Introduction
Brazilian agriculture is concentrated in the tropical and subtropical zone, where soils are highly weathered and chemically infertile, demanding amelioration by liming and high amounts of plant nutrient inputs (Cantarella et al., 1998). In Brazil, soybean is the main crop both in scale and in value, covering ~38 M ha with an average yield of 3.5 t ha−1 (Cattelan and Dall'Agnol, 2017; EMBRAPA, 2022). Annually, about 1.2 M t of P are required to produce 135.4 M t of soybean grain (Fageria et al., 2017). However, Brazil does not contain enough high-quality phosphate reserves to cover its agricultural demand, and this places the country as a large fertilizer importer (ANDA-Associação Nacional para a Difusão de Adubos, 2020). On the other hand, high amounts of plant nutrients are available in the form of organic residues. Poultry production is presently expanding, and currently, Brazil is the first world exporter (USDA, 2021). Poultry litter, as well as other animal manures, is a valuable organic fertilizer due to the presence of essential plant nutrients, including P (Rogeri et al., 2016). However, if these residues are not well managed, they can result in environmental contamination (Sharpley et al., 2007). In Brazil, 6.8 million m3 year−1 of poultry litter is produced and these residues contain ~240, 270, and 200 thousand tons of N, P, and K, respectively, which represents almost 15% of the Brazilian fertilizer demand (Corrêa and Miele, 2011). A standard chicken house with 20,000 birds and six production cycles of 60 days would generate 220 Mg of litter in each cycle with a P content of 9.3 g kg−1 P (Withers et al., 2018).
To reduce environmental impact and to improve the nutrient use efficiency of organic fertilizers, technological development for adequate use of organic residues in agriculture is highly demanded in Brazil. It is well known that continuous use of poultry litter increases plant nutrient levels in the soil (Kingery et al., 1994; Sharpley et al., 2007), being as efficient as a mineral fertilizer, particularly in P-depleted tropical soils (Frazão et al., 2019). However, due to the low nutrient concentration or the nutrient imbalance in the poultry manure, long-distance transportation is limited, resulting in long-term litter disposals in areas close to the poultry production units. Excessive application of poultry manure in the same area year after year and at high rates exceeding crop needs causes soil saturation and can lead to eutrophication of surface waters by phosphorus runoff (Sharpley and Moyer, 2000; Szogi and Vanotti, 2009). Mineral enrichment of residues may stimulate long-distance transportation of manure. Additional support has recently appeared: according to the Brazilian fertilizer legislation, the mixture of organic residues and mineral fertilizer results in a new category of farm input called organomineral fertilizers (MAPA, 2017). Furthermore, recent studies have demonstrated the positive effects of both conventional and organomineral fertilizers on crop yields (Corrêa et al., 2016; Frazão et al., 2019; Mumbach et al., 2019). However, little is known about the long-term efficacy of organomineral fertilizers on annual crop growth in an acidic Ferralsol, which covers vast areas in Central Brazil. Further, it is recognized that large areas under agriculture in Brazil have received more fertilizer P than required for crop needs since 1970 and have therefore accumulated significant legacy soil P reserves (Withers et al., 2018). Hence, studies on P fertilizer efficiency should take residual P into account.
The objective of this article is to evaluate the agronomic efficiency of organomineral fertilizers in supplying P to soybean and maintaining the soil P level of a Ferralsol as a strategy for poultry litter P recycling in a 6-year field experiment in the Brazilian Cerrado region. We further investigated the effect of legacy P or residual P on soybean yield after several years of soil P fertilization.
