DESCRIPTION OF PROBLEM
There are growing concerns that the continual feeding of inorganic P is leading to the development of eutrophication of surface waters. Excessive P runoff from soil causes an accumulation of P in water sources, and this can result in toxic algal blooms and fish kills [1]. The availability of P from feedstuffs of plant origin is low for poultry because a greater proportion of the total P is present in the phytate form, a form that has low availability [2, 3]. Many researchers have reported that the availability of P and other minerals, such as Zn, Cu, and Fe, can be increased through addition of phytase (PHY) enzyme to poultry diets, which hydrolyzes phytate [4, 5]. In addition to its mineral availability properties, PHY has been shown to decrease P excretion and reduce P-linked pollution in intensive poultry production [6].
Originally, organic acids used to be incorporated in animal diets as a fungistat [7]. Some organic acids are also very efficacious in improving growth performance and phytate-P utilization in poultry [8–10]. It is thought that organic acids improve growth by regulating the intestinal microbial flora in the gut, allowing the development of commensal bacteria and reducing pathogenic bacteria that can produce toxins [11]. Citric acid (CA) is an organic acid that has been postulated to improve FE and increase the retention of P in broiler chicks [10, 12]. One of the possible mechanisms by which CA may benefit birds is by creating an acidic environment in the gut that favors the development of Lactobacillus and thus that helps reduce the susceptibility of birds to disease [10, 13].
Dietary Cu supplementation at prophylactic levels (> 250 ppm of the diet) was reported to have beneficial effects on poultry growth [14]. Some researchers have demonstrated that supplementing broiler diets with high dietary Cu regulates intestinal microbiota through its bactericidal or bacteriostatic functions [15–17]. However, Aydin et al. [18] reported that supplementing 250 ppm of Cu from Cu proteinate had no significant effect on the numbers of pathogenic bacteria in the ileum or on the carcass. Banks et al. [19] indicated that supplementing the diet with prophylactic concentrations (> 62.5 ppm) of Cu sulfate reduced P retention in broiler chicks. The reduction in P retention has been attributed to a reduction in the hydrolysis of phytic acid by increasing dietary concentrations of Cu [20]. However, studies on the adverse effects of high dietary Cu on P retention are limited to Cu sulfate and several other Cu compounds, such as tribasic Cu chloride. To our knowledge, none has evaluated Cu proteinate in broilers.
Until now, no information has been available regarding whether, and in which way, the feeding of Cu at prophylactic concentrations (250 ppm) and dietary additions of CA and PHY might influence the growth performance and retention of minerals in broilers. The objective of this experiment was to study the influence of dietary Cu, CA, and PHY on live performance and mineral retention of broiler chickens fed a low available P (aP) diet.
MATERIALS AND METHODS
The experiment described herein was approved by the Faculty of Veterinary Medicine Animal Care and Use Committee at Istanbul University.
Experiment 1
The purpose of this study was to assess the floor pen performance and carcass traits of broilers consuming low-aP diets supplemented with Cu, PHY, and CA.
