1. Introduction
To preserve the potency of clinically important antibiotics, there has been a gradual reduction and an anticipated elimination of their use in food-producing animals including broiler chickens [1]. Consequently, farmers are faced with the challenge of maintaining productivity and preventing diseases on their farms [2]. Therefore, there is a need for alternative strategies including dietary means to promote gut health and growth performance of broiler chickens. High fiber ingredients have been shown to have prebiotic effects and their use in animal production could promote gut health and growth performance [3]. Oat (Avena sativa) hulls have high levels of lignin, an insoluble fiber [4,5], and have potential to modulate gastrointestinal microbiota and increase short chain fatty acids (SCFA) production, thus enhancing gut barrier integrity [3]. Short chain fatty acids have been shown to have beneficial effects on gut health through the interactions between diet, gut microbiota, the intestinal barrier and immunity [6]. By reducing the pH in the gut, SCFA helped to modify the composition of gut microbiota [6] and prevented the growth of pathogenic bacteria that are sensitive to pH [7]. Oat hulls (OH) are byproducts of grain milling, which are not substantially used in the food chain, thus their use in the poultry industry could also help to reduce production costs. Many studies have reported the beneficial effects of inclusion of moderate amounts of OH on the growth performance of chickens. For example, an improvement in feed conversion ratio (FCR), nutrient digestibility, reduced digesta pH, and increased gizzard weight have been observed when 2.5%, 3%, 5% or 7.5% OH were fed to broiler chickens [8–10]. An improved jejunal villi surface area in chickens fed 5% OH was also observed by Torki et al. [11]. There is dearth of information in the literature on the effect of OH on gastrointestinal SCFA. Free choice feeding of OH was beneficial in protecting broiler chickens against necrotic enteritis challenge [12]. However, it is not known if the beneficial effects of OH reported by Kheravii et al. [12] was as a result of OH per se or as a result of its free choice feeding. It has been shown that young broiler chickens may have a requirement for dietary fiber to optimize nutrient digestibility and growth performance [8]. This requirement is not yet known but studies have demonstrated the ability of poultry to self-select their own diets in order to meet their nutritional requirements [13]. Offering fiber ingredients as free choice to starter chicks could offer them freedom to eat just the amount needed without associated digestive disturbance. Thus, it seems possible that the free choice feeding of OH to broiler chickens might have more gastrointestinal benefits compared to when it is incorporated in the basal diet, but data in this field are scarce and more research is needed. No study has determined the effect of OH fed as free choice compared with OH incorporated in diets for broiler chickens, to our knowledge. The objective of this study was therefore to determine the effect of OH incorporated in the diet or fed as free choice on growth performance, carcass yield, organ weights, digesta pH, SCFA production and intestinal morphology in broiler chickens.
2. Materials and Methods
2.1. Ethical Approval
The experimental procedures for this study were approved by Dalhousie University Animal Use and Care Committee and chickens were cared for in accordance with the guidelines of the Canadian Council on Animal Care [14].
2.2. Birds and Housing
A total of 648 day old, mixed sex broiler chickens (Ross 308) were obtained from Atlantic Poultry Inc. Port Williams, Nova Scotia. Upon arrival, chicks were weighed in groups of 27 birds and assigned to floor pens (0.93 m × 2.14 m), at a stocking density of 0.076 m2/ bird. Room temperature was monitored daily and was gradually reduced from 30 to 22.6 °C from d 0 to 36. The lighting program was set to produce 18 hours of light and 6 hours of darkness throughout the experimental period and illumination was gradually reduced from 20 lx on d 0 to 5 lx on d 39.
2.3. Diets and Experimental Design
The OH used in this study was obtained from Grain Millers, Yorton, Saskatchewan, Canada. They were obtained as fine particle sized (6/64 round hole and a 7/64 round hole). Four dietary treatments were assigned to 24 pens in a completely randomized design. The dietary treatments consisted of a corn–soybean meal–wheat based diet (Basal), Basal + bacitracin methylene disalicylate (BMD), Basal + 3% OH (3% OH) and Basal + free choice OH (FCOH). Diets were iso-energetic and iso-nitrogenous and formulated on digestible amino acids and apparent metabolizable energy (AME) bases and met or exceeded National Research Council (NRC) [15] nutrient requirements for broiler chickens. The FCOH was provided in extra feeding troughs attached to the pens, in addition to the feeding trough that supplied the basal diet. Birds were fed using a phase-feeding program that consisted of starter phase (d 0 to 14), grower phase (d 14 to 22), and finisher phase (d 22 to 39). The ingredient and nutritional compositions of the diets in phases 1 to 3 are presented in Table 1. The OH samples and diets were finely ground and were all analyzed for dry matter (DM), crude protein (CP), neutral detergent fiber (NDF), acid detergent fiber (ADF), ash, fat, and minerals. Dry matter was determined according to Association of Official Analytical Chemists (AOAC) [16] method (925.09) by oven drying a 5.0 g sample at 105 °C overnight.
