INTRODUCTION
Studies from humans and rats have showed the intestinal epithelium represents the largest area of exposure of the body to external pathogens and toxins (Abreu, 2010; Slack et al., 2012). The secretory immune system, including secretory IgA (sIgA) and the mucus, serves as the first line of defense to limit epithelial contact with pathogenic microorganisms and other potentially dangerous antigens (Abreu, 2010; Slack et al., 2012; Zhang et al., 2015). Immunoglobulin A (IgA) is the predominant immunoglobulin in the intestine, where it’s found in a polymeric form vs. its monomeric form in serum (Slack et al., 2012). Polymeric IgA (pIgA) is locally produced by plasma cells in the lamina propria and must bind to its polymeric immunoglobulin receptor (pIgR) expressed basolaterally on epithelial cells for entry into the intestinal lumen. The pIgA-bound pIgR is then endocytosed and transcytosed across the epithelium to the apical surface, where the extracellular portion of pIgR is cleaved to release a secretory component (SC), a part of the sIgA complex (Kaetzel, 2005; Johansen and Kaetzel, 2011). In addition to its protective role of sIgA from cleavage by proteases, SC anchors sIgA within the mucus to facilitate the immune exclusion of intestinal antigens by entrapping them in mucus and blocking their access to epithelial cells (Everett et al., 2004; Johansen and Kaetzel, 2011; Slack et al., 2012). Therefore, nutrients contributing to the formation of the sIgA mediated secretory immune system may play a role in defense against intestinal pathogens and luminal antigens.
Threonine (Thr) is a dietary essential amino acid that has been shown to have a particularly high retention rate in the intestine vs. other essential amino acids in pigs, which suggests its importance in gut functions (Stoll, 2006). One of the primary fates of Thr extracted by the gut is the synthesis of mucins, where Thr represents as much as 28 to 35% of the total constituent amino acids (Sandberg et al., 2007; Nichols and Bertolo, 2008). High sensitivity of mucin production to luminal
Thr concentrations has been demonstrated in rats (Faure et al., 2005), pigs (Law et al., 2007), chickens (Horn et al., 2009), and ducks (Horn et al., 2009; Zhang et al., 2014). In addition to mucins, Thr accounts for 7 to 11% of the total amino acids in IgA (Sandberg et al., 2007). The requirement of Thr during lymphocyte proliferation and immunoglobulin production has been demonstrated in vitro (Taudou et al., 1984; Duval et al., 1991). In animal studies, Thr is known to affect the production of intestinal IgA in laying hens (Azzam et al., 2011) and serum IgY in ducks (Zhang et al., 2014). Therefore, Thr is a critical nutrient that participates in the production of mucins and IgA to maintain the intestinal secretory immune system.
During inflammation, Thr utilization for synthesis of intestinal proteins, such as mucins and IgA, had been increased in rats (Faure et al., 2007) and mini-pigs (R´emond et al., 2009). Therefore, it is hypothesized that limited luminal Thr availability may impair the gut secretory immune system upon exposure to inflammatory stimuli. Lipopolysaccharide (LPS), the major cell wall component of Gram-negative bacteria, serves as a very strong stimulator of inflammatory and innate immunity (Mani et al., 2012). The LPS induced inflammation and activation of the secretory immune system in a chicken ileal ex vivo model has been validated (Zhang et al., 2017). Therefore, the purpose of this study was to determine the effect of Thr on the secretory immune system by using the validated explant model and to elucidate the possible pathways involved in mucin and pIgR expression in chicken epithelial cells.
MATERIALS AND METHODS
Bird Use
All procedures and protocols were approved by the Purdue University Animal Care and Use Committee. Day-old male broiler chicks (Ross 708) were obtained from a commercial hatchery and housed in electrically heated battery cages (Alternative Design Manufacturing and Supply Inc., Siloam Springs, AR) until 21 d of age. Battery cage temperature was maintained at 35 ± 1◦C for the first wk and gradually decreased to 27◦C in the third week. The lighting schedule was 22L:2D throughout the experiment. Feed and water were provided ad libitum.