Birds, Feeding, and Management. One-day old straight-run Ross 308 chicks were obtained from a commercial hatchery [21] and housed in an environmentally controlled floor pen facility. At 1 d of age, 10,080 chicks were randomly allocated to 48 pens (each pen containing 210 chicks) and fed a commercial pelleted-crumbled broiler starter diet [21] with an aP content of 0.45% until 13 d of age (Table 1). Birds were reared from 13 to 42 d of age on experimental diets in 3 phases (i.e., 13 to 28 d, 28 to 35 d, and 35 to 42 d). After a 13-d trial, aP was formulated at 0.25% in the diet for all 3 phases (Table 1). At 13 d of age, all the chicks were weighed and legbanded, and pen averages were adjusted so that no significant difference among pen weights was realized. Broilers were raised at a stocking density of 13 birds/m2, and wood shavings were used as litter. A commercial standard lighting regimen was used (23L:1D, 1 to 7 d; 20L:4D, 7 to 28 d; 23L:1D, 28 to 42 d). The lighting regimen was provided by incandescent lights with intensities of 30 lx from 0 to 7 d, 15 lx from 7 to 28 d, and 5 lx for the remaining period. The experiment was designed with a 2 × 2 × 2 factorial arrangement of treatments consisting of 2 dietary supplemental Cu concentrations (0 and 250 ppm), 2 levels of dietary PHY (0 and 750 phytase units/kg of diet), and 2 dietary CA levels (0 and 3%). This resulted in 8 dietary treatments with 6 replicate pens of each treatment. The Cu was supplied as Cu proteinate [22]. After analyses of duplicate feed samples, Cu concentrations for starter, grower, and finisher diets for the broiler chicks were found to have 10.40, 10.50, and 9.90 ppm of Cu, respectively. The source of PHY used in the experiment was Ronozyme P CT [23]. The CA was supplied as a food-grade product [24]. Copper, PHY, and CA were added accordingly to each diet at the expense of sand to meet the formulation specifications noted in Table 1. The birds had ad libitum access to water and mash feed that met or exceeded the NRC [25] recommendations for all nutrients, with the exception of the aP and Ca levels. The Ca level (0.70 to 0.90%) in the experimental diets was below NRC [25] recommendations to prevent possible growth reduction attributable to a high Ca:P ratio. Formulated and measured concentrations of these nutrients are presented in Table 1. At d 42 of the feeding trial, 24 chickens per treatment [4 per pen (2 male and 2 female), total of 192 chickens] were slaughtered in a commercial processing plant by severing a jugular vein.
Analysis of Feed. Samples of feeds were analyzed for DM and CP, crude ash, acid-insoluble ash, Ca, P, and Cu. Crude protein was determined using the Kjeldahl total N method, in which a sample was digested with sulfuric acid in a digester [26] and then organic N was transformed to ammonium sulfate, which was distilled in alkali conditions in a distillation apparatus [27]. Protein content was calculated by multiplying the total N content by 6.25. Dry matter was determined by oven drying for 24 h at 105°C, and crude ash was calculated by combustion at 550°C for 6 h [28]. Acid-insoluble ash content was determined in the feed and excreta samples after drying for 24 h and ground through a 0.5-mm screen using a Universal mill [29]. Acid-insoluble ash was determined according to methods described by Van Keulen and Young [30]. The amount of Ca (method 968.08) was analyzed by AOAC [28] procedures in feed samples. Total P was determined colorimetrically using a wet ash procedure, as described by Sands et al. [31]. Feed level of Cu was measured by flame atomic absorption spectrophotometry [32].
Analysis of Blood. Blood samples were collected from a jugular vein during slaughter. Serum was separated by centrifugation (3,000 × g, 20 min, 4°C) and used to measure total cholesterol, high-density lipoprotein (HDL) cholesterol, and triglycerides. Serum samples were analyzed enzymatically for cholesterol [33], triglycerides [34], and HDL cholesterol [35] using commercially available reagent kits.
Analysis of Tibia and Thigh. Right tibias were collected after slaughter and weighed after soft tissue on the tibias was removed. The tibias were boiled for 15 min to remove adhering muscle and cartilage. They were then dried for 16 h at 100°C, defatted by refluxing with diethyl ether for 6 h in a Soxhlet extractor [36], dried to a constant weight in a 100°C oven for 16 h, and analyzed gravimetrically for ash content after ashing in a muffle furnace at 550°C for 24 h. The tibias were analyzed for ash, Zn, and P. The ash weight of the tibias was recorded and used for the calculation of Zn and P contents of the tibias. Tibia Zn concentration was determined according to the method of Lan et al. [37], and P concentration was determined according to the method of Pearson [38]. The right thigh muscle was collected from 24 birds from each group (192 in total) and cholesterol levels were determined. Thigh muscles (biceps femoris) without skin and adipose tissue were collected from approximately the same location, washed, chopped, and ground. The samples were then stored at −18°C. Total lipid in muscle samples was extracted according to the method described by Elkin and Rogler [39]. Briefly, approximately 1 g of muscle was homogenized with 12 mL of 2:1 chloroform:methanol (vol/ vol) by a high-speed blender [40] and the homogenate was filtered through a Whatman No. 1 filter paper [41] into a 50-mL volumetric flask. After homogenization and filtration, samples were diluted to a final volume of 50 mL using 2:1 chloroform:methanol (vol/vol). The chloroform:methanol was then removed by evaporation under N2 at 30°C. Samples were centrifuged (1,000 × g for 10 min) and filtered through a Whatman No. 1 filter paper [41]. Finally, the dried extract was reconstituted in 5 mL of 2:1 chloroform:methanol (vol/vol). Total cholesterol in thigh muscle samples was analyzed colorimetrically using the enzymatic procedure described by Allain et al. [42].
