I. INTRODUCTION
That a mounting array of synthetic amino acids will be incorporated into broiler diets to increasing extents would appear to be an entirely reasonable prediction. The caveat is that predictions are fraught with difficulty; particularly when they concern the future. However, nearly 50 years ago, Beames et al. (1968) made the following prognostication: “It is probable that the price of synthetic amino acids will continue to fall and that they will be used increasingly as replacements for conventional protein supplements”.
The inclusion of free or synthetic amino acids in broiler diets, methionine, lysine and threonine, has been routinely practiced for decades. Now additional essential amino acids including arginine, isoleucine, tryptophan and valine are commercially available. The likelihood is that the increasing global protein demand for both human food and animal feed will continue to drive protein prices higher, which has been the case for soybean meal. Against this outlook, the prices for synthetic amino acids are relatively static or even declining. In one feeding study (Selle et al., 2007), typical wheat-based broiler diets were supplemented with 2.06 g/kg methionine, 2.95 g/kg lysine hydrochloride and 0.87 g/kg threonine. This represented 40, 20 and 11%, respectively, of the specific amino acid concentrations in the diet and 2.5% of the dietary protein content. While the proportions for specific amino acids are substantial; the likelihood is that these proportions will increase and embrace a broader range of amino acids in response to escalating prices for protein meals.
This review is a speculative consideration of the implications of the probable increase in free amino acid inclusions in broiler diets in the interactive context of starch and protein digestive dynamics.
II. BACKGROUND
Golberg and Guggenheim (1962) compared the digestion of amino acids and their appearance in portal circulation in rats offered casein or soy flour as protein sources. Both the small intestinal digestion and the entry into the portal circulation of lysine from casein were more rapid than from soy flour. Moreover, the data suggests that a greater proportion of lysine entered the portal circulation from casein, the more rapidly digested protein source. Thus differences in digestive dynamics of protein-bound amino acids exist between feedstuffs but differences between free and protein-bound amino acids will be of an even greater magnitude. Free amino acids do not undergo digestion and are directly available for absorption in the upper small intestine and appear in the portal circulation more rapidly than protein-bound amino acids (Wu, 2009). Thus the digestive dynamics of free amino acids, that is the rate, quantity and site of absorption is very different to that of protein-bound amino acids. This was demonstrated by Ted Batterham (Batterham, 1974; Batterham and O'Neill, 1978) in respect of lysine monohydrochloride in grower pigs. In a review of these studies, Batterham and Murison (1981) concluded that the utilisation of free lysine with once daily feeding was 0.53 for weight gain and 0.56 for feed conversion efficiency on the basis of carcass weight, relative to frequent feeding. Thus the differential absorption rates and the asynchronous availability of free and protein-bound lysine at sites of protein accretion was pivotal. Indeed, Kondos and Adriaans (1982) claimed that a dry-extruded, ‘slow release’ lysine outperformed lysine HCl in grower pigs fed on a twice-daily basis by 9.33% in average daily gain (820 versus 750 g/day), 8.12% in feed conversion efficiency (2.49 versus 2.71) in association with a 16.5% reduction in N urinary losses. While similar outcomes had been previously reported in rats (Rolls et al., 1972), Batterham’s proposition that the digestive dynamics of free and protein-bound amino acids were asynchronous did not meet with unanimous acceptance at their time of publication. Nevertheless, it was subsequently demonstrated that the utilisation of free amino acids by broiler breeders fed once- or twicedaily was inferior on the same basis (Nonis and Gous, 2006).
An open question is how efficiently can broiler chicks utilise free amino acids? Broilers are offered diets on an ad libitum basis and their feed intake under a ‘24-hours-ons’ lighting regime is ostensibly continuous. Nevertheless, Pinchasov et al. (1990) reported that 7-21 day weight gains and feed conversions were compromised by offering chicks maizebased diets with increasing inclusions of free amino acids on an unrestricted basis but the lighting regime was not specified.
