INTRODUCTION
Least cost feed formulation relies on the assumption of additivity of digestible nutrients from the contributing ingredients. It is generally accepted that assessment of the nutritional value of individual raw materials underestimates their eventual contribution in a complex diet because of the often-substantial influence of endogenous loss. The premise is that a proportion of nutrients that leave the terminal ileum are inevitable (or “basal”) losses and diet-independent and thus will not be arithmetically additive when single ingredients are combined in a complete diet. However, assessment of these “basal” endogenous losses is not trivial and requires some compromise on methodological approach (Ravindran et al., 2004; Ravindran and Hendricks, 2004). Despite this, the importance of ensuring additivity in formulations has prompted a collaborative initiative to explore the potential of standardizing methodology for the assessment of digestibility of amino acid (AA) to account for basal endogenous AA (EAA) loss (Ravindran et al., 2017). It is likely that similar adjustments will eventually prove necessary for energy systems and perhaps also for other nutrients such as calcium and phosphorus.
Instructively, Kong and Adeola (2013a,b) noted that the apparent ileal digestibility (AID) of AA in corn and soybean meal (SBM) was undervalued by around 1 to 2% when individually assessed compared with their contribution in a corn/SBM mixture but that this discrepancy was rectified when standardized ileal digestibility (SID) values were used. More recently, Cowieson et al. (2019) observed that the AID of AA in corn and SBM were undervalued by around 7% compared with a mixture of corn and SBM (65% corn and 35% SBM) but that this was also resolved by assessment of SID values for AA. The consequence of this observation is that when diets are formulated using AID values for single ingredients, the final diet will be overformulated in digestible AA (especially for those AA that are commonly associated with EAA flow, e.g., Thr, Gly, Ser, Asp; Cowieson et al., 2009). Furthermore, when digestibility-modifying zootechnical additives such as feed enzymes are applied under AID-based systems of formulation, it may be that effects are difficult to interpret because of lack of precision in the formulation of the underlying control diets.
The fact that exogenous enzymes may influence endogenous loss has been known for some time though to what extent this may considered “basal” compared with “specific” is not clear. For example, Cowieson and Ravindran (2007) noted that exogenous phytase was capable of reducing endogenous nitrogen (N) and AA flows in broilers by 15 to 25%. Cowieson and Roos (2016) summarized similar effects for an exogenous protease where changes in intestinal morphology and mucin production were observed in several studies, implying a mode of action that involves endogenous nutrient flow and not exclusively changes to exogenous nutrient digestibility. Technically, these changes would be more accurately defined as “specific” or “diet-dependent” endogenous loss rather than “basal”, but clearly the capacity of an enzyme to modify secretory and absorptive infrastructure in an animal has implications for the value of an enzyme in feed formulation. In short, it is possible that formulating diets with enzymes would be more accurate if a standardized system for feed enzymes could be developed that would take into account the overlap in mechanism between exogenous and endogenous nutrient flows. It was therefore the purpose of this experiment to expand previous work (Cowieson et al., 2019) which explored additivity of AID or SID AA values in corn, SBM, or a mixture of corn and SBM in growing broiler chickens to include effects of exogenous phytase and protease. It was hypothesized that the effect of phytase and protease on corn and SBM when fed as single ingredients may not be fully additive when these ingredients were fed as a mixed diet. Furthermore, any nonarithmetic outcomes (subadditivity or synergy) may be AA-dependent and that these patterns may reflect the AA composition of endogenous proteins.
MATERIALS AND METHODS
Birds and Diets
The study procedures were reviewed and approved by the Massey University Animal Ethics Committee and complied with the New Zealand Code of Practice for the Care and Use of Animals for Scientific Purposes.
