INTRODUCTION
Members of the genus avian adenovirus (AAV), from the family Adenoviridae, affect birds and are considered as important pathogens in the poultry industry. However, the importance of AAV as primary pathogens remains controversial because strains from the same serotype show variable pathogenicity (Okuda et al., 2006; Marek et al., 2010).
The fowl adenoviruses (FAdVs) belong to group 1 Aviadenovirus. These are further classified into 12 serotypes, 1-8a, 8b-11, of which some members are associated to certain diseases. Although implicated, the pathogenicity of most AAV, particularly FAdVs, is not well defined (Niczyporuk et al., 2012). Some strains may act as primary or secondary pathogens (Toro et al., 2000; Niczyporuk et al., 2012, 2013). However, under certain conditions, such as inclusion body hepatitis (IBH) and hydropericardium hepatitis syndrome (HPS), FAdVs have been directly involved, wherein disease has been replicated using field isolates (Okuda et al., 2006; Mase et al., 2010).
FAdV type-c serotype-4 (FAdV-4) is the causative agent of HPS (Mazaheri et al., 1998; Schonewille et al., 2008). Other FAdV strains may be able to produce HPS, together with IBH (Zhao et al., 2015). Both diseases affect the gastrointestinal tract (GIT), wherein the virus mainly exhibits tropism. However, the virus has also been detected in the cardiovascular, hematopoietic, and respiratory systems (Cheema et al., 1989; Erny et al., 1995; Nakamura et al., 2002).
Low egg production was observed in 2 layer flocks belonging to the same company in eastern Japan. Although FAdV-4 is thought to target the digestive organs, we isolated the virus from the oviduct of chickens from both farms. To our knowledge, its apparent affinity for oviduct tissue, and possible effect on egg production, has not yet been reported before. In the present study, the pathogenicity of these viruses isolated from chicken oviduct was examined, and the virus was molecularly characterized using the hexon and fiber-2 genes.
MATERIALS AND METHODS
Case History
The first case, Y farm, is in Ibaraki Prefecture in eastern Japan. This multistage farm follows all-in allout management. The affected flock was kept in a closed house containing approximately 81,000 heads of White Leghorn chickens. This farm had 8 flocks, which were kept separately, and had approximately 700,000 birds in total. The farm chickens showed a low egg production rate of 82% at 26 wk of age, which worsened over time. Eggshell malformation, which led to an increased incidence of breakage, was also observed. The second case, M farm, also located in Ibaraki Prefecture, is a multistage farm and follows all-in all-out management. The flock was kept in a closed house of approximately 90,000 heads of White Leghorn chickens. The farm had 6 lots in 3 houses and kept more or less 490,000 birds. This farm also observed declining performance; egg production decreased from 91% at 41 wk to approximately 82% at 44 wk of age, which did not improve over time.
Chicken Samples
To investigate the cause of poor egg production, serial sampling was conducted. Live birds were collected at different ages: 7 at 199 D of age, 6 at 209 D, 5 at 212 D, 6 at 223 D, and 6 at 290 D from Y farm; 10 at 378 D and 5 at 381 D from M farm. The birds were sacrificed by cervical dislocation and opened aseptically, and the internal organs were checked for lesions or abnormalities. Portions of the parenchyma and tubular organs such as the heart, liver, spleen, kidney, oviduct, ovary, lungs, and trachea, as well as lymphoid organs such as the bone marrow, spleen, and thymus, were collected and stored at −80°C for virus isolation.
Some portions of these organs were also used for bacterial examination and immersed in 10% formaldehyde solution for histopathological examination.