The definition of digestive dynamics in this review includes the digestion of nutrients in the gut lumen, absorption of end-products into the gut mucosa, and their post-enteral availability. This process involves both static and kinetic components and static ileal digestibility coefficients are used to calculate the kinetic parameters. That is the rate, quantity and site of absorption of glucose and amino acids along the small intestine. The rate of digestion may be deduced by fitting apparent digestibility coefficients and mean retention times in intestinal segments into an exponential mathematical model. The quantity of a nutrient absorbed at the terminal ileum (g/bird/day) or other intestinal segments is a simple function of feed intakes, dietary concentrations and digestibility coefficients. The site of absorption may be defined as one of four small intestinal segments (proximal and distal jejunum, proximal and distal ileum) and the amounts of starch or protein that apparently disappear or are absorbed as glucose and amino acids may be deduced.
More than sixty years ago, Geiger (1950) opined that the relative timing of amino acid and carbohydrate uptakes can markedly affect protein synthesis and the efficiency of feed utilisation. Nevertheless, the complex impacts of starch and protein digestive dynamics in broilers still require further elucidation and the importance of digestive dynamics in relation to chicken-meat production merits greater recognition (Liu and Selle, 2014; Liu et al., 2014a). Instructively, Rerat (1993) made the following (condensed) statements:
“The food intake of single-stomached animals is discontinuous and varies in amount and composition. These quantitative and qualitative variations in food intake pose a number of questions. Do they affect the digestive processes which are related to food transit and enzyme hydrolysis, especially the extent of nutrient absorption and its kinetics? These questions cannot be answered merely by measuring digestibility. Digestibility is used to evaluate the disappearance of ingested nutrients either in the whole digestive tract or in its proximal part (ileal digestibility). Whichever technique is used, these digestibility measures are global and static and do not account for the different absorption rates which vary for different nutrients. Study of kinetics associated with differences in the relative absorption rate of the various nutrients is necessary for a fuller understanding of variations in the nutritive value of feeds.”
The validity of the Rerat (1993) summation of kinetics or dynamics is evidenced in one study (Selle et al., 2012; Liu et al., 2013a) completed by the Poultry Research Foundation. This involved broiler diets based on red, white and yellow sorghums and mash, reground pellet and intact pellet feed forms in a 3x3 factorial array of dietary treatments. As shown in Table 1, ileal digestibility coefficients of starch and nitrogen were not significantly correlated to key performance parameters. However, there were predominantly significant correlations between starch and nitrogen digestion rates and predicted glycaemic indices (or glucose absorption) with weight gain, FCR and N retention.
Table 1 - Pearson correlation coefficients (probability values in parentheses) between static and dynamic digestibility parameters and performance of broiler chicks from 7 to 27 days post-hatch (adapted from Selle et al., 2012; Liu et al., 2013a).
Further confirmation of the importance of digestive dynamics is illustrated in Figure 2. In a recent study (Truong et al., 2015) there was a negative linear relationship between starch:protein disappearance rate ratios and 40-day weight gains. The negatively correlation has an R2 value of 0.587; thus 59% of the variation in weight gain may be attributed to the disappearance rate ratio and, importantly, weight gains will be enhanced following an increase in the disappearance rate of protein (g/bird/day) relative to starch from the proximal ileum.
Figure 1 - Linear relationship (r = -0.766; P = 0.003) between starch:protein disappearance rate ratios in proximal ileum and 40-day weight gains in broilers offered maize-based diets without and with 500 FTU/kg phytase (Truong et al., 2015).
III. INTERMITTENT FEED INTAKES
Broiler chickens are fed ad libitum but this unrestricted access to feed does not equate to continuous feeding and feed intakes of broilers may be best described as intermittent and are also subject to lighting regimen. Broilers exhibit diurnal feeding patterns and consume most of their ration either at the start or end of the day (Savory, 1980). Moreover, Jensen et al. (1962) found that chicks offered pelleted diets under a ‘12-hours-on’ lighting regime spent only 4.7% of illuminated time in eating and Fujita (1974) reported that chicks spent 10.8% of time eating under a ‘14-hours-on’ lighting regime. Based on five of our studies, the average retention time in the jejunum and ileum was 2.98 hours. Coupled with the data of Denbow (2000) for retention times in the crop, proventriculus, gizzard, and duodenum, digesta reaches the terminal ileum in broilers in approximately 4.25 hours. Thus, despite essentially unrestricted access to feed in commercial production systems, intermittent feed consumption patterns still provide scope for asynchronies in digestion and absorption of nutrients in the small intestine to influence broiler performance. This logical interpretation is supported by both the data in Table 1 and a review of starch and protein digestive dynamics (Liu and Selle, 2014) in which the impacts of digestive dynamics in broilers are evident under standard lighting regimen. Starch and protein digestion occurs mainly in the jejunum through which digesta will pass in approximately 3 hours; therefore, as lighting regimen influence feed consumption patterns and extended periods without illumination should intensify imbalances in digestive dynamics.