A total of 468 male Ross 308 broiler chicks were obtained at day 1 of age from a commercial hatchery and reared in an environmentally controlled room until day 21. A pre-experimental corn–SBM–based broiler starter diet that met all the nutrient requirements (Aviagen, Newbridge, UK) was fed from day 1 to 21. This diet was formulated to contain an AME of 3,050 kcal/kg, 225 g/kg crude protein, 9 g/kg calcium, 4.5 g/kg nonphytate phosphorus, and 12.5 g/kg digestible Lys. On day 21, birds were randomly distributed to 78 wirefloored metabolism cages (6 birds per cage) and offered one of 12 experimental diets (Table 1; 6 replicate cages per diet) from day 21 to 28 or a N-free diet (from day 25–28). The experimental diets were based on the standardized ileal AA digestibility assay protocol (Ravindran et al., 2017) and were based on corn, SBM, or a mixture of corn and SBM (Table 1). Each diet was fed without or with phytase (RONOZYME HiPhos, DSM Nutritional Products, Kaiseraugst, Switzerland, 3,000 FYT/kg feed), protease (RONOZYME ProAct, DSM Nutritional Products, 15,000 PROT/kg feed), or a combination of phytase and protease (at the same inclusion concentration). One protease unit (PROT) is defined as the amount of enzyme that releases 1 mmol of p-nitroaniline from 1 mM substrate (Suc-Ala-AlaPro-Phe-pNA) per minute at pH 9.0 and 37°C. One phytase unit (FYT) is defined as the quantity of enzyme which liberates 1 μmol of inorganic phosphate per minute from 5.0 μmol/L sodium phytate at pH 5.5 and 37°C. Birds that received the N-free diet received the commercial starter diet until day 25 (Ravindran et al., 2017). All diets contained titanium dioxide (TiO2; 5 g/kg) as an indigestible marker.
The cages were housed in an environmentally controlled room. Temperature was maintained at 32°C on day 1 and gradually reduced to 24°C by day 21 and further to 21°C by day 28. Temperature modification was achieved by the use of thermostatically controlled fans and electric heaters. The birds received 20 h fluorescent illumination per d and were allowed free access to feed and water. Birds were checked at least 3 times per day (09.00, 13.00 and 16.00 h), and any unusual aspect of bird behavior or condition was recorded.
Measurements
On day 28, all birds from each replicate cage were euthanized by intracardial injection of sodium pentobarbitone for ileal digesta collection. The small intestine was immediately exposed, and the contents of the distal half of the ileum were collected by gently flushing with distilled water into plastic containers. The ileum was defined as that portion of the small intestine extending from vitelline diverticulum to a point 40 mm proximal to the ileo-cecal junction. Digesta from birds within a cage were pooled, resulting in 6 samples per dietary treatment. The digesta samples were frozen immediately after collection, lyophilized, and processed. Samples of digesta and diets were analyzed for titanium (Ti), N, or AA, including methionine and cysteine.
Chemical Analysis
Dry matter was determined using standard procedures (Method 930.15; AOAC, 2005). Samples were assayed for Ti on a UV spectrophotometer following the method of Short et al. (1996). Nitrogen was determined by combustion (Method 968.06; AOAC, 2005) using a carbon nanosphere-200 carbon, N, and sulphur autoanalyser (LECO Corporation, St. Joseph, MI). Total starch was determined using the assay procedure (Megazyme Total Starch Assay Procedure; Megazyme International Ireland Ltd., Wicklow, Ireland) based on thermostable α-amylase and amyloglucosidase. Fat was determined using the Soxhlet extraction (Method 991.36; AOAC, 2005). Calcium and P were determined by colorimetric methods after ashing the samples at 550C and acid digestion in 6.0 mol hydrochloric acid (Method 968.08D; AOAC, 2005).
Table 1. Composition1 (g/kg) of the experimental diets used in the ileal digestibility assay (21–28 D of age).