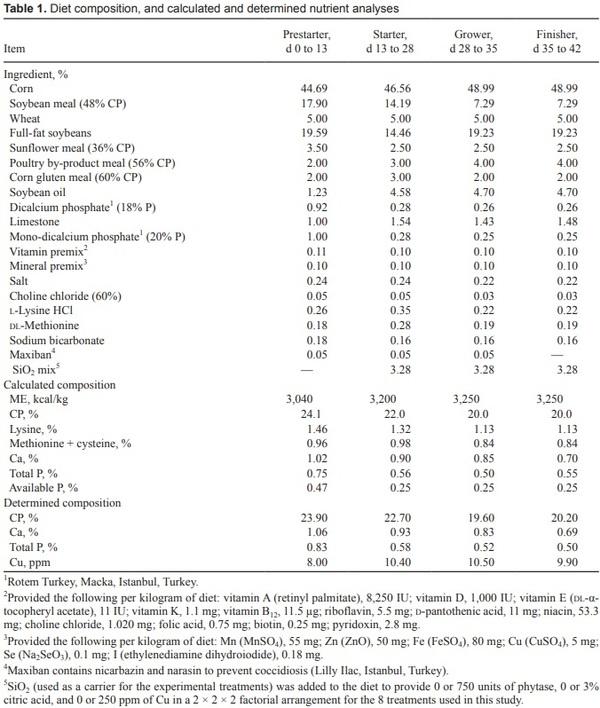
Experiment 2
The purpose of this study was to assess the retention of Cu and P in broilers consuming diets identical to those in experiment 1. At 42 d of age, 64 chickens from experiment 1 were transferred to metabolism cages; there were 4 replications per treatment, with 8 treatments and 2 birds per replicate. The metabolism cages were 20 × 40 × 35 cm (width × length × height). After 2 d of adaptation to the environment, birds were fasted for 24 h to empty gut digesta and then allowed ad libitum access to feed and water.
Total excreta were collected for 3 consecutive days. All feathers were carefully removed from the excreta by hand, and the excreta were sealed in sterile plastic bags and immediately frozen at −20°C until analysis. The thawed excreta were thoroughly mixed, weighed, and dried for 24 h in an oven (80°C). These samples were then ground to pass through a 0.5-mm sieve using a mill grinder [29] before determination of DM, Cu, and P. Dry matter content was determined on ground samples by oven drying at 100°C for 24 h [28]. Dry samples of excreta were ashed at 550°C in a muffle furnace, and the remaining ash was extracted with a 3 N nitric acid:HCl mixture (5:1, vol/vol). Acid-insoluble ash was determined according to the method of Scott and Boldaji [43]. Phosphorus was determined colorimetrically [31], and Cu concentration was determined by atomic absorption spectrophotometry [28]. Retention of Cu and P was determined by the difference from acid-insoluble ash in the excreta and feed and by total Cu or P in the excreta and feed [20].