IV. AMINO ACID CATABOLISM
The efficient anabolic incorporation of amino acids into skeletal protein is the desired outcome for chicken-meat production. However, a substantial proportion of amino acids undergo inevitable and preferential catabolism which represents a shortfall of amino acids for protein deposition. Estimates of lysine catabolism range from 15 to 30% in pigs and 20 to 24% in broiler chickens (Moughan, 2014). From a growth simulation model for 50 kg pigs, Moughan (2014) predicted that the inevitable catabolism of lysine accounted for 24-44% of losses which exceeded losses from undigested (25-32%) and endogenous flows (12-18%) of lysine. Sites of amino acid catabolism are not limited to intestinal mucosal cells but surprising quantities of amino acids are catabolised in enterocytes for the provision of energy to meet the copious requirements of the gut (Stoll et al., 1998; Wu, 1998). Initially Windmueller and Spaeth (1975) reported that the rat intestine utilised nearly all absorbed dietary glutamic acid and circulating glutamine but 60% of glutamate/glutamine was metabolised to CO2 and this catabolism made ‘an important contribution to the energy requirement of the gut’. Subsequently, Stoll et al. (1999) reported that glucose, glutamate and glutamine met 75% of the energy requirement of portal drained viscera in pigs and that dietary glutamic acid was fundamental in this respect. Poultry studies are limited but Watford et al. (1979) found that both glutamate/glutamine and glucose were oxidised in enterocytes but glucose generated 27% more oxygen in chicken enterocytes than glutamate/glutamine. Wu et al. (2005) even suggested that antimicrobial agents depress catabolism of dietary amino acids in enterocytes by reducing the intestinal mass and that this may be one mechanism for their growth promoting effects. The implication is that catabolism of amino acids in the gut mucosa is manipulable and its reduction would trigger the entry of more amino acids into the systemic circulation to become available for protein accretion with a corresponding increase in the more efficient utilisation of glucose for energy provision to the gut.
V. SLOWLY DIGESTIBLE STARCH
Data has been generated (Weurding, 2002; Weurding et al., 2003a,b) to indictae that the provision of some slowly digestible starch, or starch digested in the lower small intestine, in broiler diets enhances feed conversion efficiency. More recently it was demonstrated that slowly or gradually digestible starch enhanced energy utilisation (AME, AMEn) in addition to FCR in sorghum-based broiler diets (Liu et al., 2014b; Selle et al. 2013b, 2014). Gradually digestible starch was defined as starch digested in the distal jejunum, proximal and distal ileum as opposed to the proximal jejunum. Graded inclusions of the reducing agent sodium metabisulphite generated more gradually digestible starch which appeared responsible for the improvements in energy utilisation and FCR. A more continuous absorption of glucose from the gut pursuant to the provision of slowly digestible starch could beneficially modify insulin-induced responses. However; any interpretation of possible glucose/insulin responses is complicated by the differences between avian and mammalian species in glucose and insulin metabolism, although these differences may be overstated (Simon, 1989; Simon et al., 2011). An alternative hypothesis is that slowly or gradually digestible starch may enhance FCR in broilers via a protein-sparing effect (Weurding et al., 2003b). Enting et al. (2005) included amino acids as glutamine and casein in broiler diets based on unprocessed or hydrothermally-treated maize and peas as hydrothermal processing increases starch digestion rates and there were significant interactions between amino acid inclusions and starch digestion rates. In slowly digestible starch diets amino acids marginally improved FCR (0.60%) but, in rapidly digestible starch diets, amino acids improved FCR by 4.66% (1.638 versus 1.718). These researchers suggested that slowly digestible starch might “prevent the use of amino acids as an energy source for the gut wall”, thereby increasing their availability for protein accretion.