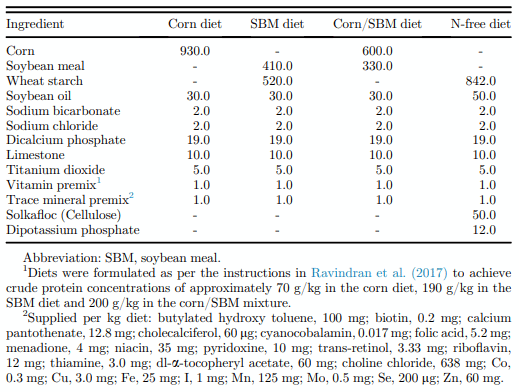
Amino acids (including proline) were determined by hydrolyzing the samples with 6 N HCl (containing phenol) for 24 h at 110°C ± 2°C in glass tubes sealed under vacuum. Amino acids were detected on a Waters ionexchange HPLC system, and the chromatograms were integrated by using dedicated software (Millenium, version 3.05.01, Waters, Millipore, Milford, MA) with the AA identified and quantified by using a standard AA mixture (product no. A2908, Sigma, St. Louis, MO). The HPLC system consisted of anion-exchange column, 2 510 pumps, a Waters 715 ultra-WISP sample processor, a column heater, a postcolumn reaction coil heater, a ninhydrin pump, and a dual-wavelength detector. Amino acids were eluted by a gradient of pH 3.3 sodium citrate eluent to pH 9.8 sodium borate eluent at a flow rate of 0.4 mL/min and a column temperature of 60°C. Cysteine and methionine were analyzed as cysteic acid and methionine sulfone, respectively, by oxidation with performic acid for 16 h at 0°C and neutralization with hydrobromic acid before hydrolysis.
Calculations
The AID of AA was calculated by the following formula using the titanium marker ratio in the diet and ileal digesta.
Where, (AA/Ti)d 5 ratio of amino acid and titanium in diet and (AA/Ti)i 5 ratio of amino acid and titanium in ileal digesta.
The basal EAA flow at the terminal ileum was calculated as grams lost per kilogram of DM intake (DMI; Moughan and Marlies Leenaars, 1992).
where, Tid = titanium in diet and Tii = titanium in ileal digesta.
Apparent digestibility data for N and AA were then converted to standardized digestibility values, using endogenous N and AA values determined from birds fed the N-free diet (Ravindran et al., 2017).
where, AID = apparent ileal digestibility of the AA, Basal EAA = basal endogenous AA loss and Ing., AA = concentration of the AA in the ingredient.
Apparent digestibility values were standardized using the following basal ileal endogenous flow values (g/kg DMI), determined by feeding N-free diet: N, 1.206; Met, 0.110; Cys, 0.149; Lys, 0.263; Thr, 0.468; Arg, 0.320; Ile, 0.285; Leu, 0.460; Val, 0.380; His, 0.098; Phe, 0.264; Gly, 0.317; Ser, 0.408; Pro, 0.375; Ala, 0.314; Asp, 0.573; Glu, 0.792; and Tyr, 0.261.
Statistical Analysis
Least square means and diet separation was determined using JMP v. 12.2.0 (SAS Institute, Cary, NC) by ANOVA and Tukey’s LSD (Haynes, 2013). To establish additivity of individual ingredient digestibility values relative to the measured value for the mixture, a linear model was applied (function lm()) in the base package of R version 3.2.2 running on Windows 7. The percent corn in the diet was coded as a numerical predictor, which resulted in a slope where the outcomes for the mixed corn/SBM diet would be a simple interpolation between the outcomes for corn and SBM. Because corn and SBM substitute each other, percent SBM was considered obsolete as a predictor. To test whether the outcome for the mixed corn/SBM diet deviates from this simple interpolation, a Boolean predictor was included, where SBM alone was coded as FALSE, corn alone as FALSE, and the mixed corn/SBM diet as TRUE. This quantifies the offset between the simple interpolation and the observed values in the mixed diet.
RESULTS
The proximate, mineral, and AA composition of the corn and SBM are presented in Table 2, and all values are in agreement with expectations, including the recovery of TiO2 in the feed samples. Phytase and protease activity recovered in the experimental diets is presented in Table 3 and is in line with expectations for these products.
The flow of EAA, presented in the methodology section above, in the terminal ileum of broilers that received the N-free diet are presented in Figure 1. The mean flow of EAA was 0.34 g/kg DMI. The flow of endogenous Asp, Glu, Thr, Ser, Leu, Pro, and Val were higher than this mean value, and other AA (notably Met and His where the endogenous flow was only 0.11 g/kg DMI) were lower.