Molecular Detection by PCR PCR
tests were performed separately using pooled intestinal tract and oviduct samples to detect AAV; pooled parenchyma and respiratory organs for infectious bronchitis virus (IBV); pooled parenchyma and bone marrow for chicken anemia virus (CAV); and pooled intestinal tract and oviduct for EDSV. Samples were manually homogenized using a sterile mortar and pestle to a concentration of 30% with Hanks’ solution (Nissui Pharma, Tokyo, Japan) containing Penicillin-G (Meiji Seika Pharma Co., Ltd., Tokyo, Japan) at 400 U/mL and streptomycin (Meiji Seika Pharma Co., Tokyo, Japan) at 0.4 mg/mL. The mixture was centrifuged at 3,000 x g for 10 min. DNA was extracted using a QIAamp DNA Mini Kit (Qiagen, Hilden, Germany) in accordance with the manufacturer’s instructions. PCR was performed using KOD Dash (Toyobo Co. Ltd., Osaka, Japan) following the manufacturer’s instructions and using previously described primers and conditions with slight modifications: for AAV, the method followed by Mase et al., 2009; for CAV, Yilmaz et al., 2001; for EDSV, Begum et al., 2013; and for IBV, Mase et al., 2004 (Supplementary Table 1).
In the case of sample-inoculated chick kidney cells (CKC), the supernatant was used for DNA extraction. AAV-PCR was performed using KOD Dash as previously described (Mase et al., 2009).
Virus Isolation
The AAV-PCR–positive oviducts from each farm were used for virus isolation. Briefly, the oviducts were homogenized separately using a sterile mortar and pestle. The mixture was brought to a concentration of 30% with Hanks’ solution (Nissui Pharma, Tokyo, Japan) containing Penicillin-G (Meiji Seika Pharma Co., Ltd., Tokyo, Japan) at 400 U/mL and streptomycin (Meiji Seika Pharma Co., Ltd., Tokyo, Japan) at 0.4 mg/mL. The mixture was centrifuged at 3,000 x g for 10 min. The supernatant was filtered through 0.20 cellulose filters (Toyo Roshi Kaisha Ltd., Japan), and 1 mL was inoculated into 9 mL of CKC in Eagle’s Minimum Essential Medium solution (Nissui Pharmaceutical Co. Ltd., Japan). This contained 100 IU/mL Penicillin-G (Meiji Seika Pharma Co., Ltd., Tokyo, Japan), 0.1 mg/mL streptomycin (Meiji Seika Pharma Co., Ltd., Tokyo, Japan), and 1.25 mcg AmphotericinB (Fungizone 50 mg Amphoterecin-B Bristol-Myers Tokyo, Japan) with 7% fetal bovine serum (FBS) (Gibco, Life Technologies, N.Y., USA) at pH 7 to form 1% solution. The solution was dispensed into 25- cm2 cell culture bottles (Nunc Thermo Scientific Jiangsu, China) and incubated at 40°C in 5% CO2. The cultures were incubated at 40°C in 5% CO2 and observed for 2–3 D and then passaged into new cell culture bottles until a cytopathic effect was observed. The AAV KR5 strain was used as a positive control.
The TCID50 in CKC was determined through previously described methods with slight modifications (Rovozzo and Burke, 1973). Six-well plates with CKC were loaded with 900 mL of Minimum Essential Medium without FBS. One milliliter of virus fluid was sieved through 0.20-μm cellulose filters (Toyo Roshi Kaisha Ltd., Japan) and diluted 10-fold from 10−1 to 10−10. Hundred microliters of each dilution was loaded into each well of one plate, with one plate assigned for each dilution. All plates were incubated at 40°C in 5% CO2 and observed for cytopathic effect until 5 D. The endpoint dilution was computed using the Reed and Muench method (Reed and Muench, 1938).
Chorioallantoic membrane inoculation
Thirty-seven 9-day-old specific pathogen–free eggs (Nisseiken, Tokyo, Japan) were prepared: 11 for each virus strain and 4 as negative controls. The viruses were inoculated onto a chorioallantoic membrane (CAM), and the inoculated eggs were incubated at 37°C and checked daily by candling. After 5 D of incubation, the eggs were aseptically opened. The embryos and CAMs were examined for lesions or changes. Each embryo was laid on its left side with the beak perpendicular to the spine. The length was measured from the highest point of the head, or between the eyes when the head was tilted, to the tip of the tail; individual weights were also measured. For additional confirmation, 5 embryos and their corresponding CAMs were selected and checked by AAV-PCR, while the negative controls were pooled.
