INTRODUCTION
As we learn more about the negative impacts of calcium (Ca) on the availability of phosphorus (P), it highlights how little we know about Ca requirements, digestibility of Ca in ingredients, and their impact on P digestibility (Angel, 2019). These impacts can be direct or indirect through chelation with phytate, which can have a profound impact on the efficacy of phytase. Historically the ratio of Ca to P has been defined as total Ca to total P or total Ca to a form of available P in the diet (NRC, 1994). References to total Ca and total P still appear in the literature today. Currently we have measures of “availability” of P in ingredients (available, digestible, and retainable P) and most nutritionists formulate diets based on an “availability” value. However, we continue to use total Ca to formulate diets and define Ca requirements. Our inability to define ingredient Ca digestibilities provide accurate estimates of digestible Ca matrix values for phytases and importantly corroborate digestible Ca needs of the animal hinders us in being able to formulate and use both Ca and P correctly. For the animal, what is important is the amounts of Ca and P that can be digested, absorbed, and potentially used for deposition in body tissues as well as for metabolic needs. To fully implement a system that allows us to feed closer to P requirements and reduce P excretions, we need to understand the digestibility of Ca in ingredients and factors that affect these and ultimately, work toward a digestible Ca (dCa) to digestible P (dP) system.
The goal would then be to define a range of optimal digestible Ca to digestible P ratios and levels for diet formulation that require that dCa and dP needs and content in ingredients be known. We also need a better understanding of the impact of other factors, beyond the actual digestibility of the Ca and P in ingredients, that affects the ability of the animal to digest and/or absorb these Ca and P. Some of factors are the physical form of concentrated Ca containing ingredients such as limestone (LM) and their solubility, use and concentration of phytase, inclusion and concentrations of vitamin D or its metabolites, as well as other feed additives, age, and diet ingredient selection (phytate source).
Because we still do not understand the role that each factor plays and the interactions that occur among Ca, P, phytate, and phytase, the current research was undertaken to develop a better understanding of these interactions and to start building toward a dCa and dP formulation system. Therefore, the objectives of current project were to determine the impacts of LM particle size, Ca source (from either LM or bone), phytate source, and presence or absence of phytase on standardized ileal digestibility (SID) of Ca (SID Ca) and P (SID P) in broilers.
MATERIALS AND METHODS
Animals and Housing
All animal care procedures were approved by the University of Maryland Animal Care and Use Committee. On day of hatch, 420 Ross 708 male broiler birds were obtained from a local commercial hatchery and placed in floor pen rooms with artificial light and temperature control. A commercial type prestarter (1–10 d of age, Table 1, formulated at 1.0% Ca and 0.50% nonphytate P) and starter (11–19 d of age, Table 1, formulated at 0.7% Ca and 0.34% nonphytate P) diets with adequate nutrient concentrations were fed. Phytase was not used in the prestarter or starter diet. In the morning of day 20, birds were weighed (average body weight was 724 g, SEM 1.05 g) and groups of 4 birds were placed into battery pens (Modified Petersime grower batteries, Petersime Incubator Co., Gettysburg, OH) preassigned to treatment (TRT) based on a within-block (room) randomization. Birds were weighed individually to ensure similar pen weight and to minimize within pen weight variation. Each wire floored battery pen (width x depth x height; 99 cm x 68 cm x 37 cm) were equipped with 2 nipple drinkers and 2 external feed troughs (length ! width ! depth; 63.5 cm x 8.9 cm x 5.67 cm). Treatment diets were fed from 20 to 22 d of age for 36 h. At the end of 36 h feeding, all birds in the battery cages were anesthetized with a gas mixture of 35% CO2, 35% N2, and 30% O2 (2–3 min) and then euthanized with CO2 (2–3 min). This was performed to minimize smooth muscle contractions in the intestine and minimize displacement of digesta distally. The distal half of the ileum was immediately removed (last half of the segment encompassed between the Meckel’s diverticulum to 3 cm above the ileocecal junction). The content was gently removed by flushing with cold double distilled water. Distal ileal contents were pooled by cage, frozen at −20°C, and freeze dried. Dried ileal samples were ground using a mortar and pestle to pass through a 0.25 mm sieve and stored in airtight containers at 4°C until analyzed.