The AID of AA in corn, SBM, and corn/SBM mixture, without or with phytase, protease, or a combination of phytase and protease is presented in Table 4. The AID of all AA other than Met, Cys, Leu, and Ala was higher (P < 0.01) in SBM or in the corn/SBM mixture compared with corn. There was no effect (P > 0.05) of phytase addition on the AID of AA. However, the effect of protease on the AID of Thr, Pro, and Cys was greater when offered in combination with phytase than without, resulting in a significant phytase by protease interaction for these AA. Addition of protease resulted in an increase (P < 0.01) in the AID of AA by 3.6%, which ranged from 2.1 and 2.3% for Arg and Met, respectively, to 6.0 and 6.4% for Thr and Cys, respectively.
Table 2. Proximate, mineral, and amino acid composition of corn and soybean meal (g/kg, as received)1.
The SID of AA in corn, SBM, and corn/SBM mixture, without or with phytase, protease, or a combination of phytase and protease is presented in Table 5. The SID of AA was not different (P > 0.05) between diets for Glu, Val, Ile, His, and Arg, whereas the SID of Cys, Met, Phe, Leu, Ala, and Pro was higher (P < 0.01) in corn compared with SBM. For N and the other AA, the SID was generally higher for SBM than for corn. Similar to the AID observations, the presence of phytase increased the effectiveness of protease on the SID of Thr, Pro, and Cys, resulting in a significant interaction for these AA. Protease addition resulted in an increase in the SID of all AA by an average of 3.4%, ranging from 2.0% for Arg to 6.1% for Cys.
The additivity of AID or SID values and the influence of exogenous enzyme addition on the same is presented in Figures 2 and 3, respectively. On an AID basis, the calculated digestibility of the corn/SBM mixture, (based on the determined AID of AA in the individual ingredients and their relative proportions in the mixture i.e., 65% corn and 35% SBM) underestimated the measured digestibility by 7.4%, ranging from 1.0% for Cys to 21.9% for Thr. However, when fed with a combination of phytase and protease, this underestimation was reduced (P < 0.05) to 6.7%. On a SID basis, the calculated digestibility of AA in corn and SBM underestimated the measured digestibility by only 2.9%, and the calculated and measured values were not significantly different. Addition of phytase and protease numerically reduced this underestimation to 2.5%, being particularly notable for Thr and Lys.
Table 3. Expected and measured enzyme activities in samples of the experimental diets.
DISCUSSION
The major difference between AID and SID values is the correction for basal endogenous flow, estimated by feeding a N-free diet. It is therefore of central importance that this correction is reliable and is indeed dietindependent and inevitable. Previous studies that have reported basal ileal endogenous flow include Siriwan et al. (1993), Ravindran et al. (2004), Adedokun et al. (2007), Golian et al. (2008), Soleimani et al. (2010), Kong and Adeola (2013a,b), and Cowieson et al. (2019), and these agree well with values presented herein. The flow of EAA in the present experiment (Figure 4) correlated positively (P < 0.001; r2 = 0.97) with the flow of EAA from broilers fed a N-free diet in previous works. Basal EAA flow contains high concentrations of Glu, Asp, Thr, Ser, Gly, and Leu and low concentrations of His, Met, Cys, Lys, and Tyr. It is perhaps unsurprising that this basal flow is moderately consistent because endogenous losses of AA originate from recalcitrant fragments of specific proteins such as sloughed enterocytes, mucin, digestive enzymes, and bacterial protein that have failed to autolytically succumb during normal N recovery processes (Moughan and Rutherfurd, 2012).
Figure 1. Flow of endogenous amino acids in the ileum of broilers fed a N-free diet (g/kg DM intake). Each bar represents the mean of 6 replicate cages and 6 birds per cage.
Table 4. Effect of exogenous enzymes on the apparent ileal amino acid digestibility (%) of corn, soybean meal (SBM), or a mixture of corn and SBM for growing broiler chickens (measured on day 28).
