Control methods are needed to abate NH3 losses from swine anaerobic lagoons to reduce the contribution of confined swine operations to air pollution. In a 15-mo meso-scale column study, we evaluated the effect of manure pretreatment on water quality, reduction of N losses, and sludge accumulation in swine lagoons using (i) enhanced solid–liquid separation with polymer (SS) and (ii) solid–liquid separation plus biological N treatment using nitrification–denitrification (SS + NDN). A conventional anaerobic lagoon was included as a control. Concentrations of total Kjeldahl N (TKN), total ammoniacal N (TAN), and NO3–N were monitored during the course of the study, and the volumes of column liquid and sludge were used to estimate N mass flows. At the end of the study, TKN and TAN concentrations in the liquid of SS columns were 35 and 37% lower than the control, respectively, and TKN and TAN concentrations in SS + NDN were 97 and 99% lower than the control. The N mass flow analysis revealed that SS reduced total N inflow by 30% and SS + NDN by 82% compared with the control. The SS was ineffective at reducing NH3 losses compared with the control. Instead, SS + NDN effectively reduced total NH3 losses by 50%, most of which occurred during the first 6 mo of the study. Although both pretreatments can stop the mass accumulation of total N in sludge, SS + NDN had the advantage of improving water quality and abating NH3 emissions from treated lagoons. As an additional environmental benefit, SS + NDN effluents could be used for crop irrigation without the risk of NH3 losses during land application.
Anaerobic lagoons are widely used across the southeastern United States to store and treat wastewater generated from confined swine operations (Barker, 1996). During lagoon storage and treatment of swine manure, gaseous losses of nitrogen (N) in the form of ammonia (NH3) occur as a result of mineralization of organic N compounds and subsequent NH3 volatilization (Blunden and Aneja, 2008). In North Carolina, intensification of swine production and widespread use of anaerobic lagoons followed by land application of their effluents can contribute to air pollution and regional NH3 atmospheric deposition (Mallin, 2000; Aneja et al., 2008, Schinasi et al., 2011). Thus, there is major public interest in best control technologies to reduce or eliminate NH3 losses from confined swine production (Aneja et al., 2009).
Several approaches have been suggested to control NH3 emission from swine lagoons, including the use of additives (McCrory and Hobbs, 2001), lagoon aeration (Westerman and Bicudo, 2002), permeable and impermeable covers (Ndegwa et al., 2008), and lagoon pretreatment (Day and Funk, 2002). The lagoon pretreatment approach has the advantage of preventing NH3 losses by reducing the N mass load in liquid manure before lagoon storage. The following two pretreatment technologies have the potential to reduce N loads from raw liquid manures: (i) enhanced solid–liquid separation with polymers (Vanotti and Hunt, 1999) and (ii) enhanced solid–liquid separation followed by nitrification–denitrification (Vanotti and Szogi, 2008).
As a lagoon pretreatment method, solid–liquid separation has been traditionally used to reduce lagoon solids buildup by separating solids from liquid raw manure before lagoon input (Barker, 1996). Usually, solid–liquid separation efficiencies of manure separators are less than 68% solids removal (Chastain et al., 2001). However, new advances over the last 15 yr in equipment and flocculant polymer applications developed for enhancing separation treatment have improved removal efficiency of solids and specific plant nutrients such as N and phosphorus (P) (Hjorth et al., 2010). For example, in a full-scale study, swine manure was pretreated before lagoon storage using a high-rate solid–liquid separation system combined with flocculant (polyacrylamide) injection (Szogi and Vanotti, 2007). The authors found the system annually separated 85% of total suspended solids (TSS) and 35% of total N (TN) from the liquid entering the lagoon.
Enhanced solid–liquid separation followed by nitrification– denitrification treats the entire waste stream from a swine operation in a multistep system that includes enhanced solid– liquid separation followed by nitrification–denitrification and soluble phosphorus removal (Vanotti et al., 2005). An evaluation of this wastewater treatment system met all the criteria to qualify as an environmentally superior technology in North Carolina (Williams, 2009), and it showed a reduction of 73% in TSS and 85% in TN in the lagoon liquid (Vanotti and Szogi, 2008).
