Introduction
Increasing use of distillers dried grains with solubles (DDGS), which contains high levels of unsaturated fatty acids (UFA) such as n-6 UFA, alters the fatty acid (FA) profile of tissues in pigs to contain more UFA with a higher iodine value (IV) that results in soft fat pork (Cromwell et al., 2011; McClelland et al., 2012). Also, increased deposition of n-6 UFA in tissues may result in increased oxidative problems for both the health of pigs themselves and the resultant pork products (Lauridsen et al., 1999). The susceptibility of pork to lipid peroxidation and rancidity has been shown to increase with high levels of polyunsaturated fatty acids (PUFA) and the degree of unsaturation of the pork to increase due to consumption of unsaturated fat sources (Mitchaothai et al., 2007; Browne et al., 2013).
Many attempts have been made to solve problems related to a high degree of unsaturation of dietary fat to prevent peroxidation of the resultant pork fat; attempts include using different fat sources or by supplementing antioxidants such as vitamin E (VE). Previous studies reported that using different fat sources such as beef tallow (TW), poultry fat, coconut oil (CN), and vegetable oils could modify the FA profile in adipose tissues and meat (Mitchaothai et al., 2007; Apple et al., 2009a,b,c; Chen et al., 2021; Świątkiewicz et al., 2021), and this could affect oxidative stability of pork since different FA have different oxidative capacity (Kellner et al., 2016).
VE is an essential vitamin for pigs and, as an antioxidant, plays important roles in reducing oxidative stress, improving oxidative stability of pork and prolonging fresh pork shelf life (Boler et al., 2009). Because VE is a fat-soluble vitamin, its absorption and bioavailability depend on the properties of the dietary fat. This fact was demonstrated by Prévéraud et al. (2014) in which α-tocopherol concentrations in plasma and tissues of pigs were influenced by dietary fat sources wherein α-tocopherol concentrations in plasma and tissues actually decreased when pigs were fed high PUFA diets (from linseed and safflower oil). Gallo-Torres et al. (1978) also reported that the profile of dietary FA is one of the key factors to determine VE status and bioavailability in plasma and tissues.
Therefore, the objectives of this aspect of the project were to identify the impacts of different dietary fat source and variable VE supplementation to the pigs grown to heavy slaughter weight on tissue FA profile, plasma and tissue VE concentrations, and antioxidant capacity.
Materials and Methods
This experiment was carried out in an environmentally controlled room at the University of Kentucky Swine Research Center. The animal slaughter and sample collections were performed at the University of Kentucky Meat Science Laboratory. The experiment was conducted under protocols approved by the Institutional Animal Care and Use Committee of the University of Kentucky.
Animals, experimental design, and housing
A total of 64 individually-fed crossbred pigs (Yorkshire * Landrace * Duroc; 32 barrows and 32 gilts; 28.41 ± 0.83 kg) were selected from a pool of 180 pigs, blocked by sex and body weight, and then randomly assigned to one of eight dietary treatments in a 4 × 2 factorial arrangement of four fat treatments (including corn starch [CS], TW, distiller’s corn oil [DCO] and CN) and two VE supplementation levels (11 and 200 ppm) in the form of DL (all-rac)-α-tocopheryl acetate (ATA, ROVIMIX E 50 ADS, DSM Nutritional Products, Inc., GA). As defined in NRC (2012), one IU VE is equal to 1 mg of dl-α-tocopheryl acetate. All pigs were housed in individual pens (0.61 × 2.44 m2) with free access to water and feed.
Diets
The diets were corn-soybean meal based in mash form and fed for five weight phases including 25 to 50 kg (Phase 1), 50 to 75 kg (Phase 2), 75 to 100 kg (Phase 3), 100 to 125 kg (Phase 4), and 125 to 150 kg (Phase 5). All experimental diets were formulated to meet or exceed nutrient requirement estimates of NRC (2012) for grow-finishing pigs including the addition of 0.30 mg of Se as sodium selenite per kg diet. Lysine levels for Phase 4 and Phase 5 were calculated with the formula provided by NRC (2012) because the pigs were slaughtered at ~150 kg, which was greater than the final tabular weight in NRC (2012). The fat inclusion level (5%) was based on the amount of corn oil that might be realized from an aggressive use of DDGS in the finishing diet. The CS diet was formulated to equalize presumed daily intake of nonfat dietary ingredients to the 5% fat-added diets by maintaining constant lysine:ME ratio between the CS and the fat-added diets. To minimize differences in non-treatment components of the diets, a basal diet for each of the four fat sources was first mixed. Then, each basal diet was divided into two fractions. One fraction was mixed with a premix to provide 11 ppm VE, and the other fraction was mixed with a premix to provide 200 ppm VE. Representative samples of corn, soybean meal, and mixed feed were collected at the feed mill for every batch of experimental diets. Samples were stored at 4 °C until analyzed for concentration of VE and FA profile in each diet.