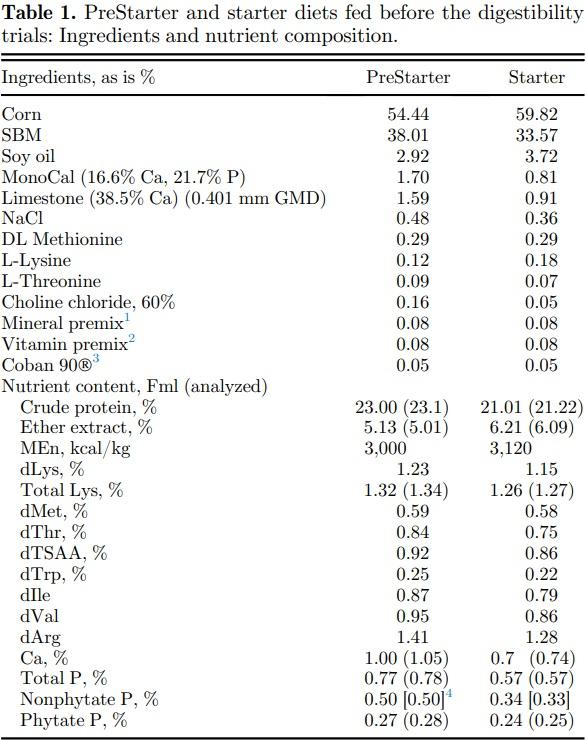
1 Mineral premix supplied per kg of diet: zinc from zinc sulfate, 80 mg; manganese from manganese sulfate, 100 mg; iron from iron sulfate, 20 mg; copper from copper sulfate, 3 mg; iodine from calcium iodate, 3.9 mg; selenium from selenium sulfate, 0.3 mg.
2 Vitamin premix supplied per kg of diet: vitamin A, 15,111 IU; vitamin D, 5,333 IU; vitamin E, 53.33 IU; vitamin B12, 26.66 mg; riboflavin, 17.78 mg; niacin, 71.11 mg; pantothenic acid, 24.89 mg; vitamin K3, 3.2 mg; folic acid, 2.13 mg; biotin, 0.142 mg; thiamine, 4.44 mg; pyridoxine, 6.22 mg.
3 Coban 90, Elanco Animal Health, USA.
4 Calculated as analyzed total P – analyzed phytate P
Photoperiod was 24 light (L):0 dark (D) from hatch to 3 d, 14L:10D from 4 to 7 d, 16L:8D from 8 to 12 d, and 18L:6D from 13 to 22 d of age. Room temperature was kept at an average of 31°C from hatch to 3 d and brooder lamps were used to provide additional heat. Temperature was lowered by 1°C every 2 to 3 d such that bird comfort was maintained and temperature was 24°C at 22 d of age. Birds were checked twice daily and weight of dead bird was recorded. Feed and water were offered for ad libitum consumption throughout the trial.
Experimental Design and Diets
One corn and corn germ–based basal diet (also used as TRT 1) was prepared with no added Ca or inorganic P (Table 2). Three calcium sources i) a commercial LM (PureCal, Cerne Calcium Company, Fort Dodge Fine & Granular Products, Ft Dodge, IA) was obtained and taken to a geometric mean diameter (GMD) of 800 μm and standard deviation (Sdgw) of 347 μm (Figure 1A; TRT 2); ii) a subsample (5 kg) of the commercial LM ground to a smaller particle size and tested (GMD = 151 μm, Sdgw = 128 μm; Figure 1B); TRT 3); or iii) bone from swine meat meal that was separated manually and then ground to the same particle size as the smaller LM (151 μm GMD, Sdgw = 112 μm, Figure 1C); TRT 4) were added to the basal diet to achieve 0.71% Ca (0.7% Ca from LM or bone) to determine the impacts of LM particle size or Ca source on Ca digestibility. In addition, a corn/soybean meal (SBM)– based diet was prepared with 800 μm LM and contained 0.7% Ca from 800 μm LM (total Ca, 0.77%) and no added inorganic P (TRT 5). A batch of each of the above diets (TRT 1–5) were mixed and subdivided into 2 parts. One half constituted the TRT without phytase (1–5) and to the other half, a 6-phytase (Axtra PHY, Buttiauxella spp., expressed in Trichoderma reesei) was added on top, based on analyzed activity, to achieve 1,000 U/kg diet for TRT 6–10. In addition, a N, Ca, and phytate-P–free (NCaPP-Free) diet was mixed and used to correct for endogenous losses of Ca and P (Table 3). The two LM samples, 800 or 150 μm, and monosodium P were added to the NCaPP-Free diet to determine LM (800 μm GMD) impact on LM Ca and inorganic P digestibility (TRT 11 and 12, respectively, Table 3) in a diet without phytate. In all TRT, titanium dioxide (TiO2) was included at 0.58% as the inert marker and Celite (Celite, Celite Corp., CA) was used as a filler to achieve 100% of the final diet.