Because lagoon pretreatment reduces solids and nutrient loadings into the lagoon, significant water quality changes were observed along with replacement of anaerobic lagoon treatment and conversion into water-holding ponds (Vanotti and Szogi, 2008). To manage these lagoons during the course of conversion to water-holding structures, new data are needed on water quality changes, gaseous losses of N, and N mass accumulation in sludge to better understand the physical, chemical, and biological processes occurring in retrofitted swine lagoons. The objective of our study was to evaluate the effect of liquid swine manure pretreatment on water quality improvement, reduction of N losses from swine lagoons, and N accumulation in lagoon sludge using solid–liquid separation alone or in combination with biological N removal. The evaluation included a lagoon N mass flow analysis using mass balance techniques to account for lagoon N losses (Muck and Steenhuis, 1982). This study was performed in the field using meso-scale lagoon columns that replicated the management of anaerobic lagoons receiving pretreated liquid swine manure. Our study included a control treatment that replicated a conventional anaerobic lagoon receiving untreated liquid swine manure from a pit recharge system.
Materials and Methods
Column Experiments
The column experiment was set up in the field approximately 15 m from a full-scale anaerobic lagoon that was retrofitted to a second-generation wastewater treatment system (Vanotti et al., 2009). The column study consisted of three treatments with two replicates. The columns comprised a total of six vessels made of clear polyvinyl chloride pipe (0.153 m i.d., 2.1 m length). The length of the columns was chosen to replicate the average depth (2.0 m) of the adjacent anaerobic lagoon. Thus, each column contained a liquid volume of 36.8 L. A metal rack supported the six columns in a vertical position. All sides of the metal rack were covered with plywood to maintain the columns in the dark except for the top 0.3 m of each column, in which liquid was exposed to natural light (Fig. 1). The columns were placed under a carport to simplify the water balance, eliminating the dilution effect from rainfall.
At the onset of the experiment, each column received 14.2 L of sludge and 22.6 L of liquid obtained from the adjacent anaerobic lagoon (Table 1). The liquid and the sludge settled for a week before weekly loading of pretreated or untreated liquid manure. The column study was performed for about 15 mo (20 Feb. 2007 to 15 May 2008) using effluents taken from different points of the second-generation wastewater treatment plant (Vanotti et al., 2009). The following three types of liquids were applied to the columns on a weekly basis: (i) liquid raw manure from a pit recharge system (control), (ii) liquid from the flocculant-enhanced solid–liquid separation module (SS pretreatment), and (iii) liquid from the biological N module that used nitrification/denitrification after solid–liquid separation (SS + NDN pretreatment). The mean concentrations of water quality parameters for the control and pretreated manure liquids before input into columns are shown in Table 2.
From each column, a 0.42-L sample was removed weekly at 0.6 m below the liquid level using a peristaltic pump and replaced with the corresponding control or pretreated liquid. The volume of column liquid replaced weekly was estimated as the equivalent volume to replace the entire liquid volume of 24,145-m3 of a full-scale anaerobic swine lagoon receiving pretreated water at a rate of 39 m3 d-1 (1.7 yr hydraulic retention time) (Szogi et al., 2006). Tap water was added weekly to each column vessel to replenish evaporation losses.
Once a month, the sludge in each column was stirred using a plunger to avoid excessive compaction and to maintain the moderately viscous consistency of the liquid–sludge interface commonly found in anaerobic lagoons (Schmidt, 2013). The plunger, consisting of a flat 0.14-m-diameter polyvinyl chloride disc with five perforations, was attached to a weight and slowly lowered from the top of each vessel into the sludge. The sludge was stirred for 1 min by gently moving the plunger up and down.
At the end of the 15-mo study, the volumes of supernatant liquid and sludge were measured for each column. The supernatant liquid was removed from each column without disturbing the sludge using a pump connected to plastic tubing that reached the surface of the sludge. After the supernatant liquid was removed, the sludge remaining in the bottom of the column was recovered by inverting the column to convey the sludge into 8-L plastic containers. A small volume (<1 L) of supernatant liquid was used to rinse and remove sludge attached onto the wall of the vessel. The 8-L plastic containers containing sludge were closed with a plastic lid and transported to the laboratory. After the sludge settled in the plastic containers, a thin layer of supernatant liquid was removed using a peristaltic pump. The final volume of sludge was estimated by measuring the equivalent volume of distilled water occupied by the settled sludge in the plastic container. Homogenized sludge samples for chemical analysis were obtained by stirring it at 50 rpm using a mechanical overhead stirrer.