Materials and Methods
Fertilizers
Granulated phosphate organomineral fertilizers were produced at the Fertilizer Laboratory of the University of Rio Verde, Goias State, Brazil. Raw organomineral fertilizer (ROMF) was produced using raw poultry litter collected in a commercial grange after six batches of broiler rearing (about 18 months). Composted organomineral fertilizer (COMF) was made using an organic compost obtained by a mixture of poultry litter and sugarcane bagasse (1:5 v/v) composted in aerated piles for 120 days (Table 1). After the drying process (45°C for 48 h), both organic residues were crushed and sieved to a size smaller than 1 mm. The final composition of the organomineral fertilizer was achieved by mixing the three parts of organic composts with one part of monoammonium phosphate (MAP), and 2% of calcium bentonite (Clariant) used as ligand, to facilitate the granulation process and increase the resistance of the granules. The organomineral fertilizers were granulated in a small disc granulator with rotation control (45 rpm, 65 degrees angle). A solution of 50 g L−1 neutral sodium silicate (Na2O (SiO2)4) was sprayed for granulation (~250 ml kg−1). Samples were oven-dried, and granules were sieved to sizes between 1 and 4 mm. In general, 100 kg of the mixture contained 5 kg N, 20 kg P2O5, and 2 kg K2O, as slight changes occurred in the mixture composition due to manual preparation for each crop season (Table 2). However, the mixture composition and characteristics were always in agreement with the Brazilian Fertilizer Regulation (MAPA, 2009). Regarding P composition in the organomineral fertilizers, about 10% of P originated from organic residue, while 90% came from monoammonium phosphate. Granules (size from 1 to 4 mm) of commercial MAP (Mosaic Fertilizer) were used as a reference soluble mineral P source.
TABLE 1. Poultry litter and poultry litter compost characterization.
TABLE 2. Fertilizers characterization.
Field Experiment
From 2010 to 2016, a field experiment was carried out at the CTC-COMIGO Research Station (Technological Center of the Farm Cooperative of Southwestern Goias) in Rio Verde, State of Goias, Brazil (Figure 1A). The climate is Aw according to Köppen's classification (Alvares et al., 2013) with a mean annual temperature of 23.4°C and mean annual precipitation of 1,496 mm, falling mostly in summer (Figure 1B). The deep, well-drained, sandy clay loam soil in the experimental site is a Ferralsol (IUSS-WRB. World Reference Base for Soil Resources, 2015) or Latossolo Vermelho (SiBCS-Sistema Brasileiro de Classificação de Solos, 2018). The soil properties of the study site are shown in Table 3. For 6 years before setting up the experiment, cropping during the summer season involved soybeans followed by winter fallow, which is the standard rainfed cropping practice in this region.
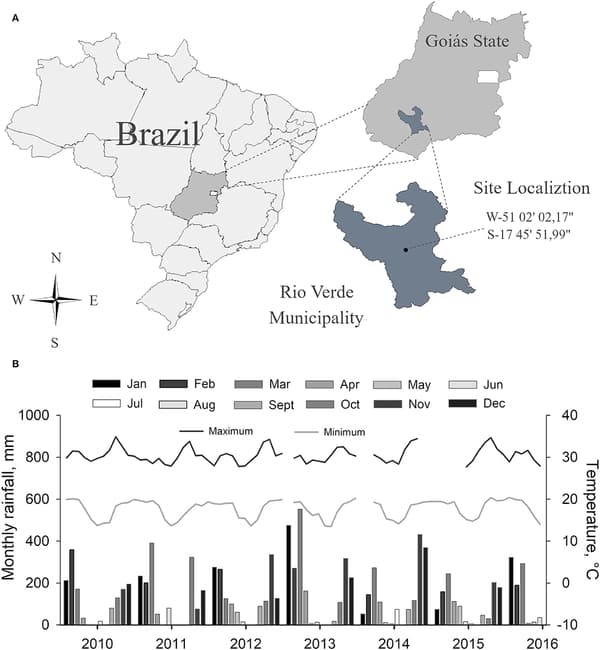
FIGURE 1. Experiment site location (A) and monthly rainfall and temperature for each crop season (B). The gaps in temperature values mean unavailable data. Rainfall was recorded on the experimental site and temperature was obtained from INMET.