Nucleic Acid Sequencing
AAV isolates from both farms were used for genetic comparison; AAV KR5 was used as a reference strain. PCR was performed to characterize the hexon and fiber-2 genes of the field AAV strains using previously described primers and conditions (Mase et al., 2009, 2010). PCR products were purified by using a QIAquick Gel Extraction Kit (Qiagen, Hilden, Germany). The nucleotide sequences of the PCR products were determined using a Big Dye Terminator cycle-sequencing kit, version 3.1, (Applied Biosystems, Foster City, CA, USA) and ABI Prism 3130 Genetic Analyzer (Applied Biosystems). DNA products were sequenced from both directions.
The hexon gene sequence data reported in this study were submitted to GenBank under accession numbers MH492323–MH492325. The fiber-2 gene sequences are yet to be assigned accession numbers.
Nucleotide Sequence Analysis
Sequence assembly and editing were performed using Codon Code Aligner (version 3.7.1, Codon Code Corporation, Centerville, MA) and Clustal X (version 2.1, UCD Conway Institute of Biomolecular & Biomedical Research, Dublin, Ireland). Identity and homology were confirmed using Basic Local Alignment Search Tool (BLAST; National Library of Medicine, Bethesda, MD). Phylogenetic and molecular evolutionary analyses were based on the hexon gene and fiber-2 gene sequences using Molecular Evolutionary Genetics Analysis Software, version 6.0 (MEGA6; Tamura et al., 2013). Phylogenic analysis of nucleic acid sequences was performed using the neighbor-joining method with the Kimura 2-parameter model. The gene sequences were also compared and analyzed with the neighbor-joining method with Poisson correction. Bootstrap values (1,000 replications) are indicated on each tree. Relevant hexon and fiber-2 gene sequences available on GenBank were used for comparison.
Statistical Analysis
The statistical significance of differences between the 2 groups was determined using SigmaPlot 14.0 (Systat Software, Inc., San Jose, CA). When normality tests and equal variance tests were passed, the data were compared using the t test. If the normality tests or equal variance tests failed, the data were compared using the Mann–Whitney rank-sum test.
Pathogenicity
The pathogenicity of KR5, Japan/Ibaraki/M-HB2/ 2016, and Japan/Ibaraki/Y-H6/2016 was examined through artificial infection using previously described methods with slight modifications (Mase et al., 2010). Sixty 1-day-old specific pathogen-free (SPF) chicks (Nisseiken, Tokyo, Japan) were kept in an isolator with biosafety level 2, as registered in the Ministry of Agriculture, Forestry and Fisheries (MAFF). Fifteen birds, for each strain, were infected orally with 0.1 mL of viral fluid. The virus strains, with known titers, were diluted to 10−1 before inoculation. The remaining 15 birds were given 0.1 mL of Hanks’ solution as negative controls. The birds were observed for 14 D for clinical signs of HPS, after which all were sacrificed by atlanto-occipital dislocation. Necropsy was performed, and organs were collected. AAV-PCR and FAdV-4-PCR assays were performed separately on pooled organs (liver, heart, and kidney) and pooled GIT samples (duodenum, jejunum, and cecum) (Mase et al., 2009; Mase et al., 2010). In addition, 8 400-day-old nonvaccinated commercial hens were reared as mentioned previously. The hens were assigned to 2 groups and given viral fluid with known titers. The 2 groups were housed separately; 6 were given 0.2 mL of undiluted Japan/Ibaraki/Y-H6/2016 intravenous (IV), while 2 were given 0.2 mL of KR5 strain IV. Birds were reared and observed for clinical signs of HPS. At 5 D and 10 D postinfection (dpi), half of the birds from each group were sacrificed and necropsied and selected organs were taken for histopathology and further testing. AAV-PCR was performed on the pooled parenchyma organs (spleen, kidney, lung, and pancreas), liver, proventriculus, oviduct, and pooled GIT samples. All animal experiments were approved by the Animal Experiment Committee of Poultry Products Quality Control.
RESULTS
Necropsy
Necropsy of bird samples from both farms revealed no significant findings, although severe egg production damage and eggshell malformation were observed. No signs of microbial infection, on-going or convalescent, were observed in the macroscopic findings.