Studies on anaerobic lagoons have shown that lagoon temperature is the most important parameter regulating N concentrations and NH3 flux from swine lagoons because of its large variations during the year (Aneja et al., 2008; Ro et al., 2008). To verify how closely columns replicated the typical seasonal water temperatures of the anaerobic lagoon, the control treatment column and the adjacent anaerobic lagoon were instrumented with temperature data loggers (0.5 m below liquid surface). Weekly average water temperatures of the columns were very similar and highly correlated (R2 = 0.95; P < 0.0001) to the weekly average water temperatures of the full-scale anaerobic lagoon (Fig. 2).
Analytical Methods
Wastewater and sludge analyses were performed according to Standard Methods for Examination of Water and Wastewater (APHA, AWWA, and WEF, 1998). Total solids (TS), volatile solids (VS), and TSS were determined according to Methods 2540 B, D, and E, respectively. The closed reflux colorimetric method was used to determine chemical oxygen demand (COD) (Method 5220 D). Total Kjeldahl N (TKN) was determined using acid digestion and the automated ascorbic acid and phenate methods adapted to digested extracts (Technicon Instruments Corp., 1977). Total ammoniacal nitrogen (TAN) was determined by the automated phenate method (Method 4500-NH3 G) and NO3–N (nitrate plus nitrite) by the cadmium reduction method (Method 4500-NO3 F) after filtration through a 0.45-μm membrane filter (Gelman Supor-450; Pall Corp.). Total organic N (TON) was calculated as the difference between TKN and TAN. Total N (TN) was the sum of TKN and NO3–N. Concentrations of total phosphorus (TP), Cu, and Zn were determined using nitric acid/peroxide block digestion (Peters, 2003) and inductively coupled plasma analysis (Method 3125 A). The pH was determined electrometrically (Method 4500-H+ B), and electrical conductivity (EC) was determined by Method 2510 B. Alkalinity was determined by acid titration to the bromocresol green endpoint (pH 4.5) and expressed as mg CaCO3 L-1 (Method 2320 B). Oxidation-reduction potentials (ORPs) were measured in the lagoon liquid at the time of sampling using an Ag/AgCl reference electrode and corrected to standard hydrogen electrode values (Method 2580 B).
Column influents were analyzed biweekly for all water parameters (Table 2). The ORP was measured in the column liquid weekly. Column effluent samples were analyzed biweekly for COD, TAN, NO3–N, pH and alkalinity and monthly for TS, VS, TSS, TKN, TP, Cu, and Zn. All analyses were combined on a monthly average basis to evaluate water quality and mass flow results. The temperature of lagoon liquid was measured continuously at 0.5 m below the water surface and stored in a StowAway TidbiT data logger (Onset Computer Corp.). Weekly temperature averages were computed from hourly water temperature logged records.
Statistical Analysis
A repeated measures analysis was conducted using the MIXED procedure of SAS (SAS Institute, 2011) to evaluate whether the time trajectories of selected water quality parameters for the three pretreatments started to diverge from their initial values at the onset of the experiment. A first-order autoregressive covariance structure AR(1) in SAS was used to test the effects of Pretreatment, Time, and Pretreatment × Time interaction. The differences in response patterns were considered different among treatments when the probability of F values for the interaction Pretreatment × Time was P < 0.01. At the end of the 15-mo study, water quality and sludge chemical composition were statistically analyzed using the ANOVA procedure of SAS. Treatment means were compared using the least square difference (LSD) option and were considered different when the probability values were P < 0.01.
Mass Flow Analysis
The mass flow analysis approach used to estimate the N loss from lagoon columns included the following three components: (i) the initial N mass (IM) of the column, (ii) the N mass flow (MF) in and out the column during the 15-mo study, and (iii) the recovered N mass (RM) at the end of the experiment. The N mass of each component was calculated as the product of N concentration and its volume. The IM and the RM of the column were computed as the sum of the N mass of the liquid and sludge fractions. Then, the N mass loss (ML) from each column was estimated as the difference between the initial mass plus the in and out mass flow and the recovered mass (ML = IM + MF – RM). Using this approach, the MLs were estimated for TN (TN = TKN + NO3–N), TAN, and TON (TON = TKN – TAN).
