I. INTRODUCTION
Since the 20th century when commercial poultry breeding first started, the growth rate of meat chickens has quadrupled, and their body structure has also changed dramatically especially with the enlarged size of the breast muscles (Muir and Aggrey, 2003). This is the result of a combination of selective breeding, efficient production systems, improved diets and veterinary care (Fanatico et al., 2007). It now takes about 38 days for day old fast-growing broilers to reach market live weights (~ 2.0-2.5kg) in comparison to the year 1976 when it took 63 days (Quentin et al., 2003). However, the fast growth rate of broilers has been associated with metabolic and skeletal disorders such as tibial dyschondroplasia, twisted legs, sudden death syndrome and ascites. (Blagojevic et al., 2009, Baghbanzadeh et al., 2008; Shim et al., 2012). In contrast to some countries, the only meat chickens available commercially in Australia are the fast-growing strains. A slower-growing strain which, although it may only appeal to a specific market and attract a premium price owing to its higher production costs, environmental footprint and reduced carcass yield (Elanco Animal Health, 2016), will have the advantage of improved leg health and lower mortality rates (Fanatico et al., 2008; Sirri et al., 2011; Cömert et al., 2016). Moreover, there is a need to develop an SGB strain in an Australian context that would provide an alternative choice for chicken meat consumers. This study provides a better understanding of the productivity, feed efficiency, and meat quality of a candidate SGB strain.
II. METHODS
Eggs were obtained from a Cobb X New Hampshire cross and incubated. Hatched chicks (116) and 120 one-day-old Cobbs obtained from a commercial hatchery were vaccinated for Marek’s disease, infectious bronchitis and Newcastle disease. The chicks were allocated into four mixed-sex pens for each breed, consisting of either 30 Cobbs or 29 SGBs, and were grown to a final weight of 2- 2.2kg live weight. Both breeds were reared on the same diet (starter: ME 12.10 MJ/kg, CP 20.2%, grower: ME 12.70MJ/kg, CP 18.7% finisher: ME 13.05MJ/kg, CP 16.8%) based on the nutrient specifications for Cobb 500. Mortality was recorded daily, and post-mortem analysis was conducted accordingly. The birds and feed were weighed on a weekly basis. At 21 days of age, 2mL of blood was drawn from 2 SGBs and 2 Cobbs from each pen which were wing tagged, and subsequently injected subcutaneously with 0.25mL of a 2% suspension of sheep red blood cells (SRBC) for an antibody response test (Haghighi et al. 2005). According to the procedures of Groves and Muir (2014), latency-to-lie (LTL) test was performed on 30 male birds for each breed when they reached the final weight, as an objective measure used to assess broiler leg strength. At the respective final weights for each breed, 6 male birds were randomly selected from each pen and housed in four metabolism pens for 48hrs collection of excreta to calculate the AME of the diet (Ravindran et al. 2000). A total of 40 broilers (20 female and 20 male) of each breed were randomly selected at their final weights for carcass analysis. A Konica Minolta chroma meter 400 was used to determined L* (lightness), a* (redness) and b* (yellowness) values for breast meat. The ultimate pH was measured by placing the electrode of a portable pH meter on the outer side of the cranial breast muscle (Quentin et al. 2003). Drip loss is a measure of the water-holding capacity (WHC) of the meat product. The breast muscle used for drip loss evaluation was stored on a suspended net and removed, wiped and weighed on days 1, 3, 5 and 10 post-slaughter. Data were analysed with a one-way analysis of variance (ANOVA) using Genstat 18th edition, with breed as the main effect. A t-test was used to compare the means and considered to be significantly different if P < 0.05.
III. RESULTS
On a weekly basis, body weight gain between the two breeds was significantly different (P < 0.001) with the final weight of 2-2.2 kg achieved by Cobbs on day 32 and SGBs on day 55. The cumulative feed consumption on a weekly basis was also significantly different between the two breeds, with Cobbs consuming 76% more feed as compared to SGBs on day 32 (P < 0.001) although, SGBs overall consumed 24% more feed than Cobbs to reach the final weight. SGBs, therefore, had a higher FCR at processing of 1.977 which was 0.536 points higher than the Cobbs (1.441). Cobbs had a significantly higher total anti-SRBC antibody titre of 13 in comparison to the SGB titre of 6 (P = 0.05), and an IgM titre of 11, compared to 4 for the SGBs. The IgG titre of 2 for both breeds meant that neither produced anti-SRBC IgG. During the LTL test, Cobbs had a significantly shorter mean standing time (177.3 secs) as compared to SGBs (242.7 secs; P < 0.01). The AME:GE of diets for Cobbs (0.79) was 2.60 % higher than the SGBs (0.77; P < 0.05).