Blood collection
Blood samples from each pig were collected initially and at the end of each phase by vena cava puncture using a syringe. After the blood collection, whole blood was transferred to a 16 × 100 mm vacutainer tube containing the anticoagulant heparin (Becton, Dickinson and Company, Franklin Lakes, NJ). Plasma samples were obtained by centrifugation at 2,500 × g for 20 min at 4 °C and then aliquoted into 1.5- mL Eppendorf Safe-Lock tubes (Eppendorf North America, Hauppauge, NY), and stored at −80 °C until plasma α-tocopherol and antioxidant capacity analyses.
Harvest and tissue sampling
Pigs were slaughtered at about 150-kg live weight under USDA inspection. After being transferred to the meat lab, pigs were slaughtered after a rest of at least 30 min. The slaughter process included electrical stunning, exsanguination, dehairing, evisceration, and carcass washing. During the process of slaughter, liver and its subsamples (only left lateral lobe) were collected within 20 min after evisceration, bagged with moisture barrier bags, flash frozen with liquid nitrogen, and then stored at −80 °C for further analysis for VE concentration, antioxidants, fat content, and FA profile.
After a 24-h cooler chill, the primal cuts were removed and fat samples from the 10th-rib backfat and the belly area even with the 1st rib were taken during the process of primal cut division. These samples were used for FA analysis and the calculation of IV value. The fat samples were vacuum packaged, and then stored at −80 °C until transported to the University of Georgia for FA profile analysis. Loin samples were also obtained after the removal of primal cuts. Two 2.54-cm chops of loin sample (around 200 g each) located at the 10th rib were collected, vacuum packaged, and stored at −22 °C until further analysis for VE concentrations.
Chemical analyses
Samples were analyzed for FA composition at the University of Georgia (including diets, liver, belly fat, and backfat tissues). Fatty acid profiles were determined by gas chromatography (GC), using a Shimadzu gas chromatograph (Model 14 A, Columbia, MD) with a fame ionization detector, the procedure was modified from Park and Goins (1994). Approximately 2 g of each nonfat-added diet sample, 1 g of each fat-added diet sample, 100 mg of each adipose sample, and 2.0 g of liver were used for analysis. After thawing, the fat cores of back fat tissues were trimmed free of lean and skin and separated into the outer layer and inner layer of fat. Samples were processed through a two-step methylation procedure. The first step was heating in 0.5 N sodium methoxide in methanol for 30 min at 90 °C, followed by the addition of boron trifluoride in methanol and heating for another 20 min. Methyl esters were then isolated in hexane and at the same time anhydrous sodium sulfate was added to remove any residual water. The processed samples were stored at 4 °C until analyzed. Tridecanoic acid (2 mg/ml in methanol) was used as the internal standard. Fatty acid methyl esters were separated on a Phenomenex, ZBWax Plus wide-bore capillary column (Phenomonex, Torrance, CA) with nitrogen as the carrier gas. Initial column temperature was 160 °C, which was held for 10 min and increased at a rate of 5 °C/min to 220 °C. Injector temperature was 250 °C, and detector temperature was 260 °C. Peaks were identified by comparison of retention times of known standards. Quantification was corrected for recovery of the internal standard and is based on the reference standard. The minimum detection limit for FAs was set at 0.01%; relative percentages of FAs detected below this level were denoted as not detected (ND). The IV of fat source and tissue was calculated using the equation below (Meadus et al., 2010), which is modified from the recommended method of AOAC, wherein the percentage of specific FA are aggregated into the collective IV value.
IV = (C16 : 1 × 0.95)+(C18 : 1 × 0.86)+(C18 : 2 × 1.732) + (C18 : 3 × 2.616)+(C20 : 1 × 0.795)+(C20 : 2 × 1.57) + (C20 : 3 × 2.38)+(C20 : 4 × 3.19)+(C20 : 5 × 4.01) + (C22 : 4 × 2.93)+(C22 : 5 × 3.68)+(C22 : 6 × 4.64)
Analysis of VE in diets, plasma, liver and loin muscle
Dietary VE was determined with a normal phase high-performance liquid chromatography (HPLC) system using fluorescence detection according to modification of an AOAC (1990) procedure (method 971.30). The concentration of VE in plasma and tissue samples was determined by HPLC at Veterinary Diagnostic Laboratory in Iowa State University. Briefly, the VE was extracted from plasma and tissues (liver and muscle samples) as described by Zaspel and Csallany (1983). Plasma and tissues extracts were then injected into an HPLC for tocopherol analysis. Tocopherols were separated by HPLC using a reversed-phase LC18-SB column (5 mm, 4.6 mm × 150 mm), the mobile phase was 100% methanol at 1.0 mL/min. Tocopherols were monitored by an UV detector at 280 nm. Identification and quantification of tocopherols were accomplished by comparison of retention time as well as peak areas with tocopherol standards of α-tocopheryl acetate and α-tocopherol. The results were expressed in mg VE/mL plasma or mg VE/g tissue.
Antioxidant capacity
Superoxide dismutase (SOD) activity was analyzed in the plasma samples collected at day 0 and at the end of each phase with SOD assay kits purchased from Cayman (Ann Arbor, MI). The SOD assay measurement measured all three types of SOD (Cu/Zn, Mn, and Fe SOD). Antioxidant measurements in the liver, including SOD activity, malondialdehyde content, glutathione (GSH), and glutathione disulfide (GSSG) concentrations, were analyzed with commercial assay kits purchased from Cayman.