TABLE 3. Soil chemical and textural characterization.
Three fertilizers (ROMG, COMF, and MAP) at four rates were used as treatments. The fertilizer doses applied were 20, 40, 60, and 80 kg ha−1 of P2O5 (based on fertilizer total P), including a control plot with no P application. The fertilizer was band-applied at sowing time in each crop season. Treatments were established in a randomized block factorial design with four replications. Each plot was 25 m2 with 10 rows of plants spaced by 50 cm. To evaluate the residual P effect and if there is evidence of P supply to crop plants after several years of P fertilization (Sattari et al., 2012), in the last crop season (2015/2016), no P was added to the soil and soybean yield was measured for all treatments to verify the effect of residual P, which is the difference between P inputs (in this case, mostly from mineral and organomineral fertilizers) and P outputs (predominantly by the withdrawal of P in harvested soybean).
Six soybean crop seasons were evaluated every summer from 2010/2011 to 2015/2016. Soybean sowing was done using a no-till planter every year at the beginning of the rainy season, with little difference in date between years, ranging from 20 October to 10 November. The soybean cultivar was Anta 82 RR in the first two crop seasons and MS 7739RR in the following seasons. After harvesting the soybean, the field was left fallow during the winter dry season. The soil was limed 1 year before setting up the experiment, and potassium was broadcast as potassium chloride (KCl) every crop season at a dose of 80 kg ha−1 of K2O. Soybean seeds were treated with N-fixing inoculants and pesticides were sprayed according to the regular agronomic protocol of COMIGO in all crop seasons.
Plant and Soil Analysis
Leaf and soil P concentrations were evaluated. Leaf samples, 10 trifoliate leaves per plot, were collected at the R1 growth stage in the seasons of 2010/2011, 2011/2012, 2012/2013, and 2013/2014. The samples were oven-dried at 65°C until constant mass, and the P concentration in the leaf tissue was determined after nitric-perchloric acid digestion by inductively coupled plasma optical emission spectrometry (Teixeira et al., 2017).
Soil samples were collected in the 0–20 cm layer after soybean harvest in the seasons of 2010/2011, 2012/2013, and 2013/2014. Mehlich-1 extractant was used to extract available P (Teixeira et al., 2017), and P concentration in extracts was determined by ICP-OES.
Soybean grains were harvested in a 10 m2 area in the center of each plot. Annual soybean yield was determined after harvest, and grain moisture was adjusted to 13%, expressing yield in kilogram per hectare (kg ha−1).
Efficiency and Statistical Analysis
The relative agronomic efficiency (RAE) was calculated for both organomineral sources based on the cumulative grain yield after 5 seasons. The following formula was used to calculate RAE:
Where Soybean Yield OMx is the sum of 5-crop soybean yields under each organomineral treatment at a dose of x kg ha−1 P2O5; Soybean Yield MAPx is the sum of 5-crop soybean yields under MAP treatment at a dose of x kg ha−1 P2O5, and Soybean Yield Control is the sum of 5-crop soybean yields in the control. The relative efficiency was calculated only when the soybean yield averages were statistically significant, which occurred only at rates of 20 and 60 kg ha−1 of P2O5.
The data were submitted for analysis of variance (ANOVA) (p ≤ 0.05) and where significant, the average values were compared using Tukey's test (p ≤ 0.05) by SAS software (SAS Institute, 2001). Regression models were best fitted using the least square fitting method with SigmaPlot version 9, from Systat Software, Inc., San Jose, California, USA, www.systatsoftware.com. Additionally, the box-plot representation method (Sokal and Rohlf, 1995) was applied to get a comparative picture of the soil P availability throughout the crop seasons and at different fertilizer doses.