Results and Discussion
Water Quality Improvements by Influent Pretreatment
The effects of pretreatments on select water quality parameters during the study are presented in Fig. 3, where each data point is the monthly average of two lagoon column replicates. The criterion to determine water quality improvement was the lowering in concentration of water quality parameters with respect to the control. According to the statistical analyses, differences over time for pH and ORP were not significant among the treatments. During the entire study, the slightly basic pH (7.5–8.5) for all treatments was typical of anaerobic lagoons (Barker, 1996). The ORP range of -200 to +300 mV indicated that all treatments had uniform typical anaerobic conditions (Szogi et al., 2004), except for one observation of the SS + NDN pretreated lagoon. On the other hand, the response patterns of VS, TSS, COD, TKN, TAN, EC, and TP significantly diverged over time (Pretreatment × Time interaction; P < 0.01) for all treatments from their uniform concentration at the onset of the experiment (Fig. 3).
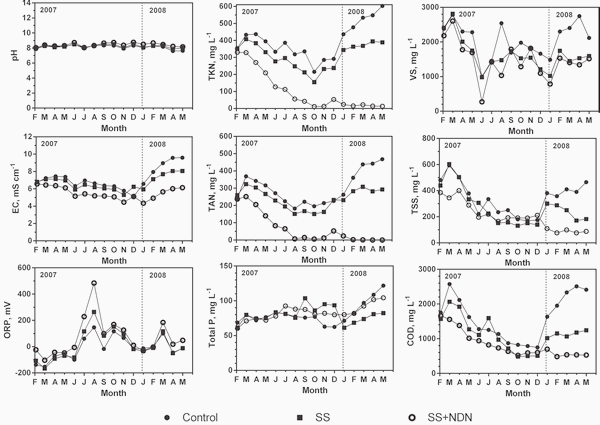
Fig. 3. Water quality changes in liquid of columns during the 15-mo study. Each data point is the monthly average of two replicates. Values at the end of the study are summarized in Table 3. COD, chemical oxygen demand; EC, electrical conductivity; ORP, oxidation reduction potential; SS, enhanced solid–liquid separation; SS + NDN, solid–liquid separation plus biological N treatment using nitrification–denitrification; TKN, total Kjeldahl nitrogen; TAN, total ammoniacal nitrogen; TSS, total suspended solids; VS, volatile solids.
Considerable variability in water quality parameter concentrations occurs seasonally in anaerobic lagoons, in particular, TKN and TAN (Bicudo et al., 1999). These parameters usually increase in winter, with the highest concentration at the beginning of spring, and decrease in summer, with the lowest concentration at the beginning of fall. Westerman et al. (2010) reported that the concentration of TN in the liquid of anaerobic swine lagoons varies seasonally (range, 200–1000 mg TN L-1), usually showing an increase in winter and a decrease in summer. They concluded that the seasonal fluctuation of TKN and TAN concentrations in anaerobic lagoons is due to the effect of temperature on microbial activity and NH3 volatilization increasing during warm weather. In our study, we found similar seasonal trends for the control treatment after changes of water temperature (Fig. 2 and 3). In particular, TSS, COD, EC, TKN, and TAN decreased in concentration during warm weather (spring and summer, 2007), with the lowest concentrations during the fall (2007), and reached the highest concentrations with cold weather (winter 2008). However, water quality parameters in the SS and SS + NDN pretreated columns changed the described typical seasonal trend (Fig. 3). This change was more pronounced in the SS + NDN pretreatment; the concentrations of TSS, COD, TKN, and TAN decreased continuously from the onset until the end of the experiment.
At the end of the 15-mo study, differences in TS and pH between the control and the two lagoon pretreatments were not statistically significant (Table 3). In all columns, total dissolved solids (TDS = TS – TSS) were a large portion of TS (95–99%) (Table 3), which explains the lack of significant differences in TS concentration levels among supernatant liquids. However, as a result of solids removal, differences in mean concentration levels of VS, TSS, COD, TKN, TAN, TP, Cu, Zn, alkalinity, and EC were significant between the control and each pretreatment (Table 3). For instance, TSS and COD concentrations were 53 and 40%, respectively, lower for the SS treatment than for the control. Total suspended solids and COD concentrations were even lower (80 and 72%, respectively) for the SS + NDN treatment compared with the control. At the end of the 15-mo study, average TKN and TAN concentrations in the columns receiving SS pretreatment were 35 and 37%, respectively, lower than the control. With the SS + NDN pretreatment, the decrease in concentration was 97% for TKN and 99% for TAN compared with the control (Table 3).