Figure 1. (A) Histopathological observations of oviduct samples from Y farm. Arrow indicates lymphocytic infiltration, 1000x. (B) Histopathological observations of oviduct samples from Y farm. Arrow indicates epithelial desquamation, 1000x. (C) CKC cultivation. M farm sample (eighth passage). Characteristic cell rounding, swelling, and monolayer detachment (arrows) were observed beginning at the sixth passage onwards. All cell cultures were incubated at 40°C in 5% CO2. (D) CKC cultivation. Negative control. Abbreviation: CKC, chick kidney cells.
Bacteria and Mycoplasma Isolation
Bacteria and Mycoplasma spp. isolation from the parenchyma, respiratory, and oviduct samples of both farms was negative.
Histopathological Examination
In histopathological examination of Y farm oviduct samples, the presence of moderate edema was confirmed. Leukocyte infiltration indicated a mild inflammatory reaction in the uterus portion of the oviduct. (Figures 1A, 1B and Supplementary Figure 1).
PCR Detection
The results of PCR are summarized in Table 1. AAV was detected in the oviduct samples from both farms using primers that detect all 3 AAV groups (Figure 2). In contrast, AAV was negative in the intestinal samples. In Y farm, CAV was positive in bone marrow samples, while the results in M farm were negative. Detection rates for CAV and AAV differed among differently aged birds. Parenchyma and intestinal samples from both farms showed negative results in EDSV-PCR, and parenchyma and respiratory organs were all negative by IBV-PCR.
Figure 2. Avian adenovirus-PCR results. Lane-M, 100-bp Ladder; PC, KR5 positive control (800-bp); NC, negative control; Lane 1, Y farm sample; Lane, 2 mol farm sample.
Virus Isolation
At the fifth blind passage of oviduct samples in CKC, AAV was detected in the supernatant of samples from both farms by PCR, but the bands appeared weak and a cytopathic effect was not observed. At the sixth passage, cell swelling, cell death, and monolayer detachment were observed (Figures 1C–1D). The results for both AAV-PCR and cytopathic effect observations were greatly improved in the seventh and eighth passages. Each virus strain was designated as Japan/Ibaraki/MHB2/2016 and Japan/Ibaraki/Y-H6/2016.
CAM Inoculation
Although gross examination of the virus-inoculated CAMs revealed no noticeable changes, embryos inoculated with the 3 virus strains consistently showed signs of stunting and hemorrhage (Supplementary Figure 2). As shown in Table 2, 1 of 11 (9.09%) embryos inoculated with isolated viruses or KR5 strain died. The length and weight of surviving inoculated embryos were less than in the negative control. There was a significant difference between virus-inoculated embryos and the negative control (P ≤ 0.05) as per the t test or Mann–Whitney rank-sum test.
The AAV-PCR results of selected embryos were as follows: for Japan/Ibaraki/Y-H6/2016, 1 of 5 (20%); for Japan/Ibaraki/M-HB2/2016, 3 of 5 (60%); and for KR5, 2 of 5 (40%), showing weakly positive results. Pooled negative controls showed negative results in both assays. The AAV-PCR results of the corresponding CAMs were as follows: for Japan/Ibaraki/Y-H6/2016, 5 of 5 (100%) positive; for Japan/Ibaraki/M-HB2/2016, 5 of 5 (100%) positive; and for KR5, 5 of 5 (100%) positive. Pooled negative controls showed negative results.
Nucleotide Sequence Analysis
Based on the nucleotide sequence of the hexon gene, both isolates belonged to FAdV-4 (Figure 3). The 2 strains showed 99.29% nucleotide sequence similarity with each other. The closest AAV strains registered in GenBank are the KR5, ATCC VR 829, MX-SHP95, and ON-1 strains. Japan/Ibaraki/Y-H6/2016 isolated from Y farm, with accession number MH492323, showed 99.15% similarity with KR5. Japan/Ibaraki/M-HB2/ 2016 isolated from M farm, with accession number MH492324, was 99.57% similar to KR5. Both isolates exhibited 99.0% similarity with the VR 829, MXSHP95, and ON1 strains. Analysis of the fiber-2 gene sequence confirmed that the isolated viruses were indeed FAdV-4 and that they were closely related to the Da60, KR5, and ON1 strains (Figure 4).