Results
Apart from the 2010/2011 crop season, fertilizer addition did result in soybean yield increase with the increasing addition of fertilizers, particularly in 2012/2013, showing a 45.2% increase with 4,500 kg ha−1 at 80 kg ha−1 P2O5 (Figure 2). However, there was no significant response to fertilizer addition in the first crop season, with an average soybean yield of 3,609 kg ha−1 (Figure 2A). In the 2011/2012 and 2012/2013 crop seasons, a slight difference in soybean yield was observed between fertilized and non-fertilized treatments, but there was no significant difference between fertilizer sources or amounts applied. In these two crop seasons, the quadratic squared root curve equation enabled the estimation of soybean yield by the P dose applied (Figures 2B,C). A linear response to increasing fertilizer rate addition was observed only in the 2013/2014 and 2014/2015 crop seasons (Figures 2D,E). However, no statistical difference in soybean yield was observed between organomineral and MAP fertilizer applied at the same P rate to the soil. The average yields were 3,462, 4,505, 2,619, and 2,387 kg ha−1 for the crop seasons of 2011/2012, 2012/2013, 2013/2014, and 2014/2015, respectively.
FIGURE 2. Soybean grain yield from treatments with different fertilizers sources and P doses in the crop seasons 2010/2011 (A), 2011/2012 (B), 2012/2013 (C), 2013/2014 (D), and 2014/2015 (E). COMF, composted organomineral fertilizer; MAP, monoammonium phosphate; ROMF, raw poultry litter organomineral fertilizer.
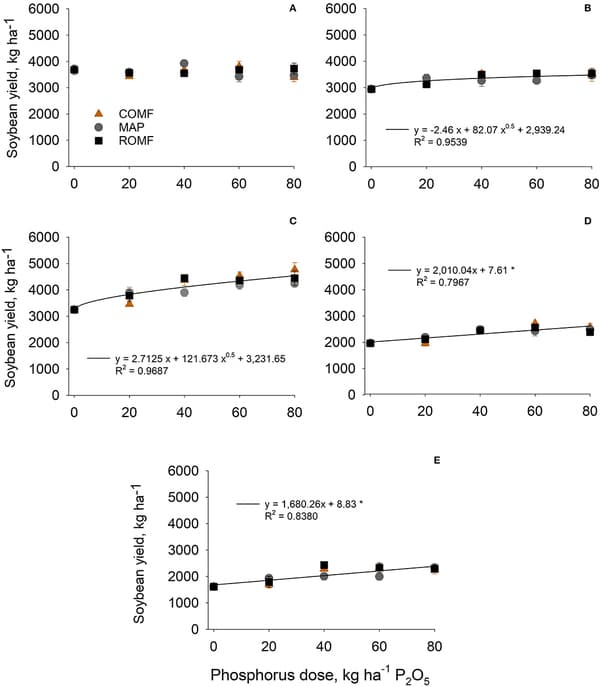
Considering the accumulated soybean yield over the five crop seasons, a significant response of P fertilization over soybean yield (Figure 3) was observed. However, a significant difference between organomineral and mineral sources was observed only for rates of 20 and 60 kg ha−1 P2O5. At the rate of 20 kg ha−1 P2O5, MAP was more efficient than the organomineral fertilizer produced from composted poultry litter (RAE = 20.3%). However, at the rate of the 60 kg ha−1 P2O5, both organomineral fertilizers were superior to MAP (RAE COMF = 174.2% and RAE ROMF = 167.6%). The organomineral fertilization at a 60 kg ha−1 P2O5 dose resulted in an average production over 5 seasons of 55.0 and 55.6 kg of soybean for each kg of P2O5 applied, for ROMF and COMF, respectively. This value means an average productivity gain of 7.8 and 9.0% about fertilization with MAP (51.0 kg of soybean for each kg of P2O5 at 60 kg ha−1 P2O5 dose).
FIGURE 3. Accumulated soybean grain yield from treatments with different fertilizers sources and P doses after five crop seasons (2010–2015). COMF, composted organomineral fertilizer; MAP, monoammonium phosphate; ROMF, raw poultry litter organomineral fertilizer.