Statistical analysis
Prior to statistical analyses, all data were evaluated to identify any potential statistical outliers according to the test published by Barnett and Lewis (1994). Briefly, a set of data is ranked from low to high: XL, X2 , ….XH, and the average and standard deviation are calculated, then suspected high or low outliers were tested by the following procedure: First, calculate the statistic T: T = (XH − mean)/s for a high value, or T = (XL − mean)/s for the low value (where s refers to the standard deviation). Second, compare the value of T with the value from critical values for 95% confidence interval (under condition of this study, the critical value is 2.03.) If the calculated T is larger than the critical value for the measurement, then the XL or XH is a potential outlier at the level of 5% significance. Potential outliers were then evaluated by reviewing study notes for that animal as well as response measures and laboratory values for other animals on that particular dietary treatment to determine if the value would be excluded from the data set.
Data analyses were performed in SAS (SAS Inst. Inc., Gary, NC) by least squares analysis of variance using the generalized linear model (GLM) as a randomized complete block design. The individual pig served as the experimental unit. The model used was:
Y = µ + Sl + Bi(l) + Vj + Fk + VFjk + SVlj + SFlk + εm(ijkl).
In this equation, the parameters represent Y = response variables (tissue fat content and fatty acid profile, VE concentration, and antioxidants), µ = overall population mean, Sl = sex (male or female), Bi(l) = block within sex (i=1, 2, 3….8), Vj = VE level (11 or 200 ppm), Fk = fat source (CS, TW, DCO, or CN).
When interactions between main effects were significant, further least squares mean separations were accomplished using the PDIFF option of SAS to analyze the treatment effects. Because these interactions were relatively sparse, main effect means are presented in the result tables and the few interactions are presented in a separate table for further information. In addition, plasma VE and SOD concentration data were also analyzed as repeated measures to determine the response trends over time.
Statistical differences were established at P ≤ 0.05, tendencies were established at P ≤ 0.10 for main effects. When it was present, the effect of sex (P ≤ 0.05) is superscripted for each measurement in the data table, interactions between sex and treatment effects are also superscripted with both described when the table is presented in the results. To focus attention on statistically relevant data, any P-value greater than 0.10 was replaced with “–”.
Results
Analyzed dietary VE level and fatty acid profile
The FA profile of different diets is provided in Table 1; the FA profile of the fats themselves is presented in Wang et al. (2022). Because the fat from the basal diets was mainly the oil from the corn used in the diets, the CS treatment had similar FA profile to the DCO treatment. For the TW and CN diets, while the 2.5% fat in the basal diet altered the FA profile of dietary fat treatments from the specific fat itself, the trend of the difference in FAs of the diets was still generally similar to that of the fat sources themselves. Among the four fat treatments, the CN treatment had the highest content of saturated fatty acid (SFA) and the lowest monounsaturated fatty acid (MUFA) and PUFA, the DCO treatment had the highest content of PUFA and the lowest content of SFA, and the TW treatment had the highest content of MUFA.
Table 1. Fatty acid profile1 of the diets according to main fat treatments (as-fed basis)
Regarding the VE content in the diets, the total VE content in the diets was different from the calculated level because of the presence of VE in the fat sources themselves and the resultant basal diets. As shown in Table 2, the analyzed α-tocopherol in acetate form followed the added VE as designed.
Intake of different fatty acids
The total intake of different FAs for the entire study followed the general trend of the fat treatments (Table 3). Among different fat treatments, pigs fed the CN diet had the highest intake of SFA including C6:0, C8:0, C10:0, C12:0, and C14:0 (P < 0.05); pigs fed the TW diet had the highest intake of MUFA including C16:1, C18:1, and C20:1 (P < 0.05); and pigs fed the DCO diet had the highest intake of PUFA including C18:2n6 and C18:3n3 (P < 0.05). Apart from these major groupings, pigs fed the TW diet also had the highest intake of C16:0, C17:0, C18:0, C18:3n6, and CLA (P < 0.05), while pigs fed the DCO diet had the highest intake of C20:0 (P < 0.05). Pigs fed the CS diet had the lowest total intake of lipid (P < 0.05) including most of the FA.
Due to the significant sex effect on ADFI, the intake of most FA was significantly different between barrows and gilts (P < 0.05) wherein barrows had higher intake of most FAs compared to gilts, with the magnitude of the difference dependent on the fat source. Interaction between sex and fat was also detected on the FAs with a chain length below 14, C20:2, and the SFA (P < 0.05). Barrows had greater C6:0, C8:0, C10:0, C12:0, C14:0, and C20:2 intake in fat-added diets than gilts, but gilts had greater intake than barrows in the CN diet (P < 0.05). The SFA intake was not different among sexes for the TW, CO, and CS diets, while barrows had greater SFA intake than gilts in the CN diet (P < 0.05).
There was no significant effect of increasing dietary VE level from 11 to 200 ppm on the FA intake except a tendency of reducing C18:3n6 intake (P = 0.10).
