Hull, UK) capable of manufacturing 180 kg of feed/h and equipped with a die ring with 3-mm holes and 35 mm thickness.
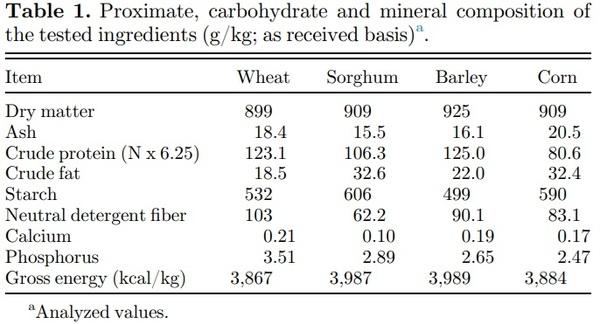
Day-old male broilers (Ross 308) were obtained from a local hatchery and raised on floor pens in an environmentally controlled room until assigned weekly to the experimental treatments. Except for the 1 to 7 d age group, birds were fed broiler starter mini pellets (230 g/kg crude protein and 3,010 kcal/kg AMEn) until d 21 and finisher pellets (207 g/kg crude protein and 3,100 kcal/kg AMEn) from d 21 to 35 (Table 2). At the beginning of each week (d 1, 8, 15, 22, 29, and 36), new batch of birds were selected randomly from the floor pens, weighed individually and allocated to cages so that the average bird weight per cage was similar. For each cereal grain, the assay diet was fed to 6 replicate cages of broilers during 6 periods, namely wk 1 (d 1−7), wk 2 (d 8−14), wk 3 (d 15−21), wk 4 (d 22−28), week 5 (d 29-35), or wk 6 (d 36−42). Each replicate cage housed 10 birds during wk 1, and 8 birds during wk 2 to 6 posthatch.
Determination of Metabolizable Energy
The AME was determined using the total excreta collection procedure (Hill and Anderson, 1958). During each week, diets were fed for 7 d, with the first 3 d serving as an adaptation period. The feed intake (FI) and total excreta output for each replicate cage were recorded over the last 4 consecutive d of assay. Daily excreta collections were pooled within a replicate cage mixed in a blender and subsampled. Subsamples were lyophilized (Model 0610, Cuddon Engineering, Blenheim, New Zealand), and dried excreta samples were ground to pass through a 0.5- mm sieve and stored in airtight plastic containers at 4°C pending analysis. The diet and excreta samples were analyzed for DM, gross energy (GE), and nitrogen (N).
Chemical Analysis
Dry matter was determined using standard procedures (Methods 930.15; AOAC, 2016). Ash was determined by a standard procedure (Method 942.05; AOAC, 2016) using a muffle furnace at 550°C for 16 h. Nitrogen was determined by combustion (Method 968.06; AOAC, 2016) using a carbon nanosphere-200 carbon, N and sulfur auto analyzer (rapid MAX N exceed, Elementar, Donaustraze, Hanau, Germany). The crude protein content was calculated as N x 6.25. The crude fat was determined by Soxtec extraction procedure (Method 2003.06;AOAC, 2016) using (Soxtec System HT 1043 Extraction Unit, H€ogan€as, Sweden). Starch was measured using a Megazyme kit (Method 996.11; AOAC, 2016) based on thermostable a-amylase and amyloglucosidase (McCleary et al., 1997). The neutral detergent fiber (Method 2002.04; AOAC, 2016) was determined using Tecator Fibertec (FOSS Analytical AB, H€ogan€as, Sweden). For minerals, samples were ashed and then calcium and phosphorus were determined colorimetrically following digestion with HCl (Method 968.08D; AOAC, 2005). Gross energy was determined by adiabatic bomb calorimeter (Gallenkamp Autobomb, London, UK) standardized with benzoic acid.
Calculations
All data were expressed on a DM basis. The AME of experimental diets was determined using the following formula:
AMEDiet (kcal/kg)
= [(FI x GEdiet) -- -- (Excretaouput x GEexcreta)]/FI
The AME of cereal grains was then calculated using the following formula:
AMEgrain (kcal/kg)
= AME of test grain diet x (100/96.2)
Nitrogen retention, as a percentage of intake, was determined as follows:
N retention (%)
= 100 x [((FI x Ndiet) -- (Excreta output x Nexcreta))/((F1 x Ndiet)]
The AMEn was then calculated by correction for zero N retention by assuming 8.73 kcal per g N retained in the body as described by Titus et al. (1959).