The gastrointestinal tract consumes in the order of 20% of incoming dietary energy for digestive and absorptive processes (Cant et al., 1996) and much of this energy is derived from catabolism of amino acids rather than glucose (Fuller and Reeds, 1998). Nevertheless, Fleming et al. (1997) found starch/glucose and glutamic acid/glutamine were approximately equally important energy substrates for small intestinal mucosal cells in the rat. Moreover, net ATP production from glucose was greater than glutamine by a two-fold factor. Similarly Posho et al. (1994) reported that, in comparison to glutamine alone, the provision of both glucose and glutamine to porcine enterocytes reduced ATP production from glutamine. This suggests that enterocytes can derive energy from glucose provided it is available. It follows that glucose from rapidly digested starch sources would be depleted in the upper small intestine, effectively forcing mucosal cells in the lower small intestine to catabolise amino acids for energy provision. Thus slowly digested starch sources would provide more glucose to the lower small intestine thereby sparing protein from oxidation and energy generation from glucose would be substantially more efficient than from amino acids.
A pivotal study was completed by van der Meulen et al. (2007) in pigs. Less rapidly digested native pea starch significantly increased the net portal flux of essential amino acids by 22.9% in comparison to more rapidly digestible maize starch. More specifically, slowly digestible starch increased portal fluxes of tryptophan and lysine by 42.3 and 28.6% in absolute terms. Also, slowly digestible starch increased proportions of tryptophan and lysine in portal circulation by 49.3 and 32.5%, respectively Relatively, as a proportion of ileally digested amino acids. Some of the van der Meulen et al. (2007) findings are shown in Figure 2 and indicate that amino acid catabolism for energy provision to the gut is an important source of amino acid losses that can be averted by slowly digestible starch.
Figure 2 - Percentage increase in net portal flux (mmol) of essential amino acids following the provision of a more slowly digestible starch source (pea versus maize starch) to pigs (adapted from van der Meulen et al., 1997).
VI. FREE AMINO ACIDS
Free amino acids do not require digestion and it follows that they will be rapidly and almost completely and rapidly absorbed in the upper small intestine to far greater extents than protein-bound amino acids. Schwartz et al. (1959) compared free and protein-bound lysine in chicks and at the highest level of supplementation, free lysine HCl supported nearly 20% greater weight gains than bound lysine in dehulled soybean meal. The more rapid digestion rates of free amino acids is illustrated in Table 2 where sorghum-based broiler diet were supplemented with methionine, lysine and threonine as free amino acids (Liu et al., 2013b). The average digestion rate for nine essential amino acids was 2.96 kamino acidx10-2min-1 with the branched chain amino acids being noticeably slower. In contrast, methionine and lysine had a remarkably higher mean digestion rate of 4.83 kamino acidx10-2min-1 because of their dietary presence in both free and bound forms. Thus free amino acids accelerate ‘protein’ digestion rates relative to starch, which could provide a better post-enteral balance of glucose and amino acids for protein deposition.
While speculative, but perhaps importantly, free amino acids may be less susceptible to catabolism in the gut mucosa because of their proximal absorption in segments of the small intestine where more glucose is available as an alternative energy substrate. On the basis of the van der Meulen et al. (2007) data, this would apply especially to tryptophan and lysine, less so to threonine, valine and isoleucine and least to methionine and arginine. If, in fact, free tryptophan and lysine are less prone to catabolism in the gut mucosa than their proteinbound counterparts, then the implications are quite profound.
Table 2 - Digestion rate (kamino acid x 10-2min-1) in descending order of amino acids in a sorghum-based broiler diet supplemented with 3.37 g/kg methionine, 3.74 g/kg lysine and 1.29 g/kg threonine as free amino acids (adapted from Liu et al., 2013b).
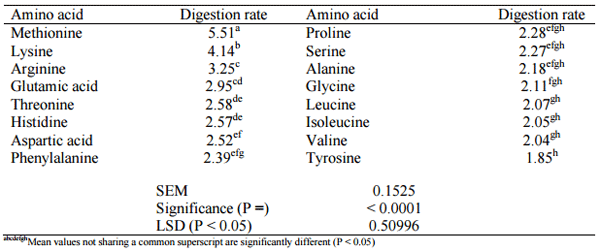
Fang et al. (2010) compared the first-pass intestinal metabolism and post-enteral availability of DL-methionine (DL-MET) with DL-2-hydroxy-4-methylthiobutyrate (DLHMTB) in pigs; however, a diet with only protein-bound methionine was not assessed. The fractional net portal balance of methionine in diets containing DL-HMTB (~80%) was significantly higher than DL-MET (~60%) by approximately one-third over a six hour postprandial period. These results suggest that free amino acids can be chemically modified in order to decrease their metabolism in the gut mucosa and increase their entry into the systemic circulation. Also, it appears that the first-pass intestinal usage of free DL-HMTB methionine was modest which lends some support to the suggestion that the propensity of free amino acids to undergo cartabolism in the gut mucosa is less than their protein-bound counterparts.