Tissue fatty acid profile in the backfat
Table 4 shows the effects of dietary VE supplementation and fat source on FA profile in the backfat. No interactions between dietary VE supplementation and fat source were observed on fatty acid profile in the backfat at slaughter except C20:4 in which increasing VE supplementation level increased C20:4 when pigs were fed the DCO diet but not any other diets. Differences (P < 0.001) were detected among pigs fed diets with the four fat treatments in all the FAs analyzed, wherein pigs fed the CN diet had the most SFA (P < 0.05) including C12:0, C14:0, and C16:0 (P < 0.05); pigs fed the DCO diet had most PUFA (P < 0.05) including C18:2n6, C18:3n3, C20:2, and C20:4 (P < 0.05) with the greatest IV (P < 0.05); pigs fed the TW diet had the most MUFA (P < 0.05) as well as C17:0, C18:1, C18:3n6, and CLA (P < 0.05) in the backfat. However, contrary to the total FA intake, pigs fed the CN diet had most C16:1 (P < 0.05) and pigs fed the CS diet had most C18:0 (P < 0.05) among different fat treatments. The VE supplementation only affected concentration of C16:1 (P = 0.03), C18:0 (P = 0.04), CLA (P = 0.08) and C20:0 (P = 0.01) in the backfat, where the concentration of C16:1 and CLA increased, but the concentration of C18:0 and C20:0 decreased with increasing dietary VE from 11 to 200 ppm.
Table 2. Vitamin E content of diets1 (as-fed basis)
Table 3. Effect of different fat source and Vitamin E (VE) supplementation on total intake1 of individual fatty acids in pigs from 28 to 150 kg
Table 4. Effect of different fat source and Vitamin E (VE) supplementation on fatty acid profile (%) in the backfat1
Sex affected the FA profile of backfat in the content of C20:0 and C20:4, wherein gilts had more C20:4 (P < 0.05) but less C20:0 (P < 0.05) than barrows. Interactions between sex and fat source were observed on content of C18:2n6, C20:0, and C20:2 (P < 0.05), and as a result PUFA (P < 0.05) and IV (P < 0.05) in the backfat, wherein gilts had more (P < 0.05) C18:2n6, C20:2, PUFA, and greater (P < 0.05) IV than barrows when they were fed the DCO diet but not the other diets, and barrows had more C20:0 than gilts when fed the DCO and TW diets (P < 0.05). No interaction between sex and VE supplementation was observed on FA profile in the backfat.
Fatty acid profile in the belly fat
As shown in Table 5, the FA profile of belly fat was affected by dietary treatment in a similar pattern to what happened in the backfat, although the absolute concentration of most FA was different from those of backfat. No interactions between dietary VE supplementation and fat source were observed on FA profile in the belly fat except C16:1 (P < 0.05) in which increasing VE supplementation level increased C16:1 when pigs were fed the CS and CN diets but not the TW and DCO diets. Differences (P < 0.05) were detected among pigs fed diets with the four fat treatments in all the FAs analyzed, wherein pigs fed the CN diet had most SFA (P < 0.05) including C10:0, C12:0, C14:0, and C16:0 (P < 0.05) as well as the greatest C16:1 (P < 0.05); pigs fed the DCO diet had most PUFA (P < 0.05) including C18:2n6, C18:3n3, C20:2, and C20:4 (P < 0.05) with the greatest IV (P < 0.05); and pigs fed the TW diet had most MUFA (P < 0.05) and C18:1 (P < 0.05) with the greatest C17:0, C18:3n6 and CLA (P < 0.05) in the belly fat. The pigs fed the DCO diet had lower C18:0 (P < 0.05) than those fed CS, TW, and CN diets and the pigs fed the DCO and CN diets had the lower C20:1 (P < 0.05) than those fed the CS and TW diets. The VE supplementation only affected concentration of C16:1 (P = 0.03) and C20:0 (P = 0.03) with a tendency for effect on C18:0 (P = 0.09) and CLA (P = 0.06) in the belly fat, wherein the concentration of C16:1 and CLA increased and the concentration of C18:0 and C20:0 decreased with increasing the dietary VE from 11 to 200 ppm.
Sex affected the FA profile of belly fat wherein gilts had more (P < 0.05) C17:0 and C20:4 than barrows. Interactions between sex and fat were detected on C12:0, CLA, and C20:2 in belly fat, wherein gilts had more (P < 0.05) C12:0 and C20:2 than barrows when they were fed the DCO diet and more CLA (P < 0.05) when they were fed the TW diet but less CLA when they were fed the CN diet. No interaction between sex and VE supplementation was observed on the FA profile in belly fat.
Fatty acid profile in the liver
As shown in Table 6, the interactions between dietary VE supplementation and fat source on FA profile observed in the liver were for C18:0, C18:2n6, and C22:5 (P < 0.05) in which increasing VE supplementation level decreased C18:0 when pigs were fed the CS and CN diets but not the TW and DCO diets and increased C22:5 when pigs were fed the DCO diet but not the other diets. Increasing VE supplementation level decreased C18:2n6 when pigs were fed the DCO diet even though that FA was greater in the DCO diet than the other diets.