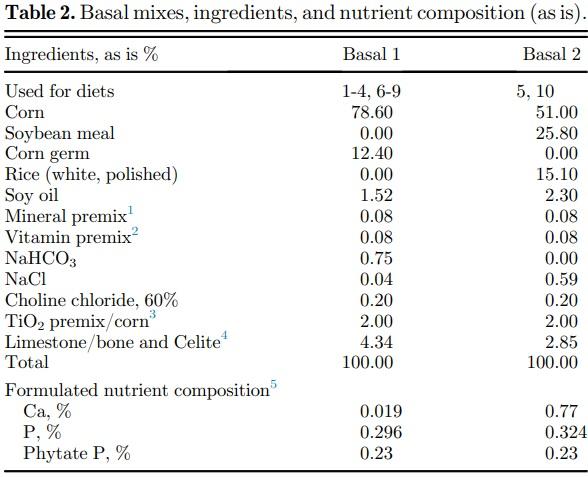
1Mineral premix supplied per kg of diet: zinc from zinc sulfate, 80 mg; manganese from manganese sulfate, 100 mg; iron from iron sulfate, 20 mg; copper from copper sulfate, 3 mg; iodine from calcium iodate, 3.9 mg; selenium from selenium sulfate, 0.3 mg.
2Vitamin premix supplied per kg of diet: vitamin A, 15,111 IU; vitamin D, 5,333 IU; vitamin E, 53.33 IU; vitamin B12, 26.66 mg; riboflavin, 17.78 mg; niacin, 71.11 mg; pantothenic acid, 24.89 mg; vitamin K3, 3.2 mg; folic acid, 2.13 mg; biotin, 0.142 mg; thiamine, 4.44 mg; pyridoxine, 6.22 mg.
3Titanium dioxide/corn mix contained 70% corn and 30% titanium dioxide. This mix was ground together 3 times through a hammer mill to remove any clumps and to maximize mix ability of titanium dioxide into complete diet.
4Limestone from the same source but of different geometric means or bone from swine meat meal ground to 151 mm GMD and Celite (filler) were added to reach 100% of final diet. Limestone and bone were added to provide a total of 0.7% Ca in the final diet.
5Analyzed values by diet are given in Table 5.
The experiment used unequal replications of TRT based on planned statistical analysis. Except for TRT 2, 3, 7, and NCaPP-Free, which had 14, 8, 14, and 6 replicates, respectively, 7 replicate pens were randomly allocated to each of the other TRT. This article contains only part of a much larger study, where TRT 2 and 7 served in all planned analysis.
Laboratory Analysis
Solubilities of the 2 LM samples and bone at 5, 15, and 30 min were determined in both the 0.2 N HCl solution (Zhang and Coon, 1997) and pH 3 buffered solution after the procedure of Kim et al. (2019).
All diets were ground to pass through a 0.5 mm screen before analysis. Samples were analyzed in duplicate except where specified otherwise. Dry matter of diets and ileal contents were determined by drying overnight in a 100°C oven (Shreve et al., 2006). Diet and ileal digesta Ca and P were determined, in triplicate, after ashing and acid digestion were analyzed using inductively coupled plasma atomic emission spectrometry (AOAC, 1999). Titanium (Ti) concentrations in diets and ileal contents were determined by a colorimetric method adopted from Short et al. (1996), where samples were first ashed at 580°C and then digested in 7.4 M H2SO4. Phytase activities in all TRT diets were determined in accordance with the ISO 30024 (2009) procedure, where one phytase unit (U) is the amount of enzyme that releases 1 mmol of inorganic orthophosphate from a sodium phytate substrate per minute at pH 5.5 and 37°C.