The P concentration in soybean leaves increased linearly with increasing amounts of P applied as fertilizers in all crop seasons, except for the 2011/2012 season (Figure 4). The average P concentration in soybean leaves was 1.88 g kg−1 of P for the crop season of 2011/2012 (Figure 4B). For the 2012/2013 and 2013/2014 crop seasons, P concentration in soybean leaves ranged from 2.49 g kg−1 of P (control treatment) to 2.99 g kg−1 of P (highest P rate applied) and from 1.56 g kg−1 of P (control treatment) to 2.04 g kg−1 of P (highest P rate applied), respectively (Figures 4C,D).
FIGURE 4. Phosphorus content in soybean leaves with doses of fertilizer sources in the crop seasons of 2010/2011 (A), 2011/2012 (B), 2012/2013 (C), and 2013/2014 (D). COMF, composted organomineral fertilizer; MAP, monoammonium phosphate; ROMF, raw poultry litter organomineral fertilizer.
After 3 crop seasons, increasing rates of fertilizers added to the soil resulted in a 9.58% linear increase in soil P availability at 0–20 cm depth from 8.35 mg kg−1 before starting the experiment to 9.15 mg kg−1, the highest soil P concentration found at the rate of 80 kg ha−1 P2O5 (Figure 5B). On the other hand, the source of P did not influence the soil P availability. During the 2013/2014 season, after four crop seasons, a quadratic squared root response was observed for soil available P (Figure 5C), showing that changes in available soil P concentration due to fertilizer application may take longer than 6 years.
FIGURE 5. Available soil P (Mehlich-1) after treatment with different organomineral and MAPfertilizers and doses in the crop seasons of 2010/2011 (A), 2012/2013 (B), and 2013/2014 (C). COMF, composted organomineral fertilizer; MAP, monoammonium phosphate; ROMF, raw poultry litter organomineral fertilizer.
A significant decrease in available P was observed after three crop seasons in the control treatment (Figure 6A). Fertilizer doses of 20 and 40 kg ha−1 P2O5 did not have a significant effect on soil available P between seasons when the soil was sampled (Figures 6B,C). An increase in P availability after three crop seasons was observed only for the highest P doses (Figures 6D,E).
FIGURE 6. Box and bar plots of available soil P in 3 crop seasons and at different organomineral and MAP fertilizer doses: control (A), 20 (B), 40 (C), 60 (D), and 80 (E) kg ha−1 P2O5. The middle horizontal line is the median value, the extent of the upper box is the first quartile, the extent of the lower box is the third quartile, and 50% of the data lie within the 2 boxes (the interquartile range, IQR). The extent of the bars is the last data point or 1.5 times the distance of the IQR if there are outliers. Outliers are marked with a dot sign in the plot. Means followed by different letters are statistically different p ≥ 0.05 (Tukey's test).
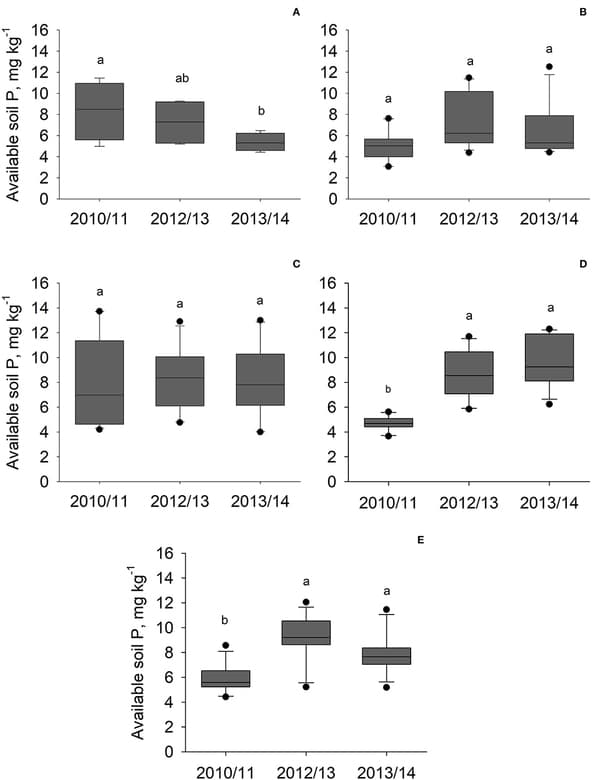
In the last harvest season (2015/2016), it appears that residual P had an effect on soybean yield, which followed an exponential model and the highest observed yields were above 3,000 kg ha−1 although no P fertilizer was applied in the 2015/2016 harvest season (Figure 7). The soybean yield curves were also similar for all P sources as there was no difference between them concerning the residual P.