Partly corresponding to the intake of FAs, but not completely, differences (P < 0.05) were detected among pigs fed dietary fat source on most FAs except C18:0, C18:3n3, CLA, C20:4, and C22:0. Among the four fat treatments, the highest content of SFA (P < 0.05) including C12:0, C14:0, and C16:0 (P < 0.05) and C20:5 (P < 0.05 except for the TW diet) was for pigs fed the CN diet. The highest content of PUFA (P < 0.05) including C18:2n6, C20:2, and C18:3n6 (P < 0.05) with the greatest IV (P < 0.05) was in pigs fed the DCO diet. The highest content of MUFAs including C16:1, C18:1, and C20:1 (P < 0.05) as well as C17:0 (P < 0.05) was for pigs fed the TW diet. Additionally, fat supplementation reduced concentration of synthesized unsaturated long-chain FAs including C22:4 and C22:5 in the liver of pigs fed fat-added diets compared to that of pigs fed the CS diet and there was less (P < 0.05) C22:6 in liver of pigs fed the DCO diet compared to that of pigs fed other fat-added diets. The VE supplementation only affected concentration of C17:0 and C18:0 (P < 0.05), with tendencies for C16:0 and C20:5 (P < 0.10), wherein increasing dietary VE from 11 to 200 ppm increased concentrations of C16:0, C17:0, and C20:5 but decreased concentration of C18:0.
Table 5. Effect of different fat source and Vitamin E (VE) supplementation on fatty acid profile (%) in belly fat1
A significant interaction between sex and fat was observed on C16:0 (P < 0.05) content in the liver wherein gilts fed the CN diet had greater C16:0 compared with gilts fed other diets while barrows fed the CS and CN diets had the most C16:0 compared with barrows fed other diets.
Plasma and tissue VE concentration
Table 7 shows the effect of different fat source and VE supplementation on VE concentration in plasma and tissue. As expected, increasing dietary VE supplementation from 11 to 200 ppm increased VE concentration in plasma for all phases, liver and loin muscle (P < 0.01). significant effects of dietary fat source were observed on VE concentration in plasma at the end of Phase 1 (P = 0.10) and Phases 2, 3, 4, and 5 (P < 0.05). At the end of Phase 2 to 5 (P < 0.05), the pigs fed the CS diet had lower plasma VE concentrations than pigs fed fat-added diets (TW, DCO, and CN); additionally, the pigs fed the CN diet had greater plasma VE concentrations than pigs fed the CS and TW diets in Phase 3 and the CS, TW, and DCO diets in Phase 4. No effect of dietary fat source was observed on VE concentration in liver and loin muscle at slaughter except for an interaction (P < 0.05) between fat source and sex where greater liver VE concentrations were observed in barrows than gilts when fed the TW and DCO diets.
Interactions between fat source and VE were observed on plasma VE concentration at the end of Phase 2 to 5 (P < 0.05; Table 8). Increasing VE supplementation level from 11 to 200 ppm increased plasma VE concentrations in pigs fed the TW and CN diets greater than pigs fed the CS and DCO diets. Pigs fed the DCO diet had the greatest plasma VE concentration from Phase 2 to 5 when supplemented with 11 ppm VE (P < 0.05) but when 200 ppm VE was supplemented, plasma VE concentrations were greatest in pigs fed the CN diet from Phase 3 and 4 and the CN and TW diets in Phase 2 and 5 (P < 0.05). Plasma VE concentration increased linearly (P < 0.05) with increasing time of feeding the 200 ppm VE diets; it increased faster (P < 0.05) in pigs fed the CN and TW diets compared to pigs fed the CS and DCO diets.
Table 6. Effect of different fat source and Vitamin E (VE) supplementation on fatty acid profile in the liver1
Antioxidant status
As shown in Table 9, the SOD activity in the plasma increased (linear; P < 0.05) with time. An interaction between fat source and VE supplementation was detected at the end of Phase 2, wherein pigs fed the CN diet had the lowest (P < 0.05) SOD activity among different dietary fat treatments when pigs were fed diets containing 200 ppm VE, while no effect was detected when dietary VE was 11 ppm. With that lone exception, no effect of dietary treatments including fat sources and VE levels was detected on the SOD activity in the plasma.
Additionally, no main effects were observed on liver antioxidants except liver SOD activity wherein pigs from the DCO treatment had greater liver SOD activity (P < 0.05) than those of the CN treatment. No interactions between dietary fat source and VE supplementation were observed on liver antioxidant status. A significant interaction between sex and fat source was observed on GSH and GSSG (P < 0.05) content in the liver wherein barrows had greater GSH than gilts when fed the TW diet and lower GSSG than gilts when fed the CS diet.
Discussion
As previously reported, the FA composition of pig adipose tissue reflects that of the diet because a portion of the FA is incorporated directly into the lipid tissues in pigs without further, or very little, modification (Averette Gatlin et al., 2002; Corino et al., 2002; Rentfrow et al., 2003; King et al., 2004). The FA in belly fat and backfat herein were principally of the SFA and MUFA groups and particularly C16:0, C18:0, C18:1, and C18:2n6.