Effect of Influent Pretreatments on Sludge
The mean concentrations and standard deviations of water quality parameters at the end of the 15-mo study are shown in Table 4 for the sludge in the three lagoon treatments. Typically, lagoon liquid has lower concentrations of most water quality parameters than the lagoon sludge, but the concentration differences are less for soluble nutrients such as TAN (Bicudo et al.,1999). Similar trends were found in our study, with lower concentrations of TS, VS, TSS, COD, TKN, TP, Cu, Zn, and Alkalinity in the lagoon liquid (Table 3) versus those in the lagoon sludge (Table 4). Unlike the lagoon liquid, differences in concentration of all water quality parameters measured in the sludge were not significant among treatments because the source of sludge was a lagoon that had been in operation for over 10 yr. However, a significant difference was observed among the average sludge volumes from the control and pretreatments at the end of the 15-mo study: 7.02 ± 0.6 L in the control lagoon, 4.54 ± 0.6 L in the SS, and 3.53 ± 0.5 L in SS + NDN (LSD0.01 = 1.51 L). Thus, the sludge volumes with SS and SS + NDN were 35 and 50%, respectively, smaller than the control. The TKN, TAN, and NO3–N concentrations and sludge volumes were used to estimate N mass flows for each treatment as discussed in the following section.
Nitrogen Mass Flow Balances
Mass flow balances have been effective at quantifying gaseous losses of N from experiments with precise measurements of input-output masses, such as our column study (Arogo et al., 2003). Gaseous losses of N are calculated from N mass in the initial storage unit (or lagoon column), N mass flow inputs and outputs through the storage unit during the evaluation period, and the leftover mass of N in the storage unit at the end of the evaluation (Muck and Steenhuis, 1982). Our study further separated the organic and inorganic N fractions in the liquid and sludge compartments of each column treatment (Fig. 4). Each graph in Fig. 4 includes ML, which is the difference between the IM plus MF in and out during 15 mo and the RM at the end of the study for each of the three treatments (ML = IM + MF – RM).
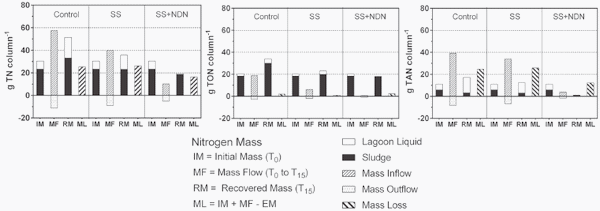
Fig. 4. Nitrogen mass flows for treatments of the column experiment. ML, nitrogen mass loss; SS, enhanced solid–liquid separation; SS + NDN, solid–liquid separation plus biological N treatment using nitrification–denitrification
The ML of TN for the control was 25.5 g N column-1 (3.14 g N m-2 d-1). On an annual basis, the ML per lagoon surface area for the conventional column (control) was 11,461 kg N ha-1 yr-1, which is comparable to the total annual NH3 emissions of 13,600 kg N ha-1 yr-1 measured in a conventional full-scale anaerobic lagoon in North Carolina (Szogi et al., 2006). Whereas the ML of 26.2 g TN column-1 (3.23 g N m-2 d-1) for the SS was similar to the control, the ML of 16.4 g TN column-1 (2.02 g m-2 d-1) for the SS + NDN was 64% smaller than the control. These TN losses included the loss of NH3 (75–98%), TON (2–14%), and NO3–N (0–11%) (Fig. 5). Although NH3 was likely lost by volatilization, release of NH3 by mineralization of organic N followed by volatilization may explain TON losses. Losses of NO3–N could only occur in the SS + NDN columns because their influent had an average NO3–N content of 215 mg L-1. Under the favorable anaerobic conditions of the column (ORP, -200 to +300 mV), the influent NO3–N would be lost by denitrification.
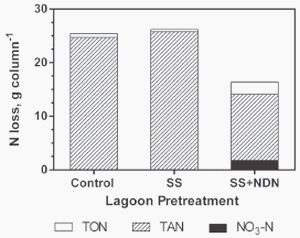
Fig. 5. Forms of N contributing to the total N mass loss from each treatment of the column experiment. SS, enhanced solid–liquid separation; SS + NDN, solid–liquid separation plus biological N treatment using nitrification–denitrification; TAN, total ammoniacal N; TON, total organic N.