Ileal Explant Culture
Culture of ileal explants followed the procedure described in our previous study (Zhang et al., 2017). Briefly, the ileum segment from each bird was collected and washed in pre-warmed (37◦C) RPMI 1640 medium (Life Technologies, Carlsbad, CA), added with 100 U/mL penicillin, 100 μg/mL streptomycin, and 0.25 μg/mL of Fungizone R (Life Technologies, Carlsbad, CA). The ileum was dissected into 5 ×5 mm-piece explants with a sterile scalpel blade and randomly distributed into the 24-well culture plates (Sigma Aldrich, St. Louis, MO). In each well of the culture plates, one explant was incubated villi up on 1.5 cm2 biopsy foam pads. In standard culture conditions, the chicken ileal explants were incubated in 1.5 mL self-made culture medium (pre-warmed in 37◦C and gassed with 5% CO2). All these operations were conducted in less than one h after the bird was euthanized. The self-made culture medium was formulated using the same recipe of RMPI1640 except for the concentration of Thr, and was supplemented with 10% fetal bovine serum, 25 mM HEPES, 100 U/mL penicillin, 100 μg/mL streptomycin, and 0.25 μg/mL of Fungizone R . All of the aforementioned regents were from Life Technologies, Carlsbad, CA. Additionally, our previous study showed the ex vivo ileal explant cultured for 2 h displayed normal morphology compared to those cultured longer; and the explant model at 20 μg/mL LPS challenge showed acute inflammatory and secretory responses at 2 h of incubation (Zhang et al., 2017).
Experiment 1
Explants were collected from 21-day-old male broilers fed a standard broiler chick starter diet (n = 6). The dose-dependent effect of Thr (zero, 20, 50 and 200 μg/mL) was determined in the presence of either zero or 20 μg/mL LPS (E. coli, serotype O55:B5; Sigma Aldrich, St. Louis, MO). For each treatment, explants were from 6 birds with 3 replicates per bird. After 2 h of incubation, the 3 replicate explants per bird were collected together into RNALater (Ambion, Austin, TX) overnight at 4◦C and frozen at −80◦C until RNA extraction.
Experiment 2
Explants were collected from 20 or 21-day-old male broilers fed either a Thr deficient diet (n = 6) or a Thr control diet (n = 6). Dietary Thr was analyzed to be 4.9 and 9.0 g/kg, which was 61.25 and 112.5% of Thr requirement of broilers in NRC (1994), for the Thr deficient and control diets, respectively. Diets were formulated and mixed as previously described (Zhang et al., 2016). Explants were cultured using 2 concentrations of Thr (zero and 50 μg/mL) in the presence of either zero or 20 μg/mL LPS (E. coli, serotype O55:B5; Sigma Aldrich, St. Louis, MO). For each treatment, explants were from 6 birds with 3 replicates per bird. After 2 h of incubation, the 3 replicate explants per bird were collected together into RNALater (Ambion, Austin, TX) overnight at 4◦C and frozen at −80◦C until RNA extraction.
Experiment 3
Explants were collected from 22-day-old male broilers fed the Thr control diet (n = 6) as in exp. 2. All of the explants were cultured with 50 μg/mL Thr in medium in the presence of either zero or 20 μg/mL LPS (E. coli, serotype O55:B5; Sigma Aldrich, St. Louis, MO). Two inhibitors and their combination were introduced to elucidate the signaling pathways of mucin 2 (MUC2) and pIgR expression as well as regulation of IgA transcytosis. Bay 11–7082 (Selleckchem, Houston, TX), a specific inhibitor for NF-κB at the final concentration of 10 μM, and PD98059 (Selleckchem, Houston, TX), a specific inhibitor for extracellular-regulated protein kinase (ERK) at the final concentration of 50 μM, were used in this study. Explants were pre-treated with inhibitors for 30 min, and then for another 2 h during LPS treatment. For each treatment, explants were from 6 birds with 3 replicates per bird. After 2 h of incubation, one replicate explant per bird was collected into RNALater (Ambion, Austin, TX) overnight at 4◦C and frozen at −80◦C until RNA extraction. The other 2 replicate explants were collected into liquid nitrogen and frozen at −80◦C until IgA measurement.