Alternatively, dietary inclusions of a given free amino acid may disrupt the digestive dynamics of that particular amino acid and/or the balance of amino acids to the detriment of protein deposition. Free lysine has been shown to impact negatively on the utilisation of [14C ]phenylalanine in pigs offered diets on a restricted basis (Batterham and Bayley, 1989).
Daenzer et al. (2001) offered diets to rats containing protein as either casein (150 g/kg) or an equivalent mix of free amino acids. Predictably, the absoprtion of free amino acids was generally more rapid than from casein. However, weight gains were lower and urinary N losses higher in rats offered free amino acids, promting the researchers to conclude that whole-body net protein synthesis was better supported by protein-bound amino acids. Importantly, the meals were offered twice-daily to rats trained to consume their ration in less than 30 minutes. Wheat starch was the primary dietary energy source and it appears that a better dynamic balance was struck between starch and casein than with free amino acids but this was observed under restricted feeding regimen.
In conclusion, in general terms, increasing usage of free amino acids will benefit chicken-meat production because they will attenuate the impact of escalating protein meal prices which is pivotal. Free amino acids will facilitate the fine-tuning of least-cost ration formulations in respect of key digestible amino acid ratios, including lysine, arginine and branched-chain amino acids. In addition, free amino acids should increase the overall rate of “protein” digestion relative to that of starch which may be of benefit. More specifically, a potential advantage is that the propensity of free amino acids to undergo catabolism in the gut mucosa may be less than protein-bound amino acids because of the more proximal absorption of free amino acids in the small intestine. This would result in the inherently more efficient derivation of energy from glucose to drive digestive processes. Alternatively, a potential disadvantage is that free amino acids may lead to an unbalanced provision of free and bound amino acids at sites of protein deposition which would compromise the utilisation of both the specific and the balance of amino acids. However, this impact would appear to be largely dependent on consumption patterns under ostensibly ad lib feeding regimes and whether or not broiler chickens are continuous or discontinuous feeders. Feeding patterns may be modified by more extended patterns of illumination and the practice of whole grain feeding may encourage more continuous feed intakes than intact pellets. Certainly, investigations into these possible positive and negative outcomes are justified as the likelihood is greater advantage can be taken from the use of free amino acids following the development of a better appreciation of their role in starch and protein digestive dynamics. Nevertheless, in overall terms, the roll-out of more free amino acids should be a positive development for the chicken-meat industry.
REFERENCES
Batterham ES (1974) British Journal of Nutrition 31: 237-242.
Batterham ES & O'Neill GH (1978) British Journal of Nutrition 39: 265-270.
Batterham ES & Murison RD (1981) British Journal of Nutrition 39: 265-270.
Batterham ES & Bayley HS (1989) British Journal of Nutrition 62: 647-655.
Beames RM, Daniels LJ & Sewell JO (1968) Proceedings of the Australian Society of Animal Production 7: 391-396.
Cant JP, McBride BW & Croom WJ (1996) Journal of Animal Science 74: 2541-2553.
Daenzer M, Petzke KJ, Bequette BJ & Methes CG (2001) Journal of Nutrition 131: 1965- 1972.
Denbow DM (2000) Gastrointestinal Anatomy and Physiology. In: Sturkie's Avian Physiology, Fifth Edition (Whittow GC) Academic Press, Waltham, MA, USA. pp. 299- 326.
Enting H, Pos J, Weurding RE & Veldman A (2005) Proceedings of Australian Poultry Science Symposium 17: 17-20.
Fang Z, Huang F, Luo J, Wei H, Ma L, Jiang S & Peng J (2010) British Journal of Nutrition 103: 643-651.
Fleming SE, Zambell KL & Fitch MD (1997) American Journal of Physiology (Gastrointestinal and Liver Physiology) 273: G968-G978.