Overall, the SS was ineffective at reducing the ML of TAN (25.8 g N column-1 or 3.18 g TAN m-2 d-1) compared with the ML of the control (24.7 g TAN column-1 or 3.04 g TAN m-2 d-1). These results may be related to the reduction of TAN adsorption capacity due to solids removal. It has been suggested that TAN adsorbed to suspended solids may not dissociate and volatilize (De Visscher et al., 2002). Instead, a significant removal of TSS from the lagoon influent may have enhanced TAN volatilization losses rather than reducing them in the treatment columns. For the SS + NDN columns, TAN losses (12.3 g N column-1 or 1.52 g TAN m-2 d-1) were 50% lower than the ML of the control. These TAN losses occurred mostly during the first 6 mo of the study (Feb.–Aug. 2007). The steep decline in TAN concentration for the SS + NDN is an indication of significant TAN losses from the onset of the experiment until August 2007 (Fig. 3). After August 2007, the ML of TAN was significantly reduced, and average influent TAN concentrations in the SS + NDN were consistently low during the entire study (154 mg L-1) relative to the control (average, 1572 mg L-1) (Table 3). Therefore, the TAN concentrations in the SS + NDN column were negligible from August 2007 until the end of the study in May 2008 (Table 3). A mass flow analysis for the last 9 mo of the study (Sept. 2007 to May 2008) was computed to estimate the reduction in TAN losses of the SS + NDN column compared with the control. For this additional mass flow analysis of the SS + NDN, we assumed (i) negligible losses of N from the sludge into the liquid column and (ii) that ML of TON occurred by mineralization releasing TAN into the lagoon column. In summary, the ML of TAN was computed as the sum of MF of TAN plus the ML of TON minus RM of TAN in the liquid column (MLTAN = MFTAN + MLTON − RMTAN). The ML of TAN of the SS + NDN was 3.4 g TAN column-1 (or 0.4 m-2 d-1) and represented a 77% reduction in ML with respect to the ML of the control (14.8 TAN column-1 or 1.8 m-2 d-1) in the remaining 9 mo of the study (Sept. 2007 to May 2008).
The accumulation of N in sludge was significantly affected by the solids removal with both liquid pretreatments. For the control columns, the mass of TN and TON built up during the 15 mo of weekly loadings with raw liquid manure (Fig. 4). The mass accumulation of TN and TON in the control columns represents the accrual of a layer of denser, more refractory, stable material underlying the more biologically active liquid zone, typical of anaerobic swine lagoons (Sheffield et al., 2000). However, the TN mass input was reduced with the SS pretreatment by 30% (40.2 g N column-1 or 4.95 g N m-2 d-1), whereas the SS + NDN pretreatment reduced the TN mass input by 82% (10.3 g N column-1 or 1.27 g m-2 d-1) compared with the control (57.6 g N column-1 or 7.10 g m-2 d-1). The mass flow analysis also revealed that continuous high-rate removal of solids with the SS or the SS + NDN pretreatment stopped the accumulation of TN and TON in sludge (Fig. 4).
In summary, the results on water quality changes and N mass flow analysis of our column study show that SS and SS + NDN pretreatments significantly differ in their efficiency to reduce NH3 emissions from lagoons. Although SS pretreatment was ineffective at reducing lagoon NH3 losses, it stopped the accumulation of solids in lagoon sludge. Because conventional anaerobic lagoons require sludge management (Schmidt, 2013), the SS would eliminate regular sludge removal from retrofitted lagoons, land requirements, and transportation costs for sludge disposal. In addition, solids removal alone can offer the opportunity for recovery of nutrients and energy from manure solids through biological or thermochemical conversion methods (Cantrell et al., 2008).