TCID50 in CKC and Pathogenicity
After 5 D incubation in CKC, the computed titers of KR5, Japan/Ibaraki/M-HB2/2016, and Japan/ Ibaraki/Y-H6/2016 were 105.25TCID50/mL, 105.40TCID50/mL, and 106.75TCID50/mL respectively. After 14 D of observation, none of the oral-inoculated chicks developed signs of HPS. All of them were sacrificed by atlanto-occipital dislocation and necropsied. At necropsy, all birds did not have lesions indicative of HPS (Table 3). The results confirm that the field strains were indeed nonpathogenic. AAV-PCR results of pooled parenchyma organs and GIT organs were all also negative. Adult hens infected with Japan/Ibaraki/YH6/2016 IV did not show clinical signs at 5 and 10 dpi; necropsy findings also did not indicate HPS. 3 of 3 (100%) pooled GIT samples were AAV-PCR positive at 5 dpi, and 1 of 3 (33.33%) was positive at 10 dpi (Table 4). On histopathological examination, aside from minimal leukocytic infiltration in the oviduct (Figure 5), there were no other significant observations.
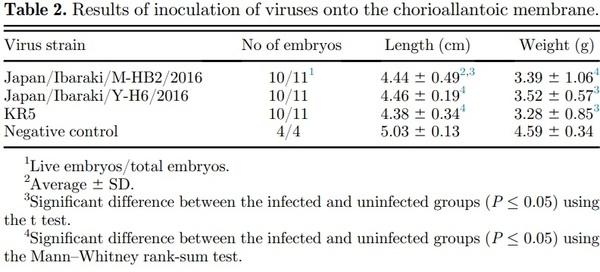
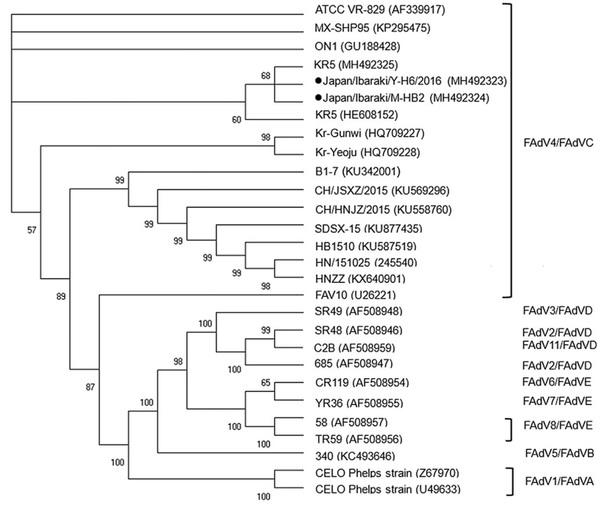
Figure 3. Phylogenetic analysis of the hexon genome of the field avian adenovirus strains. Bootstrap values (1,000 replications) are indicated in each tree. KR5 strain positive control is also shown.
DISCUSSION
The sudden occurrence of abnormal egg production on 2 layer farms (Y and M farms) was investigated. Low egg production and increased incidence of breakage were the main problems. Bacteria isolation and Mycoplasma spp. isolation both were negative, and necropsy findings were insignificant. The absence of clinical signs, nor increased mortality, and negative results in PCR for EDSV and IBV ruled out these pathogens. Histopathological examination of selected organs showed insignificant results, except in the oviducts. Microscopy of the oviducts revealed signs of edema and leukocyte infiltration; indications of mild inflammatory reaction. PCR assay for AAV was positive in both farms, and the virus was isolated from oviduct samples collected from each farm. Furthermore, CAV was detected in samples from Y farm by PCR. Based on the nucleotide sequence of the hexon gene, the 2 isolates belonged to FAdV-4.
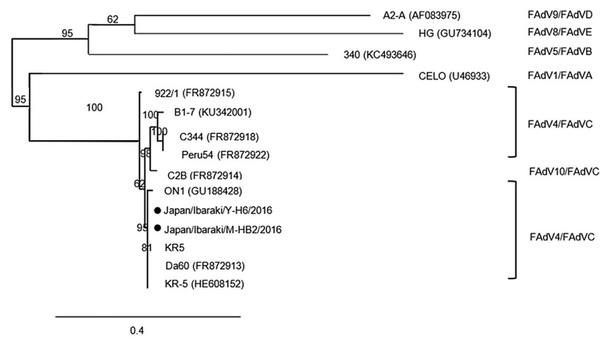
Figure 4. Phylogenetic analysis of the fiber-2 genome of the field avian adenovirus strains. Bootstrap values (1,000 replications) are indicated in each tree. KR5 strain positive control is also shown.