Statistical Analysis
The data for each grain were analyzed by one-way ANOVA using the General Linear Models procedure of the SAS (version 9.4; 2015. SAS Institute Inc., Cary, NC). Cages served as the experimental unit. Significant differences between means were separated by Least Significant Difference test. In addition, the data were subjected to orthogonal polynomial contrasts using the General Linear Models procedure of SAS (2015) to study whether responses to increasing bird age were of linear or quadratic nature. Significance of effects was declared at P < 0.05.
RESULTS
The influence of broiler age on the retention of DM and N, AME and AMEn of wheat is summarized in Table 3. The retention of both DM and N declined quadratically (P < 0.001) with advancing age. The highest DM and N retentions were recorded in wk 1. Bird age had a significant (P < 0.001) effect on the AME and AMEn of wheat. The AMEn of wheat declined quadratically (P < 0.01) with advancing age, from 3,461 kcal/kg in wk 1 to 3,219 kcal/kg in wk 2 and then plateauing to wk 6 (Figure 1A).
In birds fed sorghum-based diets, DM (P < 0.01) and N (P < 0.001) retentions decreased quadratically with advancing age of birds, where wk 1 showed the highest DM and N retentions, and then both DM and N retentions declined at wk 6 (Table 4). The AMEn of sorghum declined linearly (P < 0.001) with advancing age, from 3,762 kcal/kg in wk 1 to 3,614 kcal/kg in wk 2, plateaued to wk 5 and then declined further to 3,556 kcal/kg in wk 6 (Figure 1B).
For barley-based diets, DM and N retentions showed a significant (P < 0.001) quadratic response to bird age, where birds retained the highest DM and N during wk 1 (Table 5). Bird age had a significant (P < 0.001) effect on the AME and AMEn of barley. A quadratic (P < 0.001) reduction in the AMEn of barley was observed as birds grew older, with the AMEn decreasing between wk 1 (3,286 kcal/kg) and wk 2 (2,988 kcal/kg), increasing in wk 3 (3,117 kcal/ kg) and then plateauing (Figure 1C).
In birds fed corn-based diets, the highest DM and N retentions were observed in wk 1 and declined linearly (P < 0.05) for DM retention and quadratically (P < 0.05) for N retention with advancing age (Table 6). The AMEn of corn declined quadratically (P < 0.05) with advancing broiler age; the highest AMEn was observed in wk 1 and 5, the lowest AMEn in wk 2, with other weeks being intermediate (Figure 1D).
DISCUSSION
To the authors’ knowledge, no published data are available on age-related energy utilization responses of individual cereal grains from wk 1 to 6 of broiler chickens. A perusal of literature shows that previous studies comparing age effects have been conducted with grain-based complete diets at specific ages and most do not include wk 1. Scott et al. (1998) showed that birds at d 8 of age had a lower AME of wheat compared to d 16 (3,420 vs. 3,530 kcal/kg); the same trend was observed for barley, where the AME increased by 100 kcal/kg at d 16. Batal and Parsons (2004) found that the AMEn of a corn-soy diet increased numerically by 98 kcal/kg from d 14 to 21. Thomas et al. (2008) reported that the AMEn of a corn-based diet was 2,935 kcal/kg at d 7 and then increased to 3,109 kcal/kg at d 14 of age. However, N retention decreased with advancing age of birds from 75.5% at d 3 to 60.5% at d 14. These researchers also observed similar trends for wheat- and sorghum-based diets. In contrast, Bartov (1995) reported a significant reduction in the AMEn of a sorghum-corn-based diet from 2,923 kcal/kg at 13 d of age to 2,892 kcal/kg at 22 d of age.