The SS + NDN can offer similar benefits as SS regarding sludge management, but it has the advantage of significantly reducing the NH3 concentration in lagoon liquid and effectively reducing NH3 mass losses. Because the SS + NDN pretreatment option would convert anaerobic lagoons into water-holding facilities, the potential exists for the use of treated water to irrigate cash crops. This alternative use of treated lagoon liquid would discontinue the typical spray field disposal of lagoon effluents and eliminate the associated NH3 losses during land application
Acknowledgments
Mention of trade names or commercial products in this article is solely for the purpose of providing specific information and does not imply recommendation or endorsement by the U.S. Department of Agriculture. This research was part of USDA-ARS National Program 214: Manure and By-Product Utilization; ARS Project 6657-13630- 001-00D “Innovative animal manure treatment technologies for enhanced environmental quality.” The authors thank Mr. and Mrs. Billy Tyndall for providing their farm for this study and Ms. Aprel Ellison and Mr. William Brigman for assistance in collection of wastewater samples and water quality analyses.
This article was originally published in Journal of Environmental Quality. 43:1219–1226 (2014). doi:10.2134/jeq2013.08.0330.
References
Aneja, V.P., S. Pal Arya, D.-S. Kim, I.C. Rumsey, H.L. Arkinson, H. Semunegus, and K. Bajwa. 2008. Characterizing ammonia emissions from swine farms in Eastern North Carolina: Part 1- Conventional lagoon and spray technology for waste treatment. J. Air Waste Manage. Assoc. 58:1145– 1157. doi:10.3155/1047-3289.58.9.1145
Aneja, V.P., W.H. Schlesinger, and J.W. Erisman. 2009. Effects of agriculture upon the air quality and climate: Research, policy, and regulations. Environ. Sci. Technol. 43:4234–4240. doi:10.1021/es8024403
APHA, AWWA, and WEF. 1998. Standard methods for examination of water and wastewater. American Public Health Association, Washington, DC.
Arogo, J., P.W. Westerman, and A.J. Heber. 2003. A review of ammonia emissions from confined swine feeding operations. Trans. ASAE 46:805–817.
Barker, J.C. 1996. Lagoon design and management for livestock waste treatment and storage. Pub. EBAE 103-83. North Carolina Cooperative Extension Service, Greensboro and Raleigh, NC.
Bicudo, J.R., L.M. Safley, and P.W. Westerman. 1999. Nutrient and sludge volumes in single-cell recycle anaerobic swine lagoons in North Carolina. Trans. ASAE 42:1087–1093. doi:10.13031/2013.13256
Blunden, J., and V.P. Aneja. 2008. Characterizing ammonia and hydrogen sulfide emissions from a swine waste treatment lagoon in North Carolina. Atmos. Environ. 42:3277–3290. doi:10.1016/j.atmosenv.2007.02.026
Cantrell, K.B., T. Ducey, K.S. Ro, and P.G. Hunt. 2008. Livestock waste-tobioenergy generation opportunities. Bioresour. Technol. 99:7941–7953. doi:10.1016/j.biortech.2008.02.061
Chastain, J.P., W.D. Lucas, J.E. Albrecht, J.C. Pardue, J. Adams, III, and K.P. Moore. 2001. Removal of solids and major plant nutrients from swine manure using a screw press separator. Appl. Eng. Agric. 17:355–363.doi:10.13031/2013.6209
De Visscher, A., L.A. Harper, P.W. Westerman, Z. Liang, J. Arogo, R.R. Sharpe, and O. Van Cleemput. 2002. Ammonia emissions from anaerobic swine lagoons: Model development. J. Appl. Meteorol. 41:426–433. doi:10.1175/1520-0450(2002)041<0426:AEFASL>2.0.CO;2
Day, D.L., and T.L. Funk. 2002. Processing manure: Physical, chemical and biological treatment. J.L. Hatfield and B.A. Stewart, editors, Animal waste utilization: Effective use of manure as a soil resource. Lewis Publishers, Boca Raton, FL. p. 243–282.
Hjorth, M., K.V. Christensen, M.L. Christensen, and S.G. Sommer. 2010. Solidliqui separation of animal slurry in theory and practice: A review. Agron. Sustainable Dev. 30:153–180. doi:10.1051/agro/2009010
Mallin, M.A. 2000. Impacts of industrial animal production on rivers and estuaries. Am. Sci. 88:26–37. doi:10.1511/2000.1.26
McCrory, D.F., and P.J. Hobbs. 2001. Additives to reduce ammonia and odor emissions from livestock wastes: A review. J. Environ. Qual. 30:345–355. doi:10.2134/jeq2001.302345x
Muck, R.E., and T.S. Steenhuis. 1982. Nitrogen losses from manure storages. Agric. Wastes 4:41–54. doi:10.1016/0141-4607(82)90053-1
Ndegwa, P.M., A.N. Hristov, J. Arogo, and R.E. Sheffield. 2008. A review of ammonia emission mitigation techniques for concentrated animal feeding operations. Biosystems Eng. 100:453–460. doi:10.1016/j. biosystemseng.2008.05.010
Peters, J. 2003. Unit III 5.5: Digestion and dissolution methods for P, K, Ca, Mg, and trace elements. Peters, J., editor. Recommended methods of manure analysis (A3769). Univ. of Wisconsin–Extension, Madison.