FIGURE 7. Soybean yield in the crop season of 2015/2016 using the residual P after 5 crop seasons with organomineral and mineral fertilization. COMF, composted organomineral fertilizer; MAP, monoammonium phosphate; ROMF, row poultry litter organomineral fertilizer.
Discussion
In general, the difference in average soybean yield among the five evaluated crop seasons can be explained by the weather, mainly by the rainfall (Figure 1), and by the residual soil fertility. Concerning the weather, the lowest soybean yield was observed in the crop seasons of 2013/2014 and 2014/2015 (on average of 2,619, and 2,387 kg ha−1, respectively) due to the low monthly rainfall in January in both seasons. In these two seasons, the soybean yields were slightly below the average yield in southwestern Goias, equivalent to 2,900 and 2,594 kg ha−1, respectively (CONAB-Nacional Company of Food Supply, 2019). Drought stress at this stage of plant development (R5-beginning seed/R6-full seed) can strongly impair soybean growth as the crop plant reaches its high photosynthetic rate, making it a critical period for yield formation (Board and Kahlon, 2011). For the crop seasons of 2010/2011, 2011/2012, and 2012/2013, when the weather was favorable for crop growth, average soybean yields were 3,609, 3,462, and 4,505 kg ha−1, respectively (Figure 2), being higher than the regional yield averages for the same period (3,140, 3,120, and 2,965 kg ha−1, respectively) (CONAB-Nacional Company of Food Supply, 2019).
This study showed that P applications in the first crop season (2010/2011) did not result in a soybean yield increase, as several years of soil cultivation and P fertilization before setting up the field study have provided an adequate level of plant-available P (8.35 mg kg−1; Table 1). In the 2010/2011 crop season, there was no difference between control and even the higher 80 kg ha−1 of P2O5 dose (Figure 2A). As reported by Withers et al. (2018), it is, however, not easy to determine that residual P can be used for profitable crop production, which can be sufficiently mobilized to provide all or part of plant P demand. On the other hand, we propose that the presence of soil residual P should be taken into account in studies about agronomic efficiency between alternative P fertilizers, including organomineral, and conventional fossil-reserve based P fertilizers, such as MAP or super triple phosphate.
In the second crop season, a slight difference between the control and the fertilized treatments was observed. However, there is no significant difference among P doses (Figure 2B). A significant positive response to P fertilization was evident only in the third crop season when a squared root quadratic curve was observed (Figure 2C). This quadratic form on the squared root scale is known to describe the functional relationship between crop yield and the rate of P fertilizer (Colwell and Goedert, 1988). In the current study, we observed that residual P could provide soybean demand for up to two crop seasons resulting in good yields. In the last two crop seasons (2013/2014 and 2014/2015), due to weather conditions, crop response to P inputs was not clear. Considering the sum of all evaluated crop seasons, a squared root quadratic curve response of soybean to P fertilization (Figure 3) was observed, suggesting that the optimal dose is between 40 and 60 kg ha−1 P2O5.