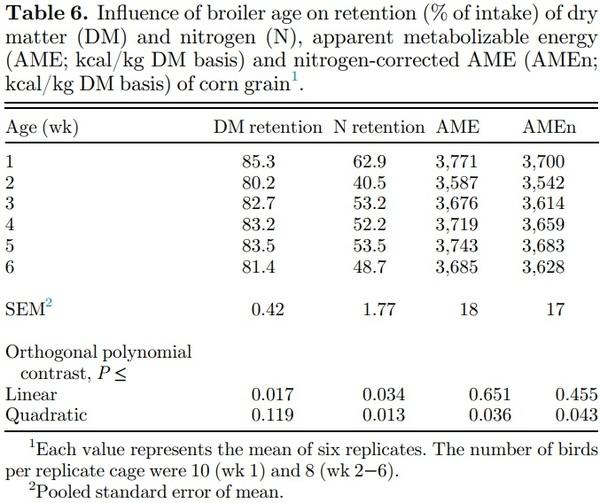
The principal motive for the present study was therefore to investigate whether 1) the age of broilers influences the AME content of common cereal grains and 2) the age effect, if present, is similar for all grains. The results showed that the AMEn of cereal grains were influenced by bird age, regardless of cereal type. The highest AMEn values were observed at the first week for all cereal grains and then the AMEn declined, either linearly (sorghum) or quadratically (wheat, barley, and corn). Similarly, the highest DM and N retention values were observed at the first week and then declined quadratically for all cereal grains. The only exception was the DM retention in corn, which declined linearly. The reductions in DM and N retention after wk 1 were associated with reductions in the AMEn of all grains.
The higher AMEn and retention of DM and N determined for wk 1 are counter-intuitive, since the digestive tract of the newly hatched chick is immature and must undergo dramatic changes before it can efficiently digest and absorb nutrients. The obvious limiting factors are the secretion and activities of digestive enzymes, and the surface area for absorption. These limitations are overcome with advancing age, resulting in concurrent improvements in nutrient utilization (Noy and Sklan, 2001). Uni et al. (1995) reported that the N retention increased from 70% at d 4 to 90% at d 14 posthatching. Similarly, they reported that the starch digestibility increased by 14% between d 4 and d 14 posthatching. Olukosi et al. (2007) observed that DM and N retention increased by 20.9% and 19.9%, respectively between wk 1 and 2. A study by Murakami et al. (1992) showed a lower AME during the first few days of life followed by an increase after the first week. In contrast, Moss et al. (2020) reported higher ME:GE ratios in broiler chicks (7−9 d) than older broilers (33−34 d) in diets based on wheat (0.799 vs. 0.765), sorghum (0.782 vs. 0.713), or DM and N retentions corn (0.796 vs. 0.785).
Three possible explanations may be provided to justify the high AMEn values determined during wk 1. First, the presence of residual yolk sac may be imparting beneficial effects on the digestion and utilization of lipids and protein, contributing to the high AMEn (Zelenka, 1968). The newly hatched chick relies on residual egg yolk lipids as the primary source of energy up to d 5 post-hatch (Sell et al., 1991; Murakami et al., 1992). Noy and Sklan (2001) estimated that the yolk represents about 20% of the body weight of the hatchling and contains about 50% lipids, providing readily available energy post-hatch. Break-down of yolk lipids, by the lipolytic enzymes in the yolk sac, provides more than 90% of the total energy required for the hatchling (Speake et al., 1998). Murakami et al. (1992) estimated that the residual yolk contributed for approximately 30% toward the total dietary energy intake from d 0 to 3 of age. It is worth emphasizing, at this point, that the energy is not a nutrient per se, but a property of energy-yielding nutrients (carbohydrates, lipids, and protein). The energy derived from carbohydrates, lipids, and protein differs, with lipids providing 2.5 times GE than carbohydrates and protein. It is therefore tempting to propose that the dependence of the newly hatched chick on high-energy yolk lipids offers it an AME advantage. However, the exact mechanism or contribution of the residual yolk sac towards nutrient utilization remains unclear (van der Wagt et al., 2020).
Second, the FI during the first week post-hatch is low as the hatchling adapts to the move from yolk nutrition to oral nutrition. It has been accepted that the dietary energy has a regulatory role on FI and birds alter their feed consumption primarily to meet their energy requirements (Leeson et al., 1993; Lamot et al., 2017). Zelenka (1997) speculated that the decrease in AMEn with advancing age could be due to increased feed consumption. A negative correlation (r = 0.64) between FI and AME in wheat-based diets has been demonstrated by Scott (2005).