Calculations
Apparent ileal digestibility of Ca and P was calculated based on the following formula using TiO2 as the inert marker:
where (Min/TiO2)d is the ratio of minerals (Ca or P) to TiO2 in the diet and (Min/TiO2)i is the ratio of minerals (Ca or P) to TiO2 in the ileal digesta.
Ileal Ca and P losses were determined in broilers fed the NCaPP-Free diet:
where (TiO2)d or (TiO2)I represents TiO2 concentration in the diet or ileal digesta, respectively. Mini is the Ca or P in the ileal digesta.
Figure 1. Particle size and distribution of limestone and bone used in experimental diets. (A) Limestone 800 μm GMD (Cerne Calcium Company, Fort Dodge Fine & Granular Products, Ft Dodge, IA). l; (B) Limestone (a) ground to 150 μm; (C) Pork bone from pork bone meal ground to a 151 μm GMD.
Standardized ileal digestibility of Ca and SID P was calculated as
SID %= 5 AID-endogenous loss
Digestible Ca or P was calculated as follows:
Digestible Min (%)= (SID Min)X Mindiet
where Min is either Ca or P; SID Min is the SID of Ca or P; Mindiet is the analyzed total Ca or P concentration in the diet.
Statistical Analysis
Data were analyzed by MIXED procedures of SAS 9.4 (SAS Institute, 2014). All treatments were first analyzed by one-way ANOVA with TRT considered as the fixed effect. In addition, four 2 x 2 factorial analyses were conducted. In all analyses, pen was included in the model as a random factor. Tukey’s (Tukey, 1949) adjustment was applied in all pairwise comparisons to protect P-values. All calculations were generated based on pen averages. Significance was declared at P < 0.05.
RESULTS AND DISCUSSION
Analyzed DM, Ca, and P of tested LM, bone, and monosodium phosphate (MSP) are shown in Table 4. Analyzed dietary Ca, total P, phytate-P, and phytase concentrations were all within 3% of formulated values (Table 5). Phytase activities in nonphytase TRT were all below the detection limit of the assay, demonstrating no cross-contamination between phytase and nonphytase TRT during mixing.
Ca Source In Vitro Solubility
Solubility of 800 μm GMD LM in the pH 3 buffered solution was 32.61, 67.7, and 88.21% at 5, 15, and 30 min (Figure 2A), respectively. Reducing LM particle size to 151 μm GMD LM increased the speed of solubilization at 5 (61.2%) and 15 (86.9%) min; but extend of solubilization at 30 min remained similar (90.7%) as compared with 800 μm GMD LM (Figure 2A). A similar trend was also observed with the 0.2 N HCl solution, but with higher solubility for both LM samples at all time points and lesser difference between the 2 samples (Figure 2B). Bone did not solubilize well with either solution, its solubility at 15 min was only 2.7 and 3.2% with pH 3 buffered or 0.2 N HCl solution, respectively (data not shown). The relationship between LM particle size and solubility has been reported widely. In a recent study, Kim et al. (2019) reported that reducing LM particle size from the same LM increased LM solubility from 82 (633 μm GMD) to 91% (63.3 μm GMD) at 15 min. They also found that even though LM solubility was on average 10% lower in a pH 3 buffered solution than in a 0.2 N HCl solution, the differences were greater with slow-solubilizing LM (17%) than fast-solubilizing LM (6%). This suggests the pH 3 buffered solution, which is not only more similar to gastric conditions and pH, also has the potential to more clearly separate LM of different solubilities. These solubility assays were developed for LM, where the Ca is not contained within an organic matrix. Further solubility work in our laboratory where pepsin was added to the pH 3 buffered solution showed that the solubility of bone was 9.3, 55.1, and 87.9% at 5, 15, and 30 min, respectively (unpublished data), suggesting that a solubility method modification may be required for different Ca sources.
Impacts of Limestone Particle Size, Presence of Phytate, Ca Source, Phytate Source, and Phytase on Digestibility
The summary results of dietary impacts on SID are shown in Table 6. Dietary effects were seen for both Ca and P digestibilities (P < 0.05). Results will be discussed separately by dietary factors.
Limestone Particle Size From the previous work conducted in our laboratory, it was found that the impact of LM on ileal Ca and P digestibility was not only affected by LM particle size but also by the origin of the LM (Kim et al., 2019). Therefore, to ensure only the particle size effect would be tested in this experiment, one single source of LM was obtained for the current trial and ground to prepare 2 different particle size LM samples.