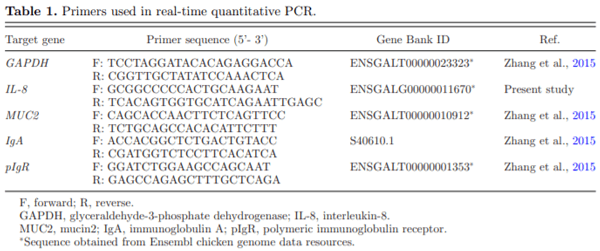
Total RNA Extraction and Reverse Transcription
Total RNA was extracted from the tissues using TRIzol reagent (Invitrogen, Carlsbad, CA) following the manufacturer’s protocol. RNA concentrations were determined by NanoDrop 1000 (Thermo Scientific, Waltham, MA), and RNA integrity was verified by 1% agarose gel electrophoresis. To eliminate contaminating the DNA, extracted RNA was purified with DNA-free DNase Treatment and Removal Kit (Ambion, Austin, TX). Then, 2 μg of total RNA from each sample was reverse transcribed into cDNA using the MMLV reverse transcription system (Promega, Madison, WI), and cDNA was then diluted 1:10 with nuclease-free water (Ambion, Austin, TX) and stored at −20◦C until use.
Quantitative real-time PCR Analysis
Real-time PCR was performed on the Bio-Rad iCycler with the Faststart SYBR green-based mix (Life Technologies, Carlsbad, CA). PCR programs for all genes were designed as: 10 min at 95◦C; 40 cycles of 95◦C for 30 s, primer-specific annealing temperature for 30 s, and 72◦C for 30 s; followed by melting curve analysis. The primer sequences used for real-time PCR are listed in Table 1. Primer specificity and efficiency were determined by using pooled samples. All primer sets with DNA amplification efficiencies between 90 and 110% were used for analysis. All reactions were analyzed in duplicate and a coefficient of variation equal to or less than 5% was deemed acceptable. Water served as a no-template control. Quantification of target transcripts was performed using the 2−ΔΔCt method (Livak and Schmittgen, 2001) with normalization against the housekeeping gene of glyceraldehyde-3-phosphate dehydrogenase (GAPDH).
IgA Measurement
Collected explants were homogenized in ice-cold PBS and centrifuged for 15 min at 1,500 × g to obtain the supernatant. Concentration of IgA in the supernatant was determined by ELISA following the manufacturer’s protocol (Bethyl Laboratories, Montgomery, TX). The total protein in the mucosal homogenates was measured colorimetrically based on the Bradford dye-bind method using a commercially available kit (Bio-Rad Laboratories, Hercules, CA) with bovine serum albumin as the standard.
Statistical Analysis
Data are reported as least square means (LSM) ±SE (n = 6) of independent experiments with different birds. All the data were analyzed for normality using the Univariate procedure of SAS 9.3, and any heteroscedastic data were adjusted to achieve normality using a natural logarithm transformation. The Proc Mixed procedure of SAS was used for data analysis. Contrast was used to separate the means of explants from birds fed a Thr deficient diet or Thr control diet. For data of explants from birds fed the same diet, the model accounted for the random effect of bird, the fixed effect of medium Thr concentrations (or inhibitor treatments), LPS challenge, and their interaction. If the interaction was significantly different (P≤ 0.05), comparisons among medium Thr concentrations (or inhibitor treatments) with or without LPS challenge were made using the PDIFF option. medium (P≤ 0.05), and this increase was suppressed by Thr addition into the culture medium in a non-dose dependent manner. In contrast, LPS challenge markedly increased pIgR mRNA expression (P = 0.003), but its expression was not influenced by Thr addition to the media.