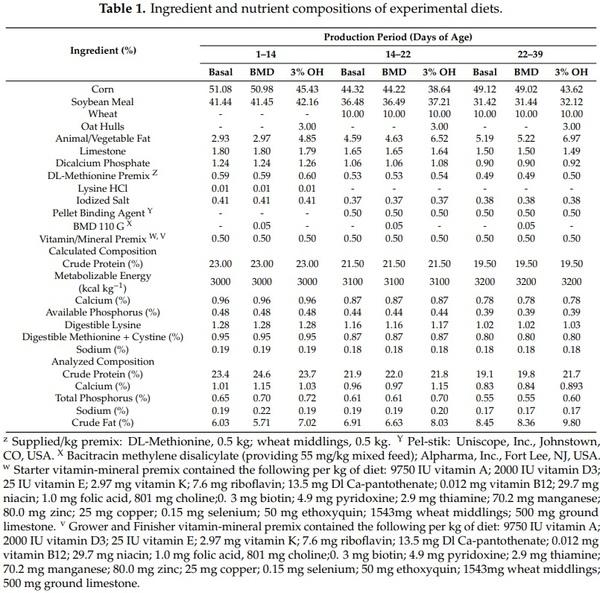
The method of Goering and Van Soest [17] was used for the determination of NDF and ADF. Nitrogen content in OH and diet samples was determined using the combustion method (990.03) [16] with an N analyzer (Model Leco CN828 Carbon Nitrogen Determinator, St. Joseph, MO) and CP was calculated as N × 6.25. The ether extract in samples was determined after hexane extraction (Method 920.39) [16] in an Ankom XT10 Fat Extractor system (Macedon, NY). Samples were analyzed for minerals (Ca, Na, K, Mg, p, Mn and Zn) after they were ashed at 600 °C for 12 h in a muffle furnace, using a Varian 725 ICP-OES inductively coupled plasma mass spectrometer (ICP-AES; Vista, Varian, Palo Alto, CA) according to the method of AOAC [18] (method 985.01). The analyzed composition of OH used in the study was presented in Table 2.
2.4. Measurements and Sampling
Body weight (BW) and feed intake (FI) were determined weekly on a pen basis and mortality was recorded daily to calculate body weight gain (BWG), and FCR. Intake of OH for the FCOH group was also measured weekly and used in the calculation of FI and FCR. On d 36, one chicken was randomly selected from each pen, individually weighed and euthanized by electrical stunning and exsanguination. After slaughter, liver, spleen, gizzard, bursa of Fabricius, ceca, and the entire intestines were removed and weighed by trained personnel. The length of the entire intestine was measured. Digesta from ileum and ceca were collected separately and their pH values were determined (Oakton™ Waterproof Big Display pHTestr™ 30, Fisher Scientific). Digesta from ceca was collected and stored in plastic sterile tubes, placed in liquid nitrogen and stored at −80 °C for analysis of SCFA. Necrotic lesions in the intestinal tract were graded according to the procedure of Shojadoost et al. [19] as follows: zero—no gross lesions; one—thin-walled or friable small intestine; two—focal necrosis or ulceration; three—larger patches of necrosis; and four—severe, extensive necrosis typical of field cases. The jejunum was taken distally from the pancreatic loop to Meckel’s diverticulum and a sample of 0.5 cm in length was taken from the center and placed in 10% buffered formalin for histological measurements. For processing, these samples were placed in acetic acid/ethanol (25/75, v/v), for 24 h, rehydrated in a bath of ethanol/water (50/50, v/v) and finally in distilled water. On d 39, two male birds per pen were slaughtered after subjection to 12 h fasting. Birds were individually weighed before electrical stunning, bled for 3 minutes after carotid and jugular veins were cut, scalded at 60 °C for 90 s, and mechanically defeathered. Carcasses were manually eviscerated and then chilled at 4 °C for 3 h before processing. Carcasses were weighed before and after chilling to determine hot and cold carcass weights as well as weights of breast muscle.