Hydropericardium hepatitis syndrome, caused by FAdV-4, occurs predominantly in young broilers and is characterized by the sudden onset of high mortality rates of 30 to 60% (Cheema et al., 1989; Zhao et al., 2015). In some instances, adult broiler breeders have been afflicted, wherein mortalities reached up to 6.4% (Abe et al., 1998). In the present study, 2 strains of FAdV-4 were isolated from oviduct samples of aged chickens. The affected chickens exhibited no significant lesions and only showed poor egg production. As these findings are conflicting with published data, these strains may have developed affinity for oviduct tissue, a characteristic similar to EDSV. Another possibility is that the viruses are undergoing latency in the oviduct tissue; vertical transmission also occurs in FAdVs (Toro et al., 2001).
Niu et al., (2019) described the effects of pathogenic FAdV-4 on the heart, lungs, and kidneys. Pericardial effusion developed in the heart, while edema was observed in the lungs and kidneys. Evidently, FAdV-4 can induce inflammatory reactions, which can lead to increased vascular exudation, resulting in cardiac effusion and edema (Niu et al., 2019). Similar inflammatory processes occurred in the oviducts of Y farm birds. Histopathological examination of the uterus portion indicated mild inflammatory reaction and edema. This may have been enough to alter the oviduct and its function (Van Eck et al., 1978).
Inoculation of embryonated eggs, through the CAM route, showed that the isolates have limited effects on chick embryos. The main lesions were stunting and cutaneous hemorrhage. Although the mortality rate was low, poor embryo growth was observed. The lengths and weights of virus-inoculated embryos were significantly low. The effects of the isolated viruses and KR5 were similar. In the PCR assay, a few random embryos were positive, showing weak bands. These results indicate the presence of very low levels of viral DNA. This may be because the virus cannot effectively penetrate the CAM; alternatively, the virus may have no affinity toward developing embryos. In contrast, PCR assays of random CAM samples were all positive with markedly strong bands, indicating high viral loads. The CAM is a double membrane; thus, compounds must pass across the surface epithelium and reach the vessels in the stroma (NowakSliwinska et al., 2014). It is possible that viral penetration was weak and localized, which may explain the differences in the PCR results. These results confirm that AAV, specifically FAdV-4, can survive inside infected eggs. The virus can be transmitted vertically; if a breeder hen was a carrier, infection can be passed on through the egg. A 9.09% fatality rate on infected embryos could mean a considerable loss in chick production. Furthermore, stunted embryos could lead to undersized chicks. Therefore, it appears that nonpathogenic FAdV-4 may have negative impacts on laying and breeder operations.
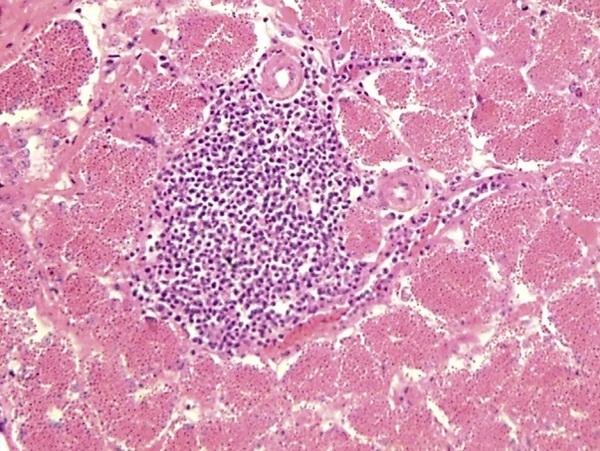
Figure 5. Histopathological observations of oviduct samples of hen infected IV with Japan/Ibaraki/Y-H6/2016. Arrow indicates lymphocytic infiltration, 400x. Abbreviation: IV, intravenous.