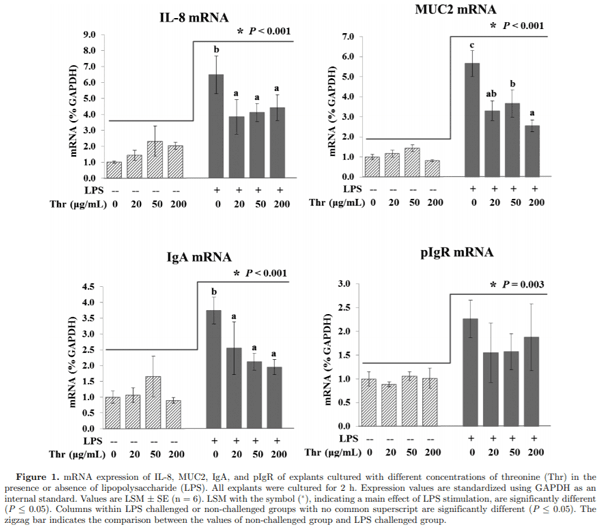
RESULTS
Experiment 1
The effect of Thr concentration on the secretory immune system in the presence or absence of an immune challenge with LPS was determined after 2 h of incubation. As shown in Figure 1, mRNA abundance of interleukin-8 (IL-8), MUC2, and IgA was significantly increased under LPS challenge (P< 0.001). This increase was highest at zero μg/mL Thr in culture
Experiment 2
In order to determine the effect of in vivo Thr deficiency or adequacy on the secretory immune system, explants were collected from birds fed a Thr deficient diet (4.9 g/kg) or a Thr adequate diet (9.0 g/kg). As shown in Figure 2, explants from birds with a Thr deficient diet had higher expression of IL-8 (P< 0.001). Expression of other genes was not affected by the in vivo dietary Thr concentration. Similar to results from exp. 1, explants from birds fed a Thr adequate diet and challenged with LPS had a significant increase in the mRNA expression of IL-8 (P = 0.002), MUC2 (P = 0.001), IgA (P = 0.003), and pIgR (P = 0.04), and this increase had a trend to be higher at zero μg/mL Thr in culture media for IL-8 (P = 0.10), MUC2 (P = 0.07), and IgA (P = 0.09). However, there was no LPS nor medium Thr effect in explants from birds fed a Thr deficient diet.
Experiment 3
To examine the necessary signaling pathways for the induction of IL-8, MUC2, and pIgR as well as regulation of IgA transcytosis, explants were stimulated with LPS in the presence or absence of individual or combined inhibitors of ERK (PD98059) and nuclear factor-κB (NF-κB) (Bay 11–7082) activation. As shown inFigure 3, inhibition of ERK, NF-κB, and their combination reduced the induction of IL-8 by LPS challenge (P≤ 0.05). However, induction of MUC2 by LPS was prevented only by NF-κB inhibition (P≤ 0.05). Interestingly, expression of pIgR was enhanced by ERK inhibition (P≤ 0.05), but suppressed by NF-κB inhibition (P≤ 0.05) under LPS challenge. Additionally, ERK inhibition decreased the proportion of IgA released into the culture medium (P≤ 0.05, Figure 4), indicating a depression of IgA transcytosis from the lamina propria. However, NF-κB inhibition had no effect on IgA transcytosis compared to the control (Figure 4).
DISCUSSION
The flowing mucus, comprised of an outer layer in direct contact with gut flora and an inner layer providing attachments for sIgA, facilitates the formation of sIgA mediated immune exclusion, and thus serves as the first line of intestinal defense (Everett et al., 2004; Abreu, 2010; Slack et al., 2012; Zhang et al., 2015). The high Thr uptake of the gut is largely due to its high occurrence in mucin and sIgA (Sandberg et al., 2007; Nichols and Bertolo, 2008), which are constitutively secreted at a basal level. A variety of factors, such as inflammatory mediators and bacterial infection, may induce additional production of mucin and IgA (Smirnova et al., 2003; McGuckin et al., 2011; Slack et al., 2012), and consequently further increase intestinal Thr utilization (Faure et al., 2007; Remond et al., 2009). Therefore, it was hypothesized that limited luminal Thr availability may impair the sIgA mediated immune defense in the inflammatory state.