In the current research, partly corresponding to the intake of different individual FAs, the pigs fed the CN diet had the most SFA, the pigs fed the DCO diet had most PUFA with the highest IV, and the pigs fed the TW diet had the most MUFA, in the backfat and belly fat. However, the content of C16:0 and C16:1 was the highest in the backfat and belly fat of the pigs fed the CN diet even though the intake of these two FAs was the highest in the pigs fed the TW diet. Additionally, the content of C20:2 in the backfat and belly fat of the pigs fed the DCO diet was the highest although its intake was the highest in pigs fed the CN diet. These departures of selected tissue results from the dietary intake indicate that the FA composition in adipose tissues may be actively modified from dietary FA intake, especially for those non-essential FA (Kloareg et al., 2007). Thus the tissue FA profile mostly, though not exactly, follows the dietary FA profile.
Table 7. Main effect of different fat source and Vitamin E (VE) supplementation on VE concentration1 in plasma and tissue
Table 8. Individual treatment effect of different fat source and Vitamin E (VE) supplementation on VE concentration1 in plasma and tissue
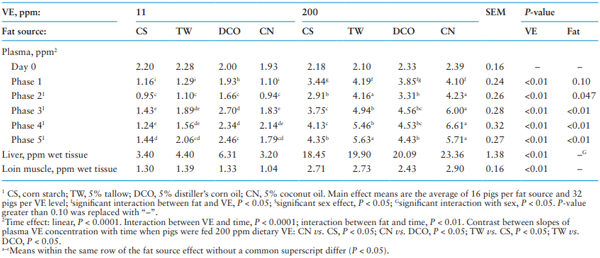
As the most common FA in grains and oilseeds, linoleic acid (C18:2n6), which is relatively low in animal fat but essential for animals, is one of the most widely evaluated FA. The changes in tissue composition of C18:2n6 with different dietary fat source followed the difference in the intake through diets, which agrees with Kloareg et al. (2007). In the present study, the intake of C18:2n6 was the highest in the DCO treatment among the four dietary fat treatments, 2.6 times as much as the intake in the CN treatment. As a result, the concentration of C18:2n6 in the backfat and belly fat of pigs fed the DCO diet was 2.9 and 2.8 times as much, respectively, as in those fed the CN diet. This similar relative ratio of increase indicated the concentration-dependent deposition of C18:2n6 in the adipose tissues. This result is in agreement with previous studies, where the proportion of C18:2n6 in subcutaneous adipose tissue and backfat of pigs increased from as low as 10% on a diet with 5% olive oil, which contains around 9.8% C18:2n6, to over 30% on a diet supplemented with 5% soy oil, which contains around 53% C18:2n6 (Averette Gatlin et al., 2002; Nuernberg et al., 2005; Apple et al., 2009a, b). Additionally, the extent of the change in C18:2n6 content in muscle and adipose tissues was always the greatest among all the FAs when supplementing unsaturated vegetable oils (such as safflower oil, soybean oil, corn oil) compared with animal fat (such as tallow and choice white grease) as a fat source for pigs (Mitchaothai et al., 2007; Corino et al., 2008; Apple et al., 2009a, b, c; Browne et al., 2013), which agrees with the result of the current study
Table 9. Effect of different fat source and Vitamin E (VE) supplementation on antioxidant status1 in pigs
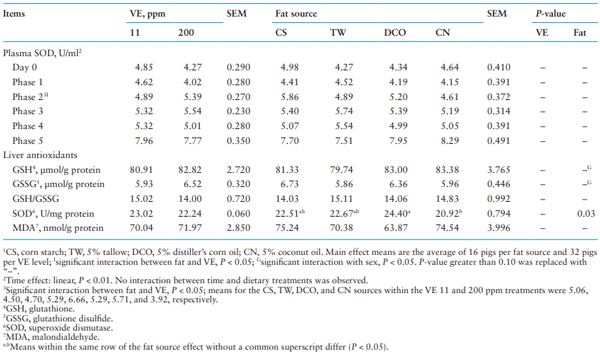
Oleic acid (C18:1) is the most abundant FA in adipose tissues of pigs, its concentration in tissues changes with different dietary fat sources in a relatively smaller range compared to linoleic acid C18:2n6 in this study. Although there was also a large difference in C18:1 intake among different fat sources with the highest intake in the TW treatment, which was 3.9 times as much as that of the CS treatment in the current study, the C18:1 concentrations in backfat and belly fat of the TW treatment was only approximately 6% higher that those of the CS treatment. Paton and Ntambi (2009) reported that the stearoyl-CoA desaturase (SCD) gene is responsible for catalyzing the conversion of SFA to MUFA and high C18:1 may inhibit its expression whereas high carbohydrate in the diet may stimulate its expression. Therefore, the highest intake of C18:1, one of the MUFA, in the TW treatment may reduce SCD gene expression and high carbohydrate content in the CS treatment may induce its expression resulting in a smaller difference in C18:1 content in the backfat and belly fat between the TW and CS treatments than expected although the TW treatment had much higher C18:1 intake (almost four times) than the CS treatment. Continuing this thought, while the intake of C18:1 in the CS treatment was the lowest, the lowest content of C18:1 in the backfat and belly fat was observed in pigs from the DCO and CN treatments. For the DCO, this result demonstrated that the high intake of PUFA probably inhibited the SCD gene expression resulting in limited conversion of SFA to MUFA (Ntambi, 1999; Paton and Ntambi, 2009). Allee et al. (1971) also reported that increasing the level of corn oil from 1 to 13% in the pig diet significantly decreased (60% to 70%) in vitro lipogenesis, although body fat content increased due to the increasing direct deposition of dietary fat. Świątkiewicz et al. (2021) reported that although a CN diet contained higher SFA but lower MUFA than the control group, the MUFA content in pork was similar between them that may result from the fact that C12:0 and C14:0, which are the highest SFA among all SFA in the meat of CN-fed pigs, were mainly from the diet but C16:0, C18:0 and MUFA were mainly from the biosynthesis in the animals. This means that dietary FA may hardly affect the C16:0 and C18:0 and MUFA content in pork although high SFA content in the diet may induce SCD gene expression.