There was no significant difference among P sources regarding soybean yield and soil P availability in any of the individually evaluated crop seasons. However, accumulated soybean yield data from all harvest seasons showed a significant difference between mineral and organomineral fertilizer at the 20 and 60 kg ha−1 P2O5 doses. The agronomic efficiency of organomineral fertilizer relative to the monoammonium phosphate (MAP) varies according to the P dose. Once the soybean yield response curve to P fertilization fits a square root quadratic curve, doses of P inputs smaller than 40 kg ha−1 P2O5 are in the responsive range for P application and doses of 60 kg ha−1 P2O5 and above are out of the responsive range for P. Monoammonium phosphate was more efficient than organomineral at the dose of 20 kg ha−1 P2O5, indicating that it is more efficient in P supply to soybean. In contrast, at 60 kg ha−1 P2O5 dose, both organomineral fertilizers are more efficient than MAP, suggesting that there are factors other than P that are responsible for a higher soybean yield, such as micronutrient availability or the presence of organic compounds (Hinsinger et al., 2011).
Evidence suggests that sustained crop yields and soil productivity can better be accomplished with organomineral fertilizers than with mineral inputs (Sá et al., 2017; Grohskopf et al., 2019; Crusciol et al., 2020), although much remains to be learned about the processes involved. Other studies have shown similar crop yields (Corrêa et al., 2016, 2018; Frazão et al., 2019; Mumbach et al., 2019). The nature of the organic structure and chemical composition among different organomineral fertilizers is highly variable and results cannot be generalized. It is not clear whether soil and climate conditions are favorable or not for the higher performance of organomineral fertilizers.
Similarly to crop yield, the nutrient concentration in plant tissue does not differ between sources with similar P solubility (Corrêa et al., 2018; Grohskopf et al., 2019; Mumbach et al., 2019). Thus, the P concentration in soybean leaves was similar for the sources used, even in different harvest seasons (Figure 4), and in all situations, the P concentration was sufficient for soybean development (Kurihara et al., 2008). It shows that organomineral fertilizer can successfully replace the mineral fertilizer at the same dose of P for crop nutrition. It is important to consider that the majority of P present in organomineral fertilizer came from MAP (> 90%) and a large portion of P in poultry litter is in inorganic form (Mackay et al., 2017). In addition, no mineralization process is required for P release. Although the contribution of poultry litter for the total P added to the soil is small, it represents about a 10% reduction in primary fossil-based phosphate demand. The use of P resources, such as poultry litter has been suggested as an important practice to deal with the shortage of natural P sources (Withers et al., 2018), and organomineral fertilizers can be an efficient way to properly recycle P from organic residues. Care is needed concerning the potential risk for soil biological contamination related to poultry litter use. Organomineral fertilizer is an efficient way to minimize biological risk once thermal and drying processes are used during fertilizer production, which is effective at destroying human and plant pathogens (Chen and Jiang, 2014).
The same levels of soil available P among treatments with different fertilizer sources (Figure 5) can also explain why the organomineral fertilizer did not increase soybean yield and leaf P content. Even when soil P availability was evaluated after three crop seasons with sequential P applications (Figure 5C), no difference was observed between mineral and organomineral fertilizers. According to some authors, the high content of organic compounds in organomineral fertilizers will reduce P adsorption in the soil solid phase. This is based on the concept that organic compounds can bind to P adsorption sites before the orthophosphate, hindering soil P fixation, and making it more available (Weng et al., 2012: Fink et al., 2016). However, soluble and reactive organic substances are only a small part of the poultry litter, which is mainly composed of lignocellulosic compounds (Sharpley and Moyer, 2000). It is also important to consider that soluble P and organic acids show different dissolution dynamics, where P diffusion may occur in a few days while organic acids dissolution depends on biological activity, which can take several weeks. Degryse et al. (2013) showed that, even when active organic acids are mixed with soluble phosphate, the protecting effect occurs only in a very thin layer around the granule, not altering the mobility and availability of P for plant uptake. A high crop yield in soils added with organomineral fertilizer is also attributed to an improvement in soil biological properties (Grohskopf et al., 2019). This finding needs more investigation as some researchers did not find soil biological alteration after organomineral fertilizer application (Morais and Gatiboni, 2015; Frazão et al., 2019).