Artificial infection of SPF chicks and commercial adult hens did not result in HPS. AAV was detected by PCR from the adult hen GIT at 5 dpi. However, detection rate was lower at 10 dpi. Following that trend, it is likely that the virus will soon be undetectable, as was observed in chicks at 14 dpi. Cook (1983) described that the KR5 strain is recoverable from the GIT of infected chicks until 21 D and until 4 to 11 D from other organs. In the present study, the viruses were undetectable from both parenchymatous organs and the GIT of the infected chicks at 14 dpi. A possible reason is that, under controlled conditions, the strains are unable to maintain the infection. In the case of the IV-infected hens, the viruses were detectable from pooled duodenum, jejunum, and cecum samples only. Fowl adenoviruses show affinity for organs in the GIT such as the pancreas (Nakamura et al., 2002); also, viral loads are highest in the liver (Li et al., 2018), proventriculus, caecum, and ileum (Okuda et al., 2006; Hess, 2017; Matczuk et al., 2017). In the present study, the pancreas and proventriculus were PCR-negative. Perhaps the nonpathogenic strains do not have strong affinity for the said organs, although these can infect the intestines. The oviduct of infected hens were also PCR-negative, and histopathological findings were unremarkable. Possibly, under field conditions, other factors such as concurrent infections and environmental stressors contribute to maintenance of infection in both young and adult birds.
Y farm and M farm experienced poor egg production starting at 26 and 44 wk of age, respectively. The difference in the time of onset could be due to concurrent infection with CAV. Chicken anemia virus may have influenced the course of the disease as a contributing pathogen, affecting the immune response (Markowski Grimsrud and Schat, 2003), or via secondary effects due to anemia (Toro et al., 2000; Niczyporuk et al., 2013). The additive effect of CAV with FAdV-4 has been investigated, and their synergism was implicated in severe disease (Toro et al., 2000). It is possible that because of weakened resistance, viral replication was uninhibited, resulting in epithelial damage and effects on oviduct function. But even without CAV, FAdV-4 can cause immune suppression (Schonewille et al., 2008). The presence of FAdVs may also cause intestinal imbalance and nutrient malabsorption as well; this may contribute to poor egg production (Matczuk et al., 2017). These effects may have occurred alone or in combination
Nucleotide sequencing of the hexon gene revealed that the isolated viruses were classified as FAdV-4. The isolates showed a similarity of 99.29%. Furthermore, one of the isolates, Japan/Ibaraki/M-HB2/2016, was found to be closely related to the KR5 strain with a similarity of 99.57%. Notably, the KR5 strain is nonpathogenic. Analysis of the fiber-2 gene sequence confirmed that the isolated viruses were closely related to nonpathogenic strains such as Da60, KR5, and ON1 (Hess, 2000; Griffin and Nagy, 2011). Furthermore, the isolated viruses differed from Peru54 and C344, both of which were isolated from HPS cases (Marek et al., 2012).
Although the closest relative is a nonpathogenic strain, the field strain still caused damage. The slight difference in genetic makeup may have conferred some pathogenic properties which are absent in KR5. Differences in open reading frames (ORF), such as having a truncated ORF, has been observed in more virulent strains (Ye et al., 2016), even in cases of very close homology. Amino acid mutations have also been detected in pathogenic strains as compared with their nonpathogenic counterparts (Liu et al., 2016). Variances in ORF or the presence of mutations in the genome of Japan/ Ibaraki/Y-H6/2016 may have resulted in the observed behavior of this strain. Unfortunately, differences in ORF or amino acid mutations among the strains were not verified. For that reason, we are unable to make inferences regarding this. In the future, additional studies must be performed to resolve these questions.
In the present study, we isolated 2 strains of FAdV-4. To our knowledge, this could be the first report of FAdV4 isolation from the oviduct. Although the effect on the oviduct and egg production was not confirmed, a different effect was observed: stunting and death in SPF embryos. Nevertheless, more studies are needed to further describe the viral effects on egg quality, on chick quality, and its epidemiology. Fowl adenovirus type-c serotype-4 may be circulating unnoticed within Japanese layer farms and causing damage to breeders or layers. Careful attention should be given when a sudden drop in egg production occurs even without marked changes in the birds.