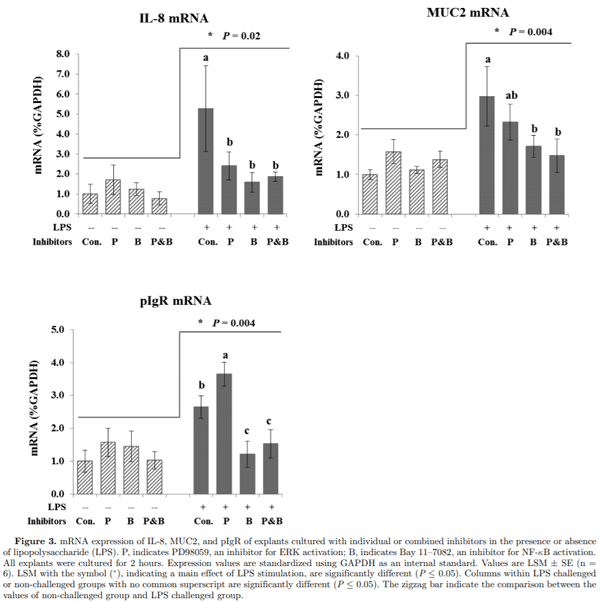
LPS has been widely used to induce inflammatory responses in cells and animals, characterized by inflammatory mediators, such as IL-1β and IL-8 (Hoffmann et al., 2002; Smirnova et al., 2003; Mani et al., 2012). In our study, IL-8 was chosen to indicate the inflammatory response, because it is a hallmark of inflammatory response to recruit heterophils to the injured tissue of chickens (Wigley and Kaiser, 2003) and is primarily regulated at the transcriptional level (Hoffmann et al., 2002). In the absence of LPS challenge, increasing Thr concentration had no effect on mRNA expression of determined genes at 2 h of incubation. However, upon exposure to LPS, increased mRNA expression of IL-8, MUC2, and IgA was even higher in Thr deprivation group at 2 h of incubation, which may reflect a compensatory increase because of the high luminal Thr requirement for the rapid release of mucin and/or IgA in the initiation of inflammation (Deplancke and Gaskins, 2001). This compensatory increase in the presence of LPS challenge is consistent with previous results that more Thr is needed for intestinal protein synthesis under inflammation (Faure et al., 2007; R´emond et al., 2009). As a transmembrane glycoprotein expressed by epithelial cells to mediate pIgA transcytosis, pIgR is the central bridge to connect innate and adaptive immunity in the intestinal secretory immune system (Kaetzel, 2005; Johansen and Kaetzel, 2011). Because of the 1:1 stoichiometry between pIgR and pIgA in the formation of sIgA (Kaetzel, 2005; Johansen and Kaetzel, 2011), it was expected that the mRNA expression of pIgR would have a similar trend to IgA under stress. However, Thr deprivation had no added effect on compensatory increase of pIgR expression with LPS challenge. This lack of pIgR expression induction may be due to the short culture duration of the explant with Thr deprivation, which was not sufficient to induce a change in the protein level of IgA, and thus no induction of pIgR expression. Another possible explanation is that the quick change of IgA transcytosis mediated by pIgR is controlled in the protein synthesis level or other mechanisms, instead of the gene transcription of pIgR.
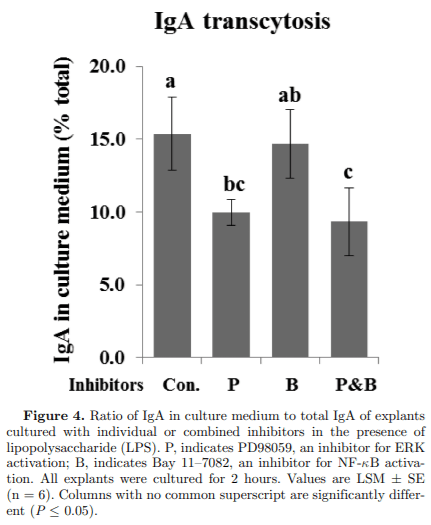
To overcome the limitation of short-term culture, in exp. 2, explants were collected from birds fed a Thr deficient diet or control diet. As expected, explants from birds fed the control diet showed dramatically higher mRNA expression of determined genes with LPS treatment, which was highest when the medium was deprived of Thr. However, explants from birds fed the Thr deficient diet had no response to LPS nor medium Thr supplementation. Therefore, in vivo Thr deficiency may have impaired inflammatory and secretory immune responses. Additionally, IL-8 mRNA abundance from the in vivo Thr deficient explants was higher, probably indicating that the Thr deficient explants already had some level of basal inflammation before they were treated with LPS. Our previous animal study showed that dietary Thr deficiency increased gut permeability (Zhang et al., 2016), which may cause intestinal inflammation by allowing increased permeation of luminal antigens (Arrieta et al., 2006). This inflammatory state may account for the unresponsiveness of MUC2, IgA, and pIgR expression to LPS challenge, because elevated inflammatory cytokine production and imbalance during a state of Thr deficiency could cause further damage to the epithelial barrier integrity and function (Arsenescu et al., 2008; Xu et al., 2014).