Statistical Analysis
The experimental design for this study was a 2 × 2 × 2 factorial arrangement of treatments (2 Cu, 2 PHY, and 2 CA levels), with 6 and 4 replicates per treatment in experiments 1 and 2, respectively. Data from both experiments were analyzed using the GLM procedure of SAS software [44], with the main effects of Cu, CA, and PHY and their 2- and 3-way interactions included in the model. The following model statement was used: Yijkl = M + Pi + Cj + Sk + PCij + PSik + CSjk + PCSijk + eijkl, where Yijkl is the response measured, M is the overall mean, Pi is the effect of PHY, Cj is the effect of Cu level, Sk is the effect of CA level, PCij is the interaction between PHY and Cu, PSik is the interaction between PHY and CA, CSjk is the interaction between Cu and CA, PCSijk is the interaction among Cu, CA, and PHY, and eijkl is the residual error. The differences among mean values were identified by the least squares means procedure [45]. When no interaction between variables was found, they were excluded from the model. For each parameter assessed, pen was the experimental unit in both experiments. Mortality data were analyzed after arcsine square root transformation [46]. Statistical significance was established at P ≤ 0.05.
RESULTS AND DISCUSSION
Live Broiler Performance and Carcass Characteristics
The effects of dietary Cu, CA, and PHY on live performance are shown in Table 2. Dietary PHY supplementation did not affect broiler live performance during the 13- to 42-d period. Body weights at 42 d and BW gain were significantly influenced by both CA and Cu supplementation, with a significant interaction between the 2 dietary supplements (P < 0.05), in which birds fed supplemental CA had improved BW gain when fed the Cu-supplemented diet (Figure 1). The main effects of dietary CA and Cu on 42-d BW and BW gain showed that addition of CA and Cu significantly increased BW and BW gain, with the feed intake of CA-supplemented diets being greater than those of diets not supplemented with CA. Citric acid supplementation at 3% also improved FCR (P < 0.01) compared with nonsupplemented diets. However, there were no significant interactions among dietary additives on FCR at any time during the experiment. Treatment did not have a significant effect on mortality in the current study (overall mortality was 2.53%).
Hot carcass and tibia weight were increased (P < 0.05 for each main effect) by PHY supplementation (Table 3). Tibia weight and P were also increased by CA supplementation (P < 0.05). The main effects of CA and Cu supplementation on tibia ash showed that addition of CA and Cu significantly increased the tibia ash. Additionally, there was a significant Cu × CA interaction (P < 0.05) on tibia weight, in which Cu seemed to have an antagonistic effect on CA (Figure 2). Tibia P content was increased with CA supplementation (P < 0.05). There was a Cu × PHY interaction for tibia ash and tibia P contents (P < 0.05) in that supplementation with Cu did not reduce tibia ash and P contents regardless of PHY supplementation. Although tibia ash content was highest in birds fed diets supplemented with both Cu and PHY, tibia P contents were not the highest for birds fed diets supplemented with PHY and Cu, respectively (Figure 3 and 4). There was a PHY × CA interaction for tibia ash (P < 0.01) in that tibia ash was increased by both PHY and CA supplementation. However, the effect of CA and PHY was less than additive because the actual combined effect was less than the 2 individual effects added together (Figure 5). Tibia Zn content was not affected by dietary supplementation.
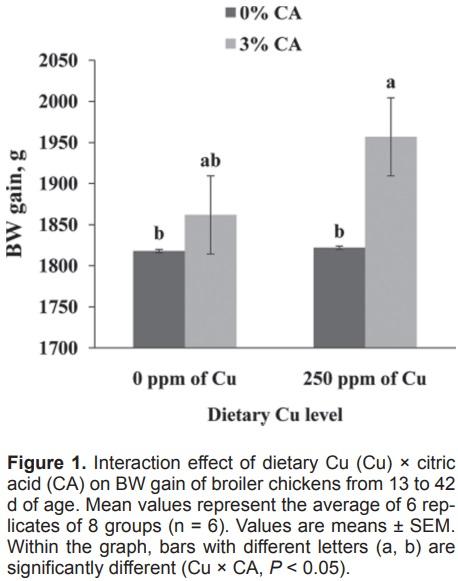
Microbial PHY supplementation influenced (P < 0.05) thigh weight, with birds fed PHY-supplemented diets having heavier thigh weights than birds not fed PHY-supplemented diets (Table 4). There were no significant main effects of dietary CA and Cu on thigh weight, P content, and Zn content of thigh meat. No treatment interaction was observed for thigh weight and thigh meat measurements.