Table 5. Effect of exogenous enzymes on the standardized ileal amino acid digestibility (%) of corn, soybean meal (SBM) or a mixture of corn and SBM for growing broiler chickens (measured on day 28).
Figure 2. Deviation between calculated (based on measured values for corn and soybean meal (SBM) and their respective concentration in the mixture) and measured values for apparent (AID) ileal amino acid digestibility in broilers. Index 100 indicates that the digestibility of the mixture was predictable based on the digestibility of the individual raw materials and their inclusion concentration in the mixture. Values over 100 indicate that the digestibility of the individual raw materials was undervalued relative to their eventual contribution in the mixture. Values under 100 indicate that the digestibility of the individual raw materials was overvalued relative to their eventual contribution in the mixture. *represents a significant difference between calculated and measured digestibility values for the specific contrast within that treatment. Each bar represents the mean of 6 replicate cages and 6 birds per cage.
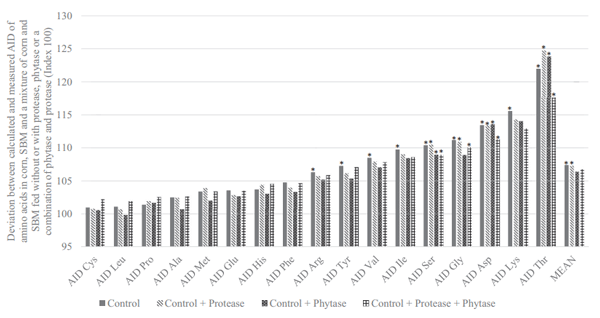
Importantly, diet-dependent endogenous loss may have a somewhat different AA profile depending on which endogenous proteins are involved in the physiological response to the incoming nutrient and antinutrient mix. Specifically, diets that stimulate an increase in intestinal mucin production may lead to greater concentrations of endogenous Cys in the digesta (from the proteolytically resistant domains of mucin), whereas if an increase in pepsin secretion is observed, significant changes may be noted in the intestinal flux of Asp (as pepsin contains a very high Asp concentration). Finally, while it may be possible to use standard values for basal endogenous loss of AA in broilers (based on literature evidence), these may not be applicable to other poultry species. Adedokun et al. (2018) reported almost 4 times higher total EAA (44,223 vs. 11,033 mg/kg DMI) and N (8,608 vs. 2,362 mg/kg DMI) losses in laying hens than broilers. Kong and Adeola (2013a) noted substantially higher basal endogenous loss in ducks compared with broilers (34,708 vs. 8,763 mg/kg DMI). It is possible that the reason these inevitable losses are higher in ducks than in chickens is associated with a lower investment in breeding programs to improve the efficiency of nitrogen cycling in the gut. In broilers, these efficiencies have been captured through significant changes in the efficiency of N metabolism over many decades as demonstrated by Saunderson and Leslie (1988) using N-methyl histidine as a biomarker of protein catabolism. It is therefore recommended that basal endogenous loss be “re-calibrated” more regularly for nonbroiler poultry species. Nevertheless, the basal flow of EAA noted in the present work is in line with values reported previously, and this sets context for interpretation of the reported treatment responses.
Figure 3. Deviation between calculated (based on measured values for corn and soybean meal (SBM) and their respective concentration in the mixture) and measured values for standardized (SID) ileal amino acid digestibility in broilers. Index 100 indicates that the digestibility of the mixture was predictable based on the digestibility of the individual raw materials and their inclusion concentration in the mixture. Values over 100 indicate that the digestibility of the individual raw materials was undervalued relative to their eventual contribution in the mixture. Values under 100 indicate that the digestibility of the individual raw materials was overvalued relative to their eventual contribution in the mixture. Each bar represents the mean of 6 replicate cages and 6 birds per cage.