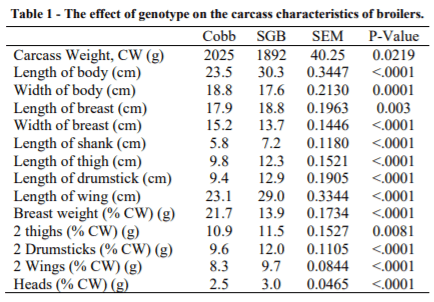
The carcass characteristics of Cobbs and SGBs are presented in Table 1. SGBs had significantly longer bodies and breast, whereas Cobbs had wider bodies and breast (P < 0.01). Significantly higher shank, thigh, drumstick and wing length were observed in SGBs as compared to the Cobbs (P < 0.001). Relative to the carcass weight, breast weight of Cobbs was significantly higher than SGBs, while the thighs, drumsticks, wings, and head were significantly heavier in SGBs (P < 0.01). The crop, proventriculus, jejunum and ileum as well as the liver and abdominal fat pad were significantly heavier in Cobbs (P < 0.05) as compared to the SGBs, which had significantly heavier gizzards, duodenum and caeca (P < 0.001).
Cobb breast meat was paler as compared to the SGBs (L* = 51.93 and 45.39 respectively) (P < 0.001). In contrast, the breast meat of SGBs was significantly redder (a* = 2.64) and less yellow (b*= 3.84) than the Cobbs (a* = 2.08; b* = 4.97) (P < 0.05). The ultimate pH of the breast for SGBs (5.74) was significantly lower than the Cobbs (5.88) (P < 0.001), while the pectoralis major of SGBs had higher drip loss than the Cobbs (P < 0.05).
IV. DISCUSSION
Weight gain, feed intake and FCR were found to be significantly different between Cobbs and SGBs as supported by previous research (Fanatico et al., 2008). Cobbs have been genetically bred for increased body weight and breast muscle yield, whereas the SGBs in the current study had a layer strain as one of its parents, thus diluting this genetic effect. The difference in feed intake between the two breeds was related to the duration of rearing (Fanatico et al., 2005). In the current study, the Cobbs had a lower cumulative feed intake (3.04 kg per bird) as compared to the SGBs (4.03kg per bird) (P<0.05). However, the daily feed intake at day 32 of Cobbs (0.790 kg/bird) was higher than the SGBs (0.726kg/bird). The fast growth rate of Cobbs leads to the rapid conversion of feed to meat. Further, SGBs have a slower growth but higher maintenance requirements due to their higher mobility, as seen in the longer LTL times reported in this study, thus affecting the feed efficiency (Fanatico et al., 2008).
The fast-growing Cobb strains had a stronger immune response (IgM) to the SRBC antigen as compared to SGBs. This is in agreement with earlier studies in turkeys (Li et al., 2000; Cheema et al., 2007) where selection for fast-growing strains altered the T cell subpopulations and the response of the humoral immunity to the SRBC antigen while also showing a decline in IgG antibody production. Genetic difference also affects the broilers’ immune response, with higher IgM titers to SRBC produced by meat chickens than layers (Koenen et al., 2002).
SGBs have stronger legs than the Cobbs as fast growth rates negatively impact the leg strength (Tuyttens et al., 2008) and results in greater pressure being exerted on their immature bones (Webster, 1995). According to de Verdal et al. (2010), chickens which possessed larger gastric compartments had more effective nutrient utilization. In the current study, Cobbs had higher proventriculus weight in comparison to the SGBs, possibly resulting in higher enzyme production and gastric secretions, subsequently improving AME utilization. Intensive selection for increased breast muscle yield in fast-growing Cobb leads to the reduction in the relative yield of other parts (Fanatico et al., 2008; Fanatico et al., 2005). The higher wing and leg-quarter yield identified with SGBs can be explained by their increased activity and greater utilisation of their wings, promoting bone mass and supporting muscle mass (Abdullah and Buchtova, 2016). The heavier fat pad in Cobbs could be a consequence of faster growth as proposed by Havenstein et al. (1994) where the selection for heavier broilers concomitantly promoted accumulation of fat.
The varying degree of redness of the breast meat seen between strains can be associated with the difference in slaughter ages with increase in the myoglobin content reported with age (Berri et al. 2001; Gordon and Charles 2002). The selection for increased growth rates and breast meat yield of Cobb broilers leads to diminished post-mortem glycolysis and higher ultimate pH (Berri et al., 2001). This may be due to the lowered glycogen content of Cobb breast muscle, thus, explaining the differences in breast muscle pH between the breeds. Berri et al. (2001) suggested a strong negative correlation between breast muscle pH 24 hours post-mortem and drip loss, thus, accounting for the higher drip loss in SGBs. A poor WHC leads to lack of juiciness in whole meat and further-processed products.
V. CONCLUSION
The main advantage of SGBs is the provision of an alternate chicken meat that is also welfare-oriented, as indicated by their longer standing time during the LTL, and would likely attract a ‘niche’ market at a premium price. However, with regards to their performance, carcass characteristics and meat quality, further research and refinement would be necessary in terms of breeding objectives and nutrient requirements to improve this strain.
ACKNOWLEDGEMENTS: Research and technical support at the University of Sydney and the Birling Poultry facility is acknowledged.
Abstract presented at the 30th Annual Australian Poultry Science Symposium 2019. For information on the latest edition and future events, check out https://www.apss2021.com.au/.