Recognition of LPS by epithelial cells and immune cells could lead to activation of multiple signaling pathways, which eventually induce the production of inflammatory chemokines, cytokines, and cellular components (Hoffmann et al., 2002; Abreu, 2010; Mani et al., 2012). The most important signaling inductions are NF-κB pathway and mitogen-activated protein kinases (MAPK) pathways; the latter include cascades of ERK, c-Jun N-terminal kinase (JNK), and p38 MAPK (Hoffmann et al., 2002). In the chicken ileal explants, the results showed expression of IL-8 by LPS appeared to be under the regulation of both NF-κB and ERK pathways as described in human cells (Hoffmann et al., 2002). However, the expression of MUC2 by LPS was up-regulated only by the NF-κB path-way, which was not consistent with the previous study that both ERK and NF-κB activations were required in MUC2 transcription (Li et al., 1998). Additionally, for pIgR transcription, NF-κB activation was required, whereas ERK inhibition led to its up-regulation, which is contrary to the results from human cell lines where pIgR expression was up-regulated by NF-κB activation but not influenced by the MAPK/ERK pathway (Bruno et al., 2010; Bruno et al., 2011). Model differences between human cell culture and chicken ileal explants could have accounted for the differences in the mode of MUC2 and pIgR regulations. One of the obvious differences is that the influence of IgA transcytosis on pIgR expression is absent in the single epithelial cell culture. Su et al. (2010) reported ERK activation was a process required in human IgA transcytosis, which was confirmed in chicken ileal explants with the down-regulation of IgA transcytosis by ERK inhibitor in the current study. ERK inhibition would induce IgA accumulated in the basolateral side of epithelial cells to stimulate more protein synthesis and/or gene transcription of pIgR (Su et al., 2010; Diebel and Liberati, 2011); therefore, the aforementioned pIgR up-regulation with ERK inhibition may reflect a compensatory effect to the blocked IgA transcytosis. Overall, it is likely that pIgR transcription could be controlled directly by NF-κB activation and indirectly by IgA transcytosis.
In conclusion, chick ileal explants with Thr deprivation from the culture medium for 2 h suffered higher inflammatory and secretory immune responses with LPS stimulation, as characterized by the highest gene expression of IL-8, MUC2, and IgA. However, explants from birds fed a Thr deficient diet after 2 h of incubation had no response to LPS challenge, and the compensatory increase of gene expression by Thr deprivation from the culture medium upon LPS exposure was not detected. These observations suggested the explants from in vivo Thr deficiency may have impaired inflammatory and secretory immune responses. Additionally, transcription of MUC2 and pIgR in epithelial cells could be regulated through the NF-κB pathway. ERK activation is involved in regulation of IgA transcytosis and may indirectly contribute to pIgR gene expression. Further studies are warranted to explore whether NF-κB and ERK pathways are implicated in the impaired inflammatory and secretory immune responses upon in vivo Thr deficiency.
This article was originally published in 2017 Poultry Science 96:3043–3051. http://dx.doi.org/10.3382/ps/pex111. This is an Open Access article under a Creative Commons Attributions License. This work is written by (a) US Government employee(s) and is in the public domain in the US.