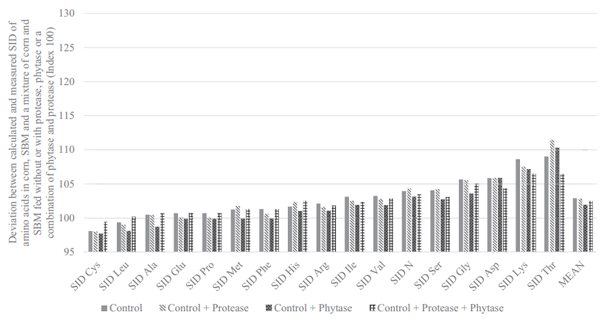
Figure 4. Correlation between the basal flow of endogenous amino acids from birds fed a N-free diet in the present experiment (x axis) and the same based on a literature review (Siriwan et al., 1993; Ravindran et al., 2004; Adedokun et al. 2007; Golian et al., 2008; Soleimani et al., 2010; Kong and Adeola, 2013a,b; Cowieson et al., 2019).
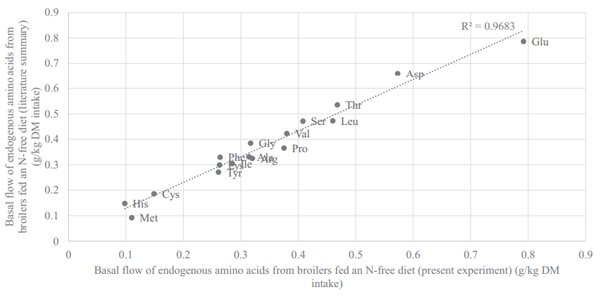
The effect of diet (corn, SBM, or a corn/SBM) and enzyme (unsupplemented, phytase, or protease) on the AID and SID of AA are presented in Tables 4 and 5, respectively. The mean AID of AA in corn (77.0%), SBM (84.6%), and the mixture (88.2%) are broadly in agreement with previous studies. For example, Cowieson et al. (2019) noted AID of AA in corn, SBM, or a 65/35 mixture of corn and SBM of 72.3, 78.1, and 78.9%, respectively, and Kong and Adeola (2013a) noted values of 79.4, 81.4, and 84.0%, respectively, for the same ingredients. Some variance from experiment to experiment is expected because of the influence of the individual ingredient sources used (both corn and SBM are known to vary in digestibility of macronutrients; Leeson et al., 1993; Douglas et al., 2000; Ravindran et al., 2005), minor changes in methodology, and in the capacity of the birds to extract nutrients from the feed (Hughes and Choct, 1997). Importantly, in previous work (Cowieson et al., 2019), the corn/SBM mixture returned a consistently higher AID of AA than the corn or SBM alone. This observation was confirmed herein, especially for Thr, Lys, Asp, Gly, and Ser, where underestimation of the true value of individual ingredients was more than 10% (Figure 2), further demonstrating the limitation of arithmetic assumptions about the nutritional value of individual ingredients in complete diets. On a SID basis, the values found in the present work are also in agreement with previous reports (Kong and Adeola, 2013a; Cowieson et al., 2019), and crucially, the underestimation of the value of the single ingredients was remedied by adjustment of AID to SID values. Cowieson et al. (2019) noted that the underestimation of the AA value of corn and SBM was around 7% when an AID system was employed compared with around 2% for a SID system. This is similar to the results presented herein (Figure 3), where no significant differences were noted between measured and calculated values for AA digestibility when a SID system was used, and the underestimation associated with the AID system (7.4%; Figure 2) was substantially corrected (2.9%; Figure 3).