Another highly abundant FA in adipose tissues of pigs is palmitic acid (C16:0), which changes to an even smaller extent than oleic acid (C18:1) and linoleic acid (C18:2n6) because this FA in the body may be mainly from biosynthesis (Świątkiewicz et al., 2021), but did change consistently when dietary fat sources changed. In this study, the intake of C16:0 was the highest in pigs fed the TW diet while lowest in pigs fed the CN diet among three dietary fat sources but the content of C16:0 in the backfat and belly fat was the highest in the pigs fed the CN diet. The C16:0 can be actively synthesized endogenously via de novo lipogenesis, and it is the end product of lipogenesis in the cytoplasm of cells. This result in the current study may be explained by the fact that the more UFA in the diets, the lower de novo synthesis of fat (Chen et al., 2021) and, then conversely, the lower the UFA the greater the de novo synthesis. Additionally, Mitchaothai et al. (2007) reported that C16:0 content in subcutaneous fat and backfat was higher with 5% TW supplementation than 5% sunflower oil supplementation which agrees with the current study in which the TW treatment had greater C16:0 content in backfat and belly fat than the CO treatment.
Another major FA comprising adipose tissues is stearic acid (C18:0), which also changes with variations in dietary FA profile, but to a much smaller magnitude than oleic acid (C18:1) and linoleic acid (C18:2n6) and a relatively larger extent than palmitic acid (C16:0). Different from the pattern of C16:0, the concentration of C18:0 was the lowest in the pigs fed the DCO diet where the intake of C18:0 was also the lowest among the three dietary fat sources. A similar result was previously reported when animal fats were compared to vegetable oils, significant differences were reported, with lower C18:0 content in adipose tissue of pigs in vegetable oil treatments compared to animal fat treatments (Apple et al., 2009a). Replacing 5% beef tallow with 5% sunflower oil decreased C18:0 from 13% to 11% in adipose tissues (Mitchaothai et al., 2007).
The variation in the concentration of the other PUFAs including C18:3n-6, C18:3n-3, and CLA in response to the dietary fat treatments generally followed the difference in the intake of the individual FAs. The increase in the deposition in the adipose tissue was mainly due to the increase in the intake of these FAs or their precurser FAs including C18:2n6, C18:3n-3, and CLA (Ramsay et al., 2001; Tischendorf et al., 2002; Kloareg et al., 2007).
The IV in backfat and belly fat displays similar trends with the FA profile in those adipose tissues and its intake, specifically the total PUFA and SFA, in which the highest IV value of backfat and belly fat was observed when pigs were fed the DCO diet, and the lowest IV value was observed in pigs fed the CN diet. The current results are in agreement with the previous documentation and reports of the close correlation between dietary FAs and pork IV (NRC, 2012; Kellner et al., 2016).
Regardless of the dietary treatment, the FA profile of the liver was different from adipose tissues. For example, the highest content of FA was C18:0 in the liver, instead of C18:1, which was highest in adipose tissues. Additionally, the higher concentrations of C20:4, which would result principally from conversion from C18:2n6 in the liver indicates the active metabolism and modifications of the essential FAs in the liver (Jump et al., 2005; Kloareg et al., 2007). These resulted in the higher content of PUFA and lower content of MUFA in the liver than the adipose tissues. Although there were differences between fat sources for most FA in the liver, and the profiles were generally closely related to the dietary FA intake, the relative composition of each FA was not decided by the intake itself. The intake of C12:0 in the CN treatment was almost 3-fold that of C14:0 and C16:0, while the liver concentration of both C12:0 and C14:0 was 1.5%, and the concentration of C16:0 was over 16%. This result is in agreement with previous studies that reported active FA modification including elongation and desaturation that occurred in the liver (Jump et al., 2005; Kloareg et al., 2007; Duran-Montgé et al., 2009). Despite this difference in FA composition in the liver, the FA profile changes did mimic in large part the difference in the dietary intake of the FA groupings similar to the response in adipose tissues. Overall, the pigs fed the CN diet had the highest content of SFA, the pigs fed the TW diet had the highest MUFA, and the pigs fed the DCO diet had the highest PUFA, in agreement with previous studies (Jump et al., 2005; Martin et al., 2008; Duran-Montgé et al., 2009).