2.5. Analysis of Short Chain Fatty AcidsCecal digesta samples were homogenized in an ethanol/20 mM phosphate buffer (85/15) buffer at a concentration of 1 mg sample/3 µL of buffer using a Precellys bead homogenizer (3 × 20 s at 5500 rpm with a 30 s pause between steps). The homogenization tubes were centrifuged at 4 °C for 10 m at 800× g and the supernatant transferred to 1.5 mL tubes. These tubes were then centrifuged at 4 °C for 10 m at 20,000× g and the supernatant transferred to a new set of 1.5 mL tubes. 25 µL of cecal digesta sample was taken for analysis; the equivalent of 8.33 mg of sample. Each sample tube had 25 µL of sample added followed by 20 µL of internal standard mixture (2000 ng/20 µL each of acetic acid-d4 and butyric acid-d7). A standard curve from 20 to 100,000 ng each of acetic acid, propionic acid, and butyric acid was also prepared. Samples and standards were treated to the same extraction conditions as follows: each tube had 75 µL of water added followed by acidification with 1M HCl and immediate addition of 200 µL of methyl-tert-butylether. Tubes were vortexed for 1 min on a multi-tube vortexer and then centrifuged at 4 °C for 10 m at 20,000× g. The supernatant was transferred to an auto sampler vial with an insert and injected for analysis by GC-MS.
2.6. Measurement of Jejunal Morphology
The specimens of mid jejunum were embedded in paraffin, sectioned (5 µm), and stained with Alcian blue (pH 2.5) according to the procedures of Shang et al. (2015). Morphological measurement of villus height, crypt depth, and total mucosal thickness was conducted using an image processing and analysis system (ImageJ, WI, USA). The ratio of villus height to crypt depth was calculated by dividing the villus height with the crypt depth. The total mucosal thickness was measured as the entire mucosal length (Villus height + crypt depth).
2.7. Statistical Analyses
A one-way analysis of variance was carried out using the mixed procedure of Statistical Analysis System (SAS) with treatments Basal, BMD, 3% OH or FCOH as factors and the following parameters as variables: feed intake, body weight gain, feed conversion ratio, jejunal morphology, digesta pH, organ weights, carcass characteristics, and concentrations of SCFA. A normal probability plot of residuals was used to ascertain the normality of all datasets in the same statistical package. Following ANOVA, differences between significant means were tested using Tukey’s honestly significant difference (HSD) test in the same statistical package. Analyzed data were presented as means, standard error of the means (SEM) and probability values. Values were considered statistically different at p ≤ 0.05 and considered as trend at p < 0.1.
3. Results
3.1. Effect of Oat Hulls on Growth Performance and Carcass Yield
As presented in Table 3, there was no effect of dietary treatment on FI except on d 28 when birds that had access to FCOH had higher FI compared to those fed 3% OH, while those fed basal and BMD diets were intermediate. On d 8 and 36, the BWG of birds fed Basal, BMD and 3% OH was not different but was significantly higher than those fed FCOH. On d 15, birds fed FCOH had lower BWG than those fed 3% OH. For the overall BWG, FCOH was significantly lower than BMD and 3% OH but not different from the basal diet. Between d 22 and 28 birds fed FCOH had higher feed intake than those fed 3% OH. On d 8 and 15, the lowest (best) FCR was observed for birds fed 3% OH, while the highest was recorded for those fed FCOH. However, FCOH significantly increased (p < 0.05) FCR only during the grower and finisher phases. As illustrated in Table 4, among the birds slaughtered on d 39, those fed 3% OH had the highest hot carcass, cold carcass and breast muscle weights, which were significantly different from those fed the FCOH. However, there was no difference in carcass yield among treatments.