Fujita H (1974) Japanese Poultry Science 11: 210-216.
Fuller MF & Reeds PJ (1998) Annual Review of Nutrition 18: 385-411.
Geiger E (1950) Science 111: 594-599.
Goldberg A & Guggenheim K (1962) Biochemistry Journal 83: 129-135.
Jensen, LS, McGinnis J, Reddy CV & Merrill LH (1962) Poultry Science 41: 1414-1419.
Kondos AC & Adriaans RM (1982) Proceedings of the Australian Society of Animal Production 14: 561-564.
Liu SY, Selle PH & Cowieson AJ (2013) Animal Production Science 53: 1033-1040.
Liu SY, Selle PH, Court SG & Cowieson AJ (2013) Animal Feed Science and Technology 183: 175-183.
Liu SY & Selle PH (2014) World’s Poultry Science Journal (submitted for publication).
Liu SY, Cowieson AJ & Selle PH (2014) Proceedings of the XIVth European Poultry Conference. European Federation, World’s Poultry Science Association. Stavanger, Norway.
Liu SY, Selle PH, Khoddami A, Roberts TH & Cowieson AJ (2014) Animal Feed Science and Technology 190: 68-78.
Moughan PJ (2014) Proceedings of the Fifth International Broiler Nutritionists’ Conference, Queenstown, New Zealand, April 2014. Poultry Industry of New Zealand, Auckland. Massey University, Palmerston. pp. 1-20.
Nonis MK & Gous RM (2006) South African Journal of Animal Science 36: 126-134.
Pinchasov Y, Mendonca CX & Jensen SL (1990) Poultry Science 69: 1950-1955.
Posho L, Darcy-Vrillon B, Blachier F & Duee P-H (1994) Journal of Nutritional Biochemistry 5: 284-290.
Rerat AA (1993) Proceedings of the Nutrition Society 52: 335-344.
Rolls BA, Porter JWG & Westgarth DR (1972) British Journal of Nutrition 28: 283-293.
Savory CJ (1980) Applied Animal Ethology 6: 71-82.
Schwartz HG, Taylor MW & Fisher H (1959) Poultry Science 38: 316-318.
Selle PH, Gill RJ & Scott TA (2007) Proceedings of the Australian Poultry Science Symposium 19: 182-185.
Selle PH, Liu SY, Cai J & Cowieson AJ (2012) Animal Production Science 52: 842-852.
Selle PH, Liu SY, Cai J & Cowieson AJ (2013) Animal Production Science 53: 378-387.
Selle PH, Liu SY, Cai J, Caldwell RA & Cowieson AJ (2013) Animal Feed Science and Technology 186: 81-90.
Selle PH, Liu SY, Cai J, Caldwell RA & Cowieson AJ (2014) Animal Feed Science and Technology 190: 59-67.
Simon J (1989) Critical Reviews in Poultry Nutrition 2: 121-148.
Simon J, Rideau N, Taouis M & Dupont J (2011) General and Comparative Endocrinology 171: 267-268.
Stoll B, Henry J, Reeds PJ, Yu H, Jahoor F & Burrin DG (1998) Journal of Nutrition 128: 606-614.
Stoll B, Burrin DG, Henry J, Yu H, Jahoor F & Reeds PJ (1999) American Journal of Physiology (Endocrinology and Metabolism) 40: E168-E175.
Truong HH, Bold R, Liu SY & Selle PH (2015) Animal Feed Science and Technology (submitted for publication).
van der Meulen J, Bakker JGM, Smits B & de Visser H (1997) British Journal of Nutrition 78: 533-544. Watford M, Lund P & Krebs KA (1979) Biochemistry Journal 178: 589-596.
Windmueller HG & Spaeth AE (1975) Archives of Biochemistry and Biophysics 171: 662- 672.
Weurding RE (2002) PhD Thesis, Wageningen Universiteit. Wageningen, The Netherlands.
Weurding RE, Enting H & Verstegen MWA (2003) Poultry Science 82: 279-284.
Weurding RE, Enting H & Verstegen MWA (2003) Animal Feed Science and Technology 110: 175-184.
Wu GY (1998) Journal of Nutrition 128: 249-252.
Wu GY (2009) Amino Acids 37: 1-17.