Irrespective of phytase, when the 800 μm GMD LM was included in the basal diet, both SID Ca and digestible Ca were higher than when the 151 μm GMD LM was included in the diet (49.18 vs. 39.09%, main effect, P < 0.05, Table 7). Phytase addition at 1,000 U/kg increased SID Ca by 28%, from 38.28 to 49.00% (P < 0.05, main effect). Kim et al. (2019) also found that smaller particle size of LM, which was ground from the same source, resulted in significantly lower apparent ileal Ca digestibility than larger particle size LM irrespective of phytase inclusion. Similarly, Anwar et al. (2016a) reported that, Ca digestibility for larger particle size portion (1,000–2,000 μm) of a LM was greater than for smaller particle size portion (<500 μm), irrespective of diet Ca to phytate-P ratio. However, it is important to note that all trials mentioned above only compared 2 particle sizes from the same LM source, while the correlation between LM particle size (solubility) and Ca digestibility may not be linear as demonstrated by Kim et al. (2019). Because Ca digestibility is largely affected by Ca-phytate-P interaction and possibly the passage rate of the Ca source from the gizzard, it may not be correct to assume that increasing LM particle size or a reduction in solubility would always benefit Ca digestibility. For example, Manangi and Coon (2007) reported Ca retentions to be quadratically associated with LM particle size in broilers. These authors found the lowest Ca retention with a ˜760 μm LM sample and the highest Ca retention when broilers were fed either 28 μm or 1,306 μm LM diet (Manangi and Coon, 2007), suggesting the relationship between LM particle size and Ca digestibility is not linear.
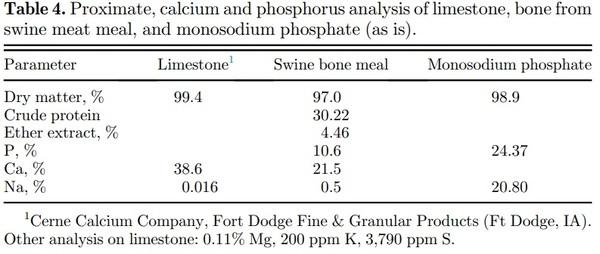
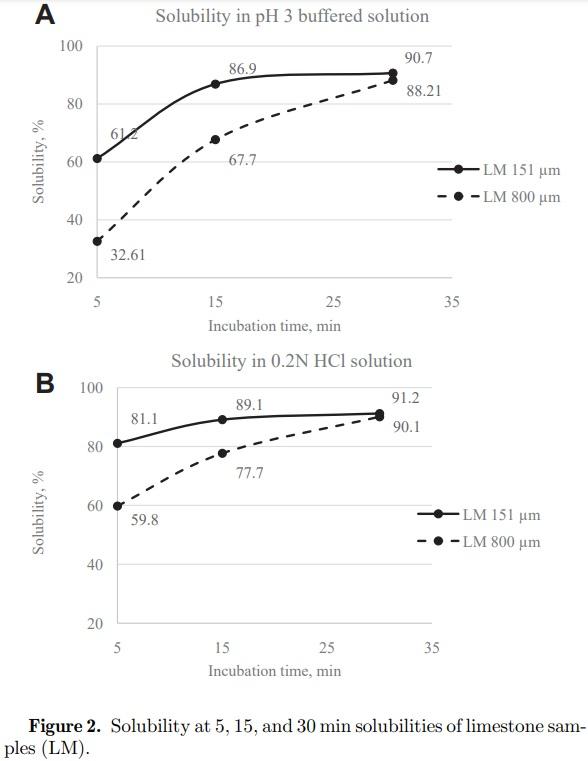
There was an interaction between LM particle size and phytase on SID P and digestible P (P < 0.05). In the absence of phytase, SID P in birds fed 151 and 800 μm GMD LM TRT was 14.08 and 24.03%, respectively (P < 0.05). Adding 1,000 U phytase/kg resulted in a 63.44 percentage point improvement in SID P when the 800 μm GMD LM diet (87.47%) was fed vs. 46.76 percentage point improvement in SID P (60.84%) when birds were fed the 151 μm GMD LM diet (P < 0.05). Previous work has shown that Ca interacts with phytate and reduces its bioavailability (Cheryan and Rackis, 1980; Graf and Eaton 1984). The degree of Ca-phytate interaction is largely dependent on [Ca]2+, or reactive Ca concentration which represents, in the case of LM, the amount of solubilized Ca from LM, on the phytic acid concentration and on the pH (Cheryan and Rackis, 1980; Graf and Eaton, 1984). Limestone Ca solubility is highest at lower pHs and thus LM solubility would have the greatest effect in the gastric portion of the digestive tract where pH are low and where exogenous phytases would be most active. Reducing particle size from the same LM source resulted in higher solubility of the LM at 5 min, which results in higher [Ca]2+ and consequently greater impact of Ca on P digestibility (Kim et al., 2018, 2019). Results from the present and previous studies (Kim et al., 2018, 2019) suggest that increased LM solubility, because of reduced LM particle size, from the same LM is not beneficial to Ca digestibility and in fact detrimental. This effect was seen in studies where phytate source was from corn SBM based diets (Kim et al., 2018) and in the present study where the effect of particle size was studied in a diet containing only corn phytate.