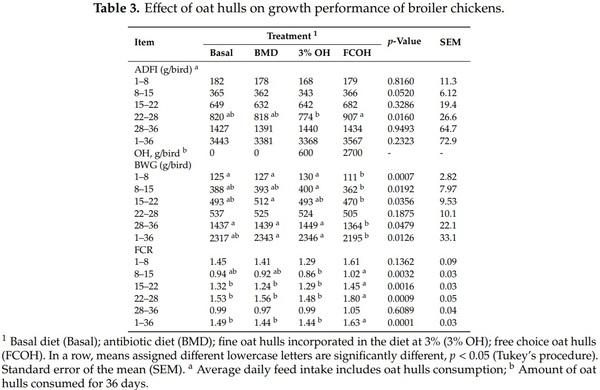
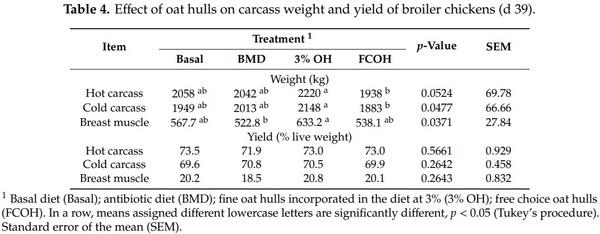
3.2. Effect of Oat Hulls on Intestinal Parameters
As presented in Table 5, there was no significant difference among treatments in the relative weights of spleen, liver, bursa, pancreas and entire intestine. Birds that had access to FCOH had higher (p < 0.0001) gizzard relative weight and showed a tendency (p = 0.0900) to have higher cecal weight, compared to the other treatment groups. There was no significant difference among treatments in intestinal length and necrotic enteritis score. However, birds fed BMD had a numerically lower score (0.33) than those on other treatments (0.83–0.92) (p = 0.1938). As shown in Table 6, there were no significant differences among treatments in villus height, crypt depth villus height to crypt depth ratio and total mucosal thickness. As illustrated in Table 7, there was no effect of treatment on ileal and ceca pH and concentrations of SCFA.
4. Discussion
Oat hulls represent the largest proportion of byproducts from the oat-milling operation [20]. If obtained from good quality oats, the OH may be as low as 25%, but values ranging from 20% to 36% have been reported in the past [21–23]. Oat hulls disposal can be a serious concern for oat millers because of this large volume of waste (Girardet and Webster, 2011). The chemical composition of the OH used in the current study was similar to those reported by Garcia et al. [24], Jimenez-Monero et al. [8] and Mossami [25]. Compared to the basal and BMD diets, the inclusion of 3% OH did not affect FI, BWG and FCR, although birds fed the 3% OH diet had the numerically highest BWG and the best FCR. Hetland and Svihus [26] and Mossami [25] reported that the inclusion of 4% OH increased FI without affecting the BWG of broiler chickens. The reason why FI was not affected in the current study could be because of the lower inclusion level of OH. It should also be noted that the OH diet in the current study was formulated to be isocaloric and isonitrogenous with the basal and BMD diets, which was not the case in the studies of Hetland and Svihus [26] and Mossami [25]. Chickens would normally compensate for decreased nutrient concentration in diluted OH diets by increasing their FI. Similar to the current study, Garcia et al. (2019) did not observe any effect on growth performance when 3% OH was fed to broiler chickens. Jimenez-Moreno et al. [8] did not observe any effect of OH included at 2.5, 5.0, or 7.5 % on FI and BWG, although an improvement in FCR was observed. Compared to the FCOH group, birds fed 3% OH, BMD, and Basal diets had higher BWG and better FCR throughout the study. During d 22 to 28, birds that had access to FCOH consumed more feed compared to those fed 3% OH, though this increase in FI intake did not result in better BWG and FCR. This suggested that birds that had access to FCOH consumed more OH at the expense of the basal diet during that period. To our knowledge, Kheravii et al. [12] was the only study in the literature that investigated FCOH in chickens. They reported that birds that had access to FCOH had lower FI and tended to have lower FCR compared to those that did not have access to FCOH. Although Kheravii et al. [12] did not report the quantity of OH consumed, the current study observed that birds that had access to FCOH consumed more than fourfold OH compared to those fed 3% OH (2700 vs 600 g/bird). During the starter phase, access to FCOH did not have a negative effect on FCR. This shows that FCOH may be more beneficial to starter chicks. This is consistent with the report of Sadeghi et al. [27] that broiler chicks preferred to consume lower separate sources of insoluble fiber at younger ages of 1 to 14 d. Kheravii et al. [12] also observed that improvement in growth performance due to FCOH was more pronounced in young broilers. During the grower and finisher phases, birds in the FCOH group consumed more OH at the expense of the nutritionally balanced basal diet, which resulted in nutrient dilution and less efficiency in converting feed to gains.