The effect of both phytase and protease on the digestibility of AA in various ingredients and diets for broilers (and other species) is well documented and has been the subject of recent reviews (Cowieson and Roos, 2014; Cowieson et al., 2017a,b). Suffice to say that the increase in AID and SID of AA associated with exogenous protease addition (around 4%) is in line with previous observations and is interpreted to be associated with an increase in the solubility and digestibility of dietary AA and a reduction in specific EAA loss (Cowieson and Roos, 2016). Similarly, the effect of phytase on the AID and SID of AA herein is also within the range previously reported in broilers (Cowieson et al., 2017a), and while this failed to reach statistical significance, it is likely that the consistent numerical increase in AA digestibility with phytase addition is associated with a reduction in the antinutritional effects of phytic acid. It is possible that the relatively modest effect of phytase on AA digestibility in corn in the present work compared with previous reports (Cowieson et al., 2017a) is associated with the low measured phytate–phosphorus concentration (1.8 g/kg; Table 2) compared with complete diets or even alternative batches of corn (1.6–2.6 g/kg; Eeckhout and De Paepe, 1994). The significant interaction between phytase and protease for the AID and SID of Thr, Pro, and Cys is intriguing and not easy to explain. In the absence of phytase, the addition of protease increased the SID of Thr, Pro, and Cys by 1.6, 1.6, and 3.6%, respectively. Phytase alone had no effect on the SID of these AA. However, when protease was added on top of phytase, an increase in the SID of Thr (1.6– 8.0%), Pro (1.6–4.5%), and Cys (3.6–8.8%) was observed. This statistically confirmed synergy between phytase and protease is interesting and may be associated with cooperation between these enzymes for access to substrate, for example protease improving solubility of phytate, or perhaps through physiological effects involving myo-inositol, sodium partitioning, or more generally, AA absorption and peptide transport (Cowieson et al., 2017c; Moss et al., 2018). Nevertheless, the pattern of response to both protease and phytase on AA digestibility is consistent with previous reports (Ravindran et al., 1999; Cowieson and Roos, 2014; Cowieson et al., 2017a; Siegert et al., 2019) and reinforces the value of these additives in broiler chicken nutrition.
As far as the authors are aware, the present work is the first to explore the effect of exogenous enzymes on the AID and SID of AA for individual ingredients and a mixture of the same ingredients and was an important objective of the work. Crucially, if the effect of an enzyme on an individual ingredient is not translatable to a complete diet, it calls into question many of the enzyme application systems used in feed formulation today. Matrices for complete diets (applied “artificially” to the enzyme in formulation) would, perhaps, be immune from criticism on this axis. However, this is only if they have been derived by feeding complete diets, without or with the enzyme of interest and that this was replicated adequately to bring confidence to the derivation of the final matrix recommendation. Enzyme values that are derived using single ingredient evaluation studies may be subject to criticism if these cannot be translated into complete diets because of lack of arithmetic coherence. However, in the present work, the effect of both phytase and protease on the individual ingredients was proportionately predictable based on the effect in the mixed diet. Furthermore, even when the AID system was considered (Figure 2), the use of the combination of phytase and protease improved the additivity of AA contributions from corn and SBM overall and also for specific AA such as Lys, Ile, Val, Tyr, and Arg. This is a novel and important feature of exogenous enzymes and implies that enzyme use may give more formulation flexibility to nutritionists by enhancing the arithmetic additivity of AID (and indeed SID) systems. The reason for this observation is not clear but may be related to effects of these enzymes on basal or specific EAA flow. The effect of enzymes on specific endogenous loss is an area for future study so that matrices for enzymes can be further enhanced to promote compatibility with various formulation approaches.
CONCLUSIONS
It can be concluded that the SID AA system for feed ingredient appraisal results in more precise and predictable outcomes for mixed diets than is the case for AID AA approaches and should be used wherever possible. Furthermore, exogenous protease is an effective tool to promote the digestibility of AA in broilers and may do so to a greater extent than is the case for phytase. The synergistic effects of phytase and protease on the SID of Thr, Cys, and Pro warrants further attention, especially considering endogenous protein flow, peptide transport, and sodium partitioning. Crucially, this is the first report that has demonstrated additivity of AA digestibility effects of protease and phytase under AID and SID systems and gives confidence in enzyme matrices that have been derived in single ingredients. The use of exogenous phytase and protease may also improve the additivity of AID and SID AA values for use in complex diets. Thus, exogenous enzymes may not only promote nutrient utilization from feed ingredients but increase the flexibility in formulation approaches for nutritionists. These effects require further elucidation and evaluation in more complex ingredient mixtures.
ACKNOWLEDGMENTS
This work was financially supported by DSM Nutritional Products.
Conflict of Interest Statement: The authors did not provide any conflict of interest statement.