Ro, K.S., A.A. Szogi, M.B. Vanotti, and K.C. Stone. 2008. Process model for ammonia volatilization from anaerobic swine lagoons incorporating varying wind speeds and gas bubbling. Trans. ASABE 51:259–270. doi:10.13031/2013.24219
SAS Institute. 2011. SAS/STAT software, version 9.3. SAS Institute, Inc., Cary, NC.
Schinasi, L., R.A. Horton, V.T. Guidry, S. Wing, S.W. Marshall, and K.B. Morland. 2011. Air pollution, lung function, and physical symptoms in communities near concentrated swine feeding operations. Epidemiology 22:208–215. doi:10.1097/EDE.0b013e3182093c8b
Schmidt, A.M. 2013. Sludge management for anaerobic lagoons and runoff holding ponds. UNL Extension Pub. G1371. Univ. of Nebraska–Lincoln Extension, Institute of Agriculture and Natural Resources, Lincoln.
Sheffield, R.E., J.C. Barker, and K.A. Shaffer. 2000. Sludge management and closure procedures for anaerobic lagoons, AG604. North Carolina State Univ. Cooperative Extension, Raleigh.
Szogi, A.A., P.G. Hunt, E.J. Sadler, and D.E. Evans. 2004. Characterization of oxidation-reduction processes in constructed wetlands for swine wastewater treatment. Appl. Eng. Agric. 20:189–200. doi:10.13031/2013.15891
Szogi, A.A., and M.B. Vanotti. 2007. Abatement of ammonia emissions from swine lagoons using polymer-enhanced solid-liquid separation. Appl. Eng. Agric. 23:837–845. doi:10.13031/2013.24053
Szogi, A.A., M.B. Vanotti, and A.E. Stansbery. 2006. Reduction of ammonia emissions from treated anaerobic swine lagoons. Trans. ASABE 49:217– 225. doi:10.13031/2013.20241
Technicon Instruments Corp. 1977. Individual/simultaneous determination of nitrogen and/or phosphorus in BD acid digests (dialyzer): Industrial method 337-74W/B. Technicon Instrument Corp., Tarrytown, NY.
Vanotti, M.B., and P.G. Hunt. 1999. Solids and nutrient removal from flushed swine manure using polyacrylamides. Trans. ASAE 42:1833–1840. doi:10.13031/2013.13347
Vanotti, M.B., and A.A. Szogi. 2008. Water quality improvements of wastewater from confined animal feeding operations after advanced treatment. J. Environ. Qual. 37:S86–S96. doi:10.2134/jeq2007.0384
Vanotti, M.B., A.A. Szogi, and P.G. Hunt. 2005. Wastewater treatment system. U.S. Patent 6893,567 B1. Date issued: 17 May 2005.
Vanotti, M.B., A.A. Szogi, P.D. Millner, and J.H. Loughrin. 2009. Development of a second-generation environmentally superior technology for treatment of swine manure in the USA. Bioresour. Technol. 100:5406–5416. doi:10.1016/j.biortech.2009.02.019
Westerman, P.W., J. Arogo Ogejo, and G.L. Grabow. 2010. Swine anaerobic lagoon nutrient concentration variation with season, lagoon level, and rainfall. Appl. Eng. Agric. 26(1):147–152. doi:10.13031/2013.29472
Westerman, P.W., and J.R. Bicudo. 2002. Application of mixed and aerated pond for nitrification and denitrification of flushed swine manure. Appl. Eng. Agric. 18:351–358. doi:10.13031/2013.8598
Williams, C.M. 2009. Development of environmentally superior technologies in the U.S. and policy. Bioresour. Technol. 100:5512–5518. doi:10.1016/j. biortech.2009.01.067