The VE supplementation displayed very limited effects on the FA profile of adipose tissues. The VE supplementation only affected the concentration of C16:1, C18:0, C20:0, and CLA in the backfat and belly fat, where the concentration of C16:1 and CLA increased but the concentration of C18:0 and C20:0 decreased with the increasing dietary VE from 11 to 200 ppm. The results for CLA and C20:0, while exhibiting at least a statistical tendency, were of minor magnitude.
In the current study, VE concentration in plasma and tissues (liver and loin muscle) increased when dietary VE level increased from 11 to 200 ppm. Difference in plasma VE concentrations increased with the increasing duration of feeding diets containing 200 ppm VE compared with 11 ppm VE. As previous data demonstrated, the VE can be absorbed and deposited into different tissues readily, and the deposition increases linearly with increasing dietary supplementation (Monahan et al., 1990; Dove and Ewan, 1991; Yang et al., 2009; Lauridsen, 2010; Lauridsen et al., 2013). Regarding the interaction between VE and fat source in plasma VE concentrations, when the 200 ppm of VE was supplemented to fat added diets, plasma VE concentrations increased by the end of the study compared with the no-added-fat diet and plasma values increased faster and greater in pigs fed the CN and TW diets compared to pigs fed the CS and DCO diets. The interaction between fat source and VE on VE concentration in plasma is in agreement with previous studies, where the VE concentrations in plasma and tissues (liver, muscle, and adipose tissues) decreased when pigs were fed high PUFA diets containing linseed and safflower oil compared to the no-added-fat control diet and increased when pigs were fed high SFA diets such as olive oil and coconut oil (Prévéraud et al., 2014; Prévéraud et al., 2015). The MUFA content was also reported to be positively associated with tissue VE concentrations, due to the favorable role of oleic acid (C18:1) in the secretion of VE enriched chylomicrons (Prévéraud et al., 2015). The VE emulsified in medium-chain triglycerides has been demonstrated to have better gastrointestinal absorption compared to long-chain triglycerides (Gallo-Torres et al., 1978). The CN had the highest content of SFA with a high content of medium-chain FA, and the TW had the highest content of MUFA among the three fat sources. On the other hand, due to the higher digestibility of n-6 FA than other classes, the n-6 FA also enhance the deposition of VE in the tissues although most PUFA, especially n-3 PUFA, may reduce VE deposition (Prévéraud et al., 2015).
Contrary to the effect observed in plasma where increasing dietary VE supplementation from 11 to 200 ppm increased VE concentration in liver and loin muscle, an effect of fat source and an interaction between fat source and VE supplementation were not observed. However, in high VE treatments, the pigs fed the CN diet had numerically more VE in the liver by 26.6% and slightly more in loin muscle than pigs fed the CS diet within the 200 ppm VE treatments. Thus, the results in liver and muscle may agree to some extent with the interaction between fat source and VE observed in plasma.
The antioxidant system including nonenzymatic components (GSH, VE, Se, and vitamins) and a series of antioxidant enzymes (SOD, glutathione peroxidase [GSH-Px], and catalase [CAT]) can eliminate excessive oxidative radicals under stress condition (Lu et al., 2010). In the liver, pigs from the DCO treatment had higher SOD activity than pigs from the CN treatment, irrespective of VE supplementation. The highest content of free FA in the DCO used in the current study reflected the potential oxidation of this fat source, whereas the CN had the lowest content of free FA among the fat sources. Upon the intake of oxidized fat, liver antioxidant activity is reported to increase due to greater oxidative stress (Hung et al., 2017). However, the lack of difference in pigs fed either 11 or 200 ppm dietary VE in antioxidant activities in plasma and liver does not agree with previous studies that have reported improved enzymatic antioxidants system including SOD, CAT, and GSH-Px with increased VE supplementation (Lauridsen et al., 1999; Lauridsen, 2010; Cheng et al., 2017). This disparity might be partly due to a very well-controlled environment in the current study, which may have maintained a low total stress level. A further study with oxidative stress challenge might be helpful to better understand the potential interaction between fat source and VE supplementation.
Dietary VE supplementation did not affect FA profile in backfat, belly fat, and liver consistently, while dietary FA composition with different fat sources affected most of the FA profile in backfat, belly fat, and somewhat differently in liver. The high level of VE supplementation increased plasma, liver, and muscle VE concentrations and dietary fat sources affected plasma VE concentrations with an interaction with the VE supplementation level where the TW and CN increased the VE absorption and deposition greater than the DCO.
Acknowledgments
This work is supported by the National Institute of Food and Agriculture, U.S. Department of Agriculture, Hatch-Multistate Program (Project 2350937000) under accession number 1002298. The authors express appreciation to the National Pork Board, Fats and Proteins Research Foundation, and DSM Nutritional Products for additional financial support of this research. Special appreciation is expressed to Phibro Animal Health and Nutrition for ingredients used in the experiments. Appreciation is also expressed to D. Higginbotham and F. Berry for help in diet preparation, to K. Sparrow, W. Graham, and R. Elliott for care of pigs.
Conficts of Interest Statement
The authors declare no conflicts of interest.
This article was originally published in Journal of Animal Science, 2022, 100, 1–13 https://doi.org/10.1093/jas/skac184. This is an Open Access article distributed under the terms of the Creative Commons Attribution-NonCommercial License (https://creativecommons.org/licenses/by-nc/4.0/).