Lower FI reduces the feed passage rate in the digestive tract, which improves the digestibility of nutrients by increasing the time of contact with digestive enzymes and absorptive cells (Washburn, 1991). In a study, ten Doeschate et al. (1993) found that the DM and N digestibility of a corn-soy diet was higher when the FI was lower. With advancing age, increasing consumption of diets with high inclusions of cereals overload the digestive tract with starch, resulting in incomplete digestion of starch. Under these conditions, a negative correlation was observed between FI and starch digestibility in wheat, causing reductions in the AME (Svihus and Hetland, 2001; Svihus, 2011). Vergara et al. (1989) noted that the increase in digesta passage rate with age is related to increased FI. Hughes (2008) reported a linear correlation between the AME and total tract digesta transit time (r = 0.31). Uni et al. (1995) showed that the passage time of digesta in the digestive tract increased from 74 min at d 7 to 122 min at d 22 of age.
Finally, the role of the microbiome, or the lack of it, in the newly hatched chick cannot be discounted. The digestive tract of the hatchlings is sterile (Gabriel et al., 2006) but rapidly colonized by microbiome via the feed and environment. Intestinal microbiome contains various bacterial species that are heavy consumers of amino acids and energy (McBurney et al., 2003) for their rapid growth and colonization. Thus, the absence or low bacterial population during wk 1 may provide an advantage to the host in terms of nutrient utilization and AME.
Subsequent decline in the AMEn after wk 1 may be partly attributed to the increasing load of microbiome.
The current findings disagree with the widely assumed perception that energy utilization increases with age (Tancharoenrat et al., 2013; Yang et al., 2020). The absence of positive effect of advancing age on the AMEn of all grains in the current study was also confirmed by the lack of positive effects on DM and N retention. Studies on the influence of age of birds on nutrient digestibility have shown contradictory results. Uni et al. (1995) revealed that the digestibility of starch, N and fatty acids increased with advancing age. Szczurek et al. (2020), on the other hand, reported that the apparent and standardized ileal digestibility coefficients of amino acids of wheat were higher in birds at 14 d of age vs. 27 d of age. Similarly, Olukosi and Bedford (2019) showed a reduction in fat digestibility by 45% between d 7 and d 14 of age of broiler chickens, which was attributed to the faster passage rate in older birds. In contrast, Tancharoenrat et al. (2013) found that lipid digestibility in soybean oil increased by 50% at wk 2 compared to wk 1 post-hatch (0.898 vs. 0.591), which was attributed to limitations of bile secretion and emulsification to utilize fats.
One possible reason for the discrepancies reported between studies is the use of different methodologies. Several approaches are available to determine the AME, with the main consideration being the inclusion level of the test ingredient in the test diet (Wu et al., 2020). Calculations of the AME value of ingredients also differ in different methods. Even within the same method, AME of ingredients can vary depending on the formulation of the basal diet (Wu et al., 2020). According to Sibbald (1982), AME estimates are lowered with increasing inclusion levels of the test ingredient in assay diets. McIntosh et al. (1962), however, concluded that the diet composition (balanced or unbalanced) has no influence of AME of ingredients.
The direct method was employed in the present study to measure the AME of cereals, wherein the test diets were composed almost solely with the test ingredient. This method is simple and the need for a reference diet is eliminated. However, some ingredients are not palatable and cannot be tested by the direct method. An alternative method is the substitution method, where the test ingredient is substituted for a portion of a reference diet composed of practical ingredients. Differences between methodologies may contribute to discrepancies in AME values of ingredients. Lockhart et al. (1967) found that the AME of wheat measured by the substitution method was 81 kcal/kg higher than that measured by the direct method. Olukosi (2021) reported that the AMEn value of barley measured by the regression method was 708 kcal/kg greater than that measured by the substitution method (2,622 vs. 1,914 kcal/kg); however, the AMEn of corn was not influenced by the methodology suggesting that the influence of the methodology on AME estimations is ingredient dependent. Similarly, Lee and Kong (2019) comparing direct vs. regression methods and Veluri and Olukosi (2020) studying substitution vs. regression methods, showed that the assay method can influence the AME and AMEn value of feed ingredients, and that should be considered in cross-study comparisons. Given that the methodology can influence AME estimates, future studies are warranted to measure the AME of cereals using other methods at different broiler ages.
CONCLUSIONS
The results of the present study demonstrated that, regardless of the cereal, the age of broilers has substantial impact on the AMEn values of cereal grains. These results question the validity of applying a single AME or AMEn value for broilers of different ages, which can under- or overestimate the energy utilization. Therefore, to improve the precision of feed formulations and production efficiency, age dependent AME and AMEn values may need to be considered when formulating broiler diets.