Compared to the basal and BMD diets, birds fed 3% OH had similar carcass and breast muscle weights. However, birds fed 3% OH had the numerical highest hot carcass weight (7.9% higher than the basal diet), cold carcass (10.2% higher than the basal diet) and breast muscle weights, which were significantly higher (p ≤ 0.05) when compared with the FCOH group. However, there were no significant differences in carcass yield (% live weight). Lonkar et al. [28] reported that the inclusion of 2% OH in broiler diet showed a significant improvement in ready-to-cook yield and a numerical improvement in carcass eviscerated and giblet yield. This has not been the case with high fiber inclusion levels. Shahin and Abdelazim [29] demonstrated that a high fiber diet (8% inclusion) suppressed carcass weight compared to low fiber (4% inclusion). Mourao et al. [30] also indicated that broilers fed 5–10% citrus pulp and dehydrated pasture had lower carcass weights and carcass relative live weights due to a more developed gastrointestinal tract.
Our findings showed that birds that had access to FCOH had increased gizzard weight and tended to have increased cecal weight. This is consistent with the report of Kheravii et al. [12] that birds that had access to FCOH had heavier gizzards compared to those that did not have access. A diet containing OH would be retained longer in the gizzard due to being much lignified and more resistant to grinding as reported by Rogel et al. [31,32]. A large and well-developed gizzard would be able to completely grind feed particles and improve nutrient digestibility and utilization compared to smaller ones. This is consistent with the fact that birds in the FCOH consumed 2100 g/bird more OH than those in the 3% OH group, in the current study. There was no effect of OH on entire intestinal length and weight in the current study. Previous studies have shown that increased intestinal length is more associated with feeding soluble fiber than insoluble fiber. For example, Jimenez-Moreno et al. [9] reported longer intestines in chicks fed sugar beet pulp (containing soluble fiber) than those fed rice hulls (containing insoluble fiber). Similarly, Iji et al. [33] reported that broilers fed a higher soluble fiber type had greater intestinal weight. Oat hulls contained 535 g/kg insoluble non-starch polysaccharides and no soluble non-starch polysaccharides were detected [12]. There was no effect of treatment on jejunal morphology in the current study. Similarly, Torki et al. [11] and Jimenez-Moreno et al. [9] did not detect any effect of OH inclusion on the jejunal villus height of the gastrointestinal tract of broilers. Kimiaeitalab et al. [34] also did not observe any effect of sunflower hull inclusion on the ileal mucosal morphology of broiler chickens. An increased jejunal villus height is indicative of a higher absorptive surface area of the jejunum. Previous studies have reported that feeding soluble fiber reduced intestinal villus height, while insoluble fiber did not [27,33]. Thus, it seems that the negative effect of dietary fiber on intestinal villus height reported in previous studies is more related to an increase in digesta viscosity, which is usually associated with soluble fiber.
Digesta pH can be an indicator of the proliferation of acid-intolerant pathogenic bacteria or the presence of SCFA. The current study did not observe any effects of OH on digesta pH and ceca SCFA. Similarly, Kimiaeitalab et al. [34] did not observe any effect of 3% sunflower hull inclusion in diets for broilers or pullets on SCFA concentration, but observed higher SCFA concentrations in pullets compared to broilers. Kheravii et al. [12] reported that broilers given FCOH had lower concentrations of acetic, propionic and valeric acid, but a higher concentration of succinic acid compared to those without access to FCOH. It should be noted that the study of Kheravii et al. [12] involved necrotic enteritis challenge, which could have stimulated the production of succinic acid, which was suggested to have immunomodulatory effects among the challenge birds. It has been reported that succinate or succinic acid increased pro-inflammatory cytokines such as interleukin−8 and interleukin−1b [35,36]. Several factors may affect the concentrations and proportions of SCFA in birds and these include sites of fermentation in the gut (which changes with age), the type and amount of the fiber ingredient, microbiome composition, and the age of birds [37–39].
5. Conclusions
The inclusion of 3% OH in diets for broiler chickens has the potential to improve growth performance and carcass weight. Oat hulls fed as free choice increased gizzard weight in broiler chickens but reduced FCR in the grower and finisher phases. There was no effect of OH incorporated in the diet at 3% or fed as free choice on jejunal morphology, digesta pH and cecal SCFA. Further research on the effect of different physical forms of OH on cecal microbiota is underway to elucidate the reason for the lack of effect on cecal SCFA.
This article was originally published in Sustainability 2020, 12, 3744; doi:10.3390/su12093744. This is an Open Access article distributed under the terms and conditions of the Creative Commons Attribution (CC BY) license (http://creativecommons.org/licenses/by/4.0/).