Cholesterol Metabolism
Total serum cholesterol and thigh meat cholesterol contents were significantly affected by dietary Cu supplementation (Tables 4 and 5). Birds fed the high-Cu diet had significantly (P < 0.05) lower total serum cholesterol levels than those fed diets without added Cu, with differences of 22.44 mg/dL of total cholesterol. The thigh meat cholesterol content of birds fed the Cu-supplemented diet was decreased significantly (P < 0.05), with differences of 12.04 mg/100 g of cholesterol. No main effect or interaction effects of dietary Cu addition were observed for serum HDL cholesterol or triglyceride levels. There were also no significant effects of dietary PHY, CA, or their interaction on total serum cholesterol, HDL cholesterol, and triglyceride levels.
Retention of Minerals
Broilers fed the PHY-supplemented diet had significantly (P < 0.05) lower levels of P in the excreta compared with birds fed a diet without PHY (Table 6). Supplementing a low-aP diet with microbial PHY also increased the retention of P (P < 0.01). Phosphorus retention as a percentage of P intake increased significantly with the addition of dietary CA (P < 0.05). There were no significant main effects of dietary PHY and CA supplementation on the retention of Cu. Copper retention increased significantly (P < 0.001) with the addition of dietary Cu. No interaction effects were observed in the mineral retention portion of this study.
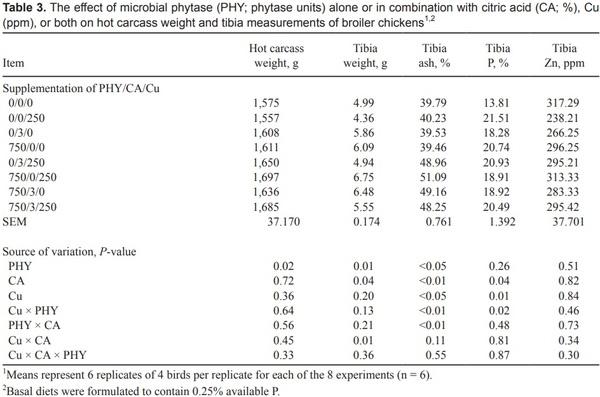
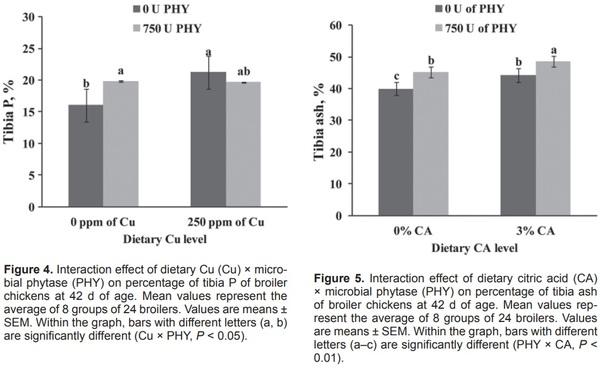
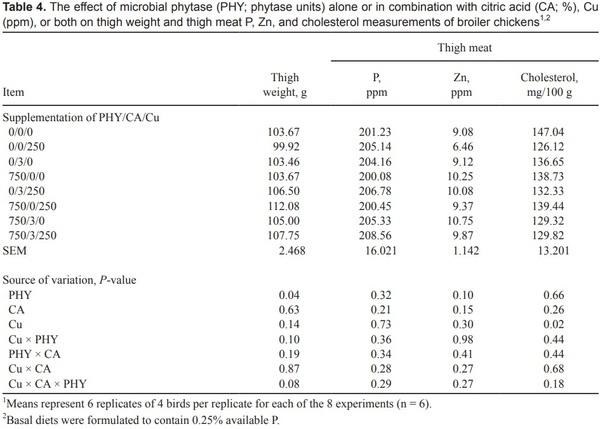
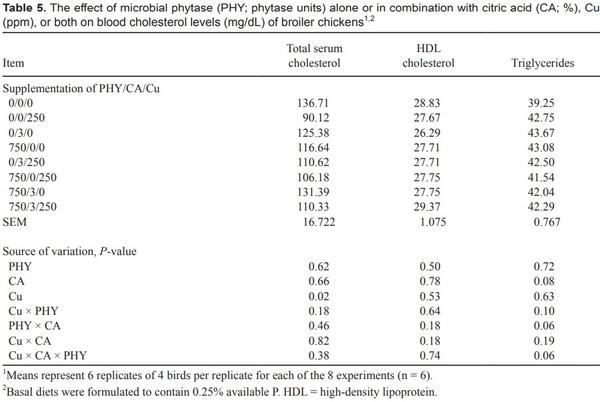
Nutritional interest in CA has increased greatly in light of its capacity to improve P use in poultry. The greater BW gain and improvement of FCR with addition of CA was likely due to the improved digestibility of minerals. There was a Cu × CA interaction in which the BW gain of birds was increased by supplementation with both Cu and CA. This interaction could mean that in the current study, CA supplementation in addition to Cu supplementation could contribute to using P effectively and eliminating the possible negative effect of using a low-aP diet. The increased growth response obtained from 3% CA in this experiment is in agreement with the results of Snow et al. [47], who found that the addition of dietary CA improved the BW gain and FE of broiler chicks fed a diet with 0.13% aP. Similar results on feed consumption and FE have been observed for the addition of CA to broiler diets [10, 48]. Other researchers have also reported an increase in tibia ash by supplementing a low-aP diet similar to ours with CA [47, 49]. The mechanism of action of CA on P availability is not well defined. It has been speculated that CA could bind Ca, with a concomitant reduction in their inhibitory effects on intestinal phytic acid hydrolysis [50].