Ca Source Two Ca sources were tested in this trial, bone from swine meat meal and LM both taken to the same GMD (150 μm) to avoid possible particle size confounding effects. There were interactions between Ca source and phytase for both SID Ca and P (P < 0.05, Table 8). Irrespective of phytase, Ca from bone was more digestible than from LM (P < 0.05). Apparent ileal Ca digestibility of meat and bone meal was reported to be between 44.6 and 56% by Anwar et al. (2016b), which is similar but slightly lower than current bone SID Ca value. This difference may be in part because in the present study, bone separated off from meat and bone meal was tested while Anwar et al. (2016b) tested meat and bone meal. In addition, methodology differences may account for lower digestibilities reported by Anwar et al. (2016b), where Ca concentration used in their study, carried out from 27 to 31 d of age, was formulated to 0.83% but varied between 0.80 and 0.99 for the different meat and bone meals. Experimental diets in this research were tested between day 20 and 22 of age and were formulated to contain 0.70% Ca (0.73% Ca by analysis for the bone containing diet). Phytase increased LM SID Ca from 33.56 to 42.62% (P < 0.05) but had no effect on SID Ca originating from bone, which was 68.68 and 70.42% at 0 and 1,000 U phytase/kg, respectively. These data suggest an important and large difference in the SID Ca response to phytase that appears to be dependent on Ca source and is potentially related to the reactivity of the Ca in the different Ca sources. Reactivity will be related to solubility, as it refers to ionized Ca or reactive calcium from LM that is directly related to solubility. For bone the solubility at 5 min at pH 3 was very low (<10%) regardless of solution used. This is due in part to the fact that Ca in bone is contained with an organic matrix mainly containing proteins and thus a different solubility assay is needed to determine Ca solubility from bone. Work performed in our laboratory after this study showed that when pepsin was used in the solution the solubility of the same bone preparation used in this study bone was 9.3, 55.1, and 87.9% at 5, 15, and 30 min, respectively (unpublished data). Low reactivity or solubility quickly after a LM reaches the low pH in the proventriculus and gizzard would be related to less potential chelation between the soluble Ca ions and phytic acid. For LM, a low (<10%) initial (5 min) solubility would have a positive impact on P digestibility and phytase efficacy but it will be important to have a high (>80%) 30 min solubility a 30 min solubility has a large impact on LM Ca digestibility.
When there was no added Ca from LM or bone, SID P from the corn-based diet was 72.18 and 91.13% with phytase added at 0 and 1,000 U/kg, respectively (P < 0.05, Table 8), suggesting that phytate P could be highly digestible for broiler birds under the right circumstance. Similar high Ca digestibilities in the absence of added Ca from LM have been reported previously (Tamim and Angel 2003; Tamim et al., 2004). Adding either Ca from LM or bone reduced SID P by as much as 81% (TRT 4) when no phytase was added (P < 0.05), suggesting Ca, irrespective of source has a substantial effect, on SID P. But most importantly, the effect is much different in magnitude depending on Ca source, with LM Ca having a much greater negative effect on SID P than bone Ca (Table 8). The effect of Ca is likely driven, in part, by the interaction between Ca and phytate with solubility or reactivity of the Ca in the source studied at low pH impacting this interaction. The source of Ca also affected the magnitude of response in SID P with a substantially (P < 0.01) smaller improvement in SID P of 16.57 percentage points from phytase (50.50–67.07%) when the dietary Ca originated from bone vs. an improvement in SID P of 46.76 percentage points (14.08 vs. 60.84%) when Ca originated from LM. It is important to note that even though the phytate concentration was the same in these diets, total P was much higher in the diet containing bone (0.505%) vs. that containing LM (0.291%). It is known that dietary inorganic P concentration in the diet affects P digestibility (Li et al., 2014; Sommerfeld et al., 2018); therefore, the reduced response of SID P to phytase may be in part attributed to higher inorganic P in the bone TRT.