The soil P availability decreased over time without P fertilizer application (Figure 6A). The intermediate annual dose of 40 kg ha−1 P2O5, was sufficient to keep soil P availability to plant uptake over time (Figure 6C), and the highest doses of P2O5 (60 and 80 kg ha−1 P2O5) improved soil P availability in the last two harvest seasons (Figures 6D,E). Constant P fertilizer application can lead to the accumulation of P in soil (McLaughlin et al., 2011; Rodrigues et al., 2016) and, conversely, low or no soil P application can lead to a reduction in soil P availability (Gatiboni et al., 2007). Although the accumulation of P in tropical soils due to the successive P fertilizations mainly occurs in no-labile forms, a significant increase in available P may also occur (Rodrigues et al., 2016). This study showed that soil P accumulation was observed at high fertilizer doses because the P removal with soybean outputs after five harvest seasons was smaller than the fertilizer P applied. This legacy P (residual P) is recognized as an important P pool to reduce the need for P input in crop farms (Withers et al., 2018). Results have shown that the equilibrium dose of P under the experimental conditions is ~40 kg ha−1 P2O5, which is close to the amount of P exported by soybean harvest (Bortolon et al., 2018). This dose is enough to maintain available soil P and to provide P for soybean yield over time.
Concerning the effect of residual P on soybean yield, the soybean yield in the season 2015/2016 without P application reflects the accumulation of soil P after five crop seasons (Figure 7). In this study, residual P refers to the remaining soil P that was predominantly not taken up by the crop plants after 11 years of soybean cropping (6 years before and after the field experiment), but, as reported by Bowman et al. (2009), contributes to P in soil solution and can be taken up by crops in following harvest seasons. In the 2015/2016 harvest season, soybean yields at all fertilizer doses reflect the effect of residual P. The increase in yield was observed up to 40 kg ha−1 P2O5, followed by a plateau at the highest doses. It seems that the dose of 40 kg ha−1 P2O5 applied over the years was enough to keep the soil P availability above the sufficiency level for soybean growth. The observed yield at 40 kg ha−1 P2O5 was higher than the average Brazilian soybean yield, which means that even in highly weathered tropical soils, such as the Ferralsol in this study, it is possible to manage the residual P for crop nutrition for at least one harvest season. According to Withers et al. (2018), cultivated soils with high amounts of residual P can be observed in large areas in Brazil. This condition is relatively new in the Brazilian Cerrado, where low fertility and high P response were observed in most cases.
Conclusion
The P legacy from previous P fertilization was enough for soybean crop yield in the first crop season, and the applied annual dose higher than 40 kg ha−1 P2O5 was able to maintain the soil P availability and soybean yield in more than one harvest season.
Organomineral fertilizers based on poultry litter, raw or composted, have similar efficiency in P supply for soybean. Thus, composting poultry litter to produce organomineral fertilizers is not needed, and this represents an advantage in industrial processing.
Organomineral fertilizer is more efficient than mineral fertilizer at high doses, suggesting agronomic benefits additional to P supply, such as biological or micronutrients effects, although more investigation is needed to understand the mechanisms involved.
As two-thirds of organomineral consists of poultry litter, this fertilizer can be considered as an effective input to recycle P and other nutrients present in animal manure, which is an important strategy to reuse P and decrease the pressure over limited phosphate reserves.
This article was originally published in Frontiers in Agronomy. 4:785753. doi:10.3389/fagro.2022.785753. This is an Open Access article distributed under the terms of the Creative Commons Attribution License (CCBY).