Supplementing broiler diets with Cu at prophylactic concentrations (> 125 ppm) can enhance broiler live performance [51, 52]. With regard to aP content of the diets, most of the previous research findings on the effect of Cu supplementation on broiler performance were done with diets that had a normal aP content. However, the effects of Cu supplementation on a low-aP diet were examined in only a limited number of studies. In contrast to our results, Banks et al. [20] reported no significant effect on live performance in broiler chicks fed a lowaP diet supplemented with Cu. However, in their study, the duration of the experiment was too short (9 to 21 d) to make direct comparisons with the current study. Pekel et al. [14] found that adding 250 ppm of Cu from Cu sulfate to a corn-soybean meal diet increased the feed consumption of broilers during a 21-d study, which is different from the results of the current study. Birds fed 250 ppm of supplemental Cu had significantly lower total serum cholesterol than birds fed diets without supplemental Cu, with a difference of 22.44 mg/dL of total cholesterol. This result is consistent with that of Bakalli et al. [53], who reported that adding 250 ppm of Cu from Cu sulfate to a broiler diet significantly decreased plasma cholesterol. The mechanism leading to a decrease in total blood cholesterol induced by high dietary Cu in broilers has not been clearly elucidated. However, no main effect or interaction effects of dietary Cu addition were observed for serum HDL cholesterol or triglycerides. Similar findings have been reported by Konjufca et al. [54] in broilers fed diets supplemented with 180 ppm of Cu from Cu sulfate. In contrast, Bakalli et al. [53] found a significant increase in plasma HDL cholesterol with Cu supplementation. Konjufca et al. [54] hypothesized that supplemental Cu would decrease blood glutathione levels, and this could cause a reduction in tissue cholesterol concentrations. This report is in agreement with results of the current study, in which we report that broilers fed a Cu-supplemented diet had lower thigh meat cholesterol in parallel with decreased blood cholesterol. Because Cu was confirmed to decrease blood cholesterol in the current study, the result regarding thigh meat cholesterol concentrations is not surprising.