Presence of Phytate The impact of phytate on SID Ca and P was compared between phytate from corn-based diets and phytate-free diets with 2 different particle size LM, from the same LM batch (Table 9). There was no interaction between phytate and LM GMD (P > 0.05). The SID Ca of birds fed the smaller particle size LM (151 µm GMD) diet, irrespective of phytate concentration, was lower (53.23%) than that of the 800 µm GMD LM (63.64%, P < 0.05, main effect). In the absence of phytate, SID Ca from LM was 78.59% but was reduced by 51 to 38.28% in the presence of phytate from corn (P < 0.05, main effect). A similar effect was reported by Tamim et al. (2004).
There was an interaction between phytate source and LM GMD on SID P (P < 0.01, Table 9). When LM was added to diets with phytate from corn, LM particle size affected SID P (P < 0.01), being lower when diets contained smaller particle size LM. The interaction (P < 0.01) of the presence of phytate and LM particle size supports the previously described interaction of Ca and phytate, being more pronounced when fine LM was more rapidly solubilized. By contrast, the SID P of diets with no phytate was not affected by LM particle size (P > 0.05, Table 9), being 91.63% on average. Because all P was from MSP in the phytate-free diets, the SID P represents P digestibility from MSP. Using a linear regression method, Shastak et al. (2012) reported the prececal P digestibility of MSP of 53% in 25-day-old broilers fed corn/SBM-based diet, which was substantially lower than the current results. The discrepancies may be attributed to the basal diet formulation (phytate-free vs. corn/SBM) used, specific process used to make the MSP (Viljoen, 2001), and digestibility methods used (36 h vs. 10 d feeding). In support of our data, Van der Klis and Versteegh (1996) give a 92% P digestibility for MSP and the Dutch feed tables (CVB, 2016) reported retained P digestibility of MSP.1H2O to be 91%, which is close to the value obtained in the present study.
Phytate Source. There was a main effect (P < 0.01) of phytate source and phytase on SID Ca but no (P > 0.05) interaction (Table 10). Across phytase concentrations, diets containing phytate originating from both corn and SBM had a higher SID Ca of 55.92 vs 49.18% when the phytate originated only from corn (P < 0.05). Phytase inclusion at 1,000 U/kg increased SID Ca from 46.16 to 58.94% (P < 0.05, main effect), suggesting that the improvement in SID Ca was similar regardless of phytate source (SBM/corn vs. corn only). In the absence of phytase, the diet containing phytate from corn had a lower (24.03%) SID P than when diets contained phytate from both corn and SBM (32.46%, P < 0.05). Greater improvement on SID P in the presence of phytase were seen when corn was used as the sole phytate source as compared with the combination of corn/SBM (P < 0.05) mainly because in the absence of phytase corn phytate is so poorly digested. Similar results were also reported by Leske and Coon (1999) where fecal myoinositol hexakisphosphate hydrolysis was lower, irrespective of phytase (600 Escherichia coli phytase U/kg) for corn vs. SBM in both broiler and laying hens. These results suggest that the effects of phytate on SID P, and similarly, the magnitude of the phytase effect on SID P are dependent on the source of phytate in the diet.
CONCLUSION
Based on the results of this experiment, one can surmise that 1) reducing LM particle size did not only reduce SID Ca but also had a greater negative impact on SID P; 2) Ca-phytate interaction is an important cause of the relatively low LM Ca or phytate-P utilization in broilers, without which, both are much more digestible; 3) the degree of Ca-phytate interaction and the response to phytase is dependent on both Ca and phytate source.