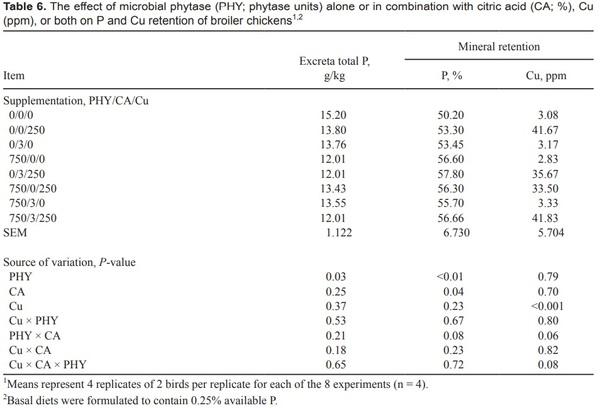
Dietary PHY supplementation did not affect broiler live performance during the 13- to 42-d period. These results on growth performance from 13 to 42 d of age are consistent with the results of Angel et al. [55], who reported no difference in growth performance in broilers from 18 to 32 d when the aP concentration of the diet was reduced from 0.32 to 0.25% with PHY supplementation. The lack of efficacy of PHY on live performance throughout the 13 to 42 d could be due to the 0.45% level of aP fed during the chick starter period. Because the need for dietary aP is highest during the starter phase, when broilers are forming their bone structure, it may be that the 0.45% aP content of the chick starter diet provided positive benefits to the bird throughout the entire study [56]. In this study, mortality was not significantly affected by dietary PHY during the entire study (13 to 42 d) after the birds had been fed a sufficient-aP (0.45%) diet from 0 to 13 d (2.59 and 2.44% for the 0 and 750 phytase units/kg treatments). Similarly, Waldroup et al. [6] suggested a minimum of 0.25% dietary aP to prevent high mortality rates in broilers fed a corn-soybean meal diet. Although it has been demonstrated in many studies that PHY addition positively affects production performance in poultry [4, 57, 58], only a limited number of studies have investigated its effects on carcass characteristics. Hot carcass and tibia weights were increased with PHY supplementation. Pillai et al. [59] also reported improvements in carcass yield in 6-wk-old broiler chickens fed a low-aP diet supplemented with 1,000 phytase units/kg of Escherichia coli PHY. Published data about the effect of dietary PHY on concentrations of Zn in tibia ash are controversial. Similar to results of the current study, Qian et al. [60] and Sebastian et al. [4] reported no significant effects on any of the minerals measured in total tibia ash in broiler chicks fed a low-aP diet supplemented with PHY. In contrast, Viveros et al. [58] observed increased Zn concentrations (by 8%) in tibias of 6-wk-old broiler chicks with PHY supplementation. Copper supplementation increased tibia ash content when the diets were supplemented with PHY, but tibia P content was intermediate for birds fed diets supplemented with PHY and Cu, respectively. Microbial PHY supplementation influenced thigh weight, with birds fed PHY-supplemented diets having heavier thigh weights than birds fed diets not supplemented with PHY. This was mostly due to the greater hot carcass weights of birds fed PHY-supplemented diets. As expected, supplementing a low-aP diet with PHY increased P retention and decreased P output, which agrees with the results of previous studies in broiler chickens [4, 58].
CONCLUSIONS AND APPLICATIONS
1. Application of 0.25% dietary aP after birds were fed an adequate-P diet for 0 to 13 d in conjunction with PHY supplementation should allow for markedly reduced excretion of P in the litter, with an improvement in P retention.
2. Interactions between Cu and CA suggest that these additives have some beneficial influence on the live performance of broilers from 13 to 42 d.
3. Dietary prophylactic Cu from Cu proteinate in the diet may be feasible to reduce cholesterol concentrations in broiler meat.
4. In conclusion, under the conditions of the current study, the potential exists for diminishing environmental pollution resulting from P excretion by using PHY and CA in broiler diets without compromising production performance.
This article was originally published in 2012 Journal of Applied Poultry Research 21:335–347. http://dx.doi.org/ 10.3382/japr.2011-00416. This is an Open Access article under a Creative Commons License.