Introduction
Confined swine (Sus scrofa L.) production generates large volumes of wastewater typically stored and treated in anaerobic lagoons. These lagoons usually require a sludge maintenance plan consisting of regular sludge removal followed by land application (Barker, 1996). Anaerobic lagoon liquid and sludge are both rich in nutrients, particularly nitrogen (N), phosphorus (P), copper (Cu), and zinc (Zn). However, P can be the limiting nutrient on land requirements for application of effluent and sludge (Schmidt, 2013). In North Carolina, intensification of swine production and the widespread use of anaerobic lagoons followed by land application of effluents historically increased soil P beyond crop nutrient needs (Cahoon and Ensign, 2004). Thus, regional accumulation of P along with Cu and Zn in excess of soil assimilative capacity have the potential to pollute water resources through soil leaching or runoff (Nelson et al., 2005; Novak et al., 2000; Novak et al., 2008). An alternative to resolve this environmental problem is the pretreatment of liquid raw manure prior to lagoon input to decrease the P, Cu and Zn land application rates while reducing the frequency and cost of lagoon sludge management.
Solid-liquid separation and nitrification-denitrification are two advanced treatment methods for treating liquid swine manure prior to lagoon input (Szogi et al., 2015). Solid-liquid separation methods are used to divide the liquid and solid fractions of manure by physical and chemical processes (Hjorth et al., 2010). The combination of physical (gravity or mechanical) and chemical (flocculant addition) processes have enhanced the separation efficiency of solids and removal of P, Cu and Zn (Vanotti et al., 2009). On the other hand, the aim of the nitrification-denitrification process is to biologically transform ammonium N into innocuous N gas, and successfully used in advanced wastewater treatment after enhanced solid-liquid separation (Vanotti et al., 2005; Vanotti and Szogi, 2008). In a study using experimental lagoon columns, we reported the effect of (i) enhanced solid-liquid separation alone, and (ii) solid-liquid separation plus biological N pretreatments on water quality, N losses, and accumulation of N in swine lagoon sludge (Szogi and Vanotti, 2014). Herein, we report additional new data on P, Cu and Zn concentrations and their mass accumulation in experimental lagoon columns with the objective to better understand the processes occurring in swine lagoons retrofitted with advanced pretreatment technologies.
Materials and Methods
Experimental set up
The meso-scale column lagoon study was located in a swine finishing farm in Sampson Co., North Carolina, USA. The column study was set up in the field, adjacent to an anaerobic lagoon retrofitted with a full-scale second-generation wastewater treatment plant that treated the entire waste stream from the swine finishing operation in a multi-step system that included enhanced solid-liquid separation followed by nitrification-denitrification and soluble P removal (Vanotti et al., 2009).
The lagoon columns were made of 2.1-m length clear polyvinyl chloride pipe (0.153-m i.d.) to replicate the average depth (2.0 m) of the adjacent anaerobic lagoon (Figure 1). The columns were placed under a carport to simplify the water balance, eliminating the dilution effect from rainfall (Westerman et al., 2010). At the beginning of the experiment, each column received 14.2 L of sludge and 22.6 L of liquid obtained from the adjacent anaerobic lagoon to replicate the anaerobic lagoon conditions.
Figure 1 − Meso-scale lagoon column set-up supported with a metal rack. All sides of the metal rack were covered with plywood to maintain the liquid-sludge columns in the dark except for the topmost 0.3 m of each column, which was exposed to natural light.
Effluents taken from three different points in the treatment plant were used for the meso-scale column lagoon study. Thus, the lagoon column study consisted of the following three treatments: (i) liquid raw manure as the influent of the conventional lagoon treatment (control treatment); (ii) liquid from the flocculant-enhanced solid-liquid separation module (treated raw liquid manure in a one-step process) as the influent for the solid separation lagoon pretreatment (SS pretreatment); and (iii) liquid from the biological N module (a two-step process of biological N removal after solid-liquid separation) as the influent for the solid separation plus nitrification-denitrification lagoon pretreatment (SS + NDN pretreatment). The study was conducted with two lagoon column replicates per treatment.
From each column, a 0.42-L sample was removed weekly at 0.6 m below the liquid level using a peristaltic pump and replaced with the corresponding control or pretreated liquid (Table 1). Tap water was added weekly to each column vessel to replenish evaporation losses.
Once a month, the sludge in each column was gently stirred using a plunger to avoid excessive compaction and to maintain the moderately viscous consistency of the liquid-sludge interface commonly found in anaerobic lagoons (Schmidt, 2013). The plunger was constructed using a flat 0.14-m diameter polyvinyl chloride disc with five perforations attached to a weight placed in the center of the disc. The plunger was slowly lowered from the top of each column into the sludge using a string to stir the sludge for about one minute by slowly moving the plunger up and down. The study lasted approximately 15 months (20 Feb 2007 to 15 May 2008). At the end of the 15-month study, the volumes of supernatant liquid and sludge were measured for each column as described by Szogi and Vanotti (2014).
To verify how closely columns replicated the typical seasonal water temperatures of the anaerobic lagoon, the control treatment column and the adjacent anaerobic lagoon were instrumented with temperature data loggers at 0.5 m below liquid surface (HOBO, Onset Computer Corp., Bourne, MA). The weekly mean water temperatures of the columns (TC) during the 15 mo-study were highly correlated and very similar to the weekly mean water temperatures of the adjacent full-scale anaerobic lagoon (TAL) such that TC = 0.92*TAL + 3.00 (R2 = 0.95; p < 0.05).
Analytical methods
Wastewater and sludge analyses were performed according to Standard Methods for Examination of Water and Wastewater (APHA, 1998). Total suspended solids (TSS) were determined according to Method 2540 E, and pH was determined electrometrically (Method 4500-H+ B). Total P (TP) was determined after sulfuric acid digestion and the automated colorimetric method (Szogi and Vanotti, 2009), while soluble reactive P (SRP) was determined using the same colorimetric method after filtration of undigested liquid sample through a 0.45-μm membrane filter. Total Cu, and Zn were determined using nitric acid/ peroxide block digestion (Peters, 2003) and inductively coupled plasma analysis (Method 3125 A).
Table 1 − Composition of the influent liquids in the 15-month column study (means ± standard deviation, n = 15).
Mass flow analysis
A mass flow analysis approach was used for estimating the mass of P, Cu, and Zn accumulated in lagoon columns. For each element, the mass flow analysis included the following three components (Figure 2): (i) initial mass (IM) in the column determined at the beginning of the experiment (T0); (ii) total mass (TM) expected at the end of the 15-month study (T15); (iii) the recovered mass (RM) determined at the end of the experiment (T15). The TM was estimated as IM plus the mass inflow (Min) minus the mass outflow (Mout). The mass of each component was calculated as the product of element concentration and the corresponding volume of liquid or sludge. The total element mass in IM, TM and RM of each column was the sum of the element mass in the liquid and sludge fractions. The net mass input (NMI) to each lagoon column was the difference between IM and RM. The ratio TM/RM was used to estimate the percent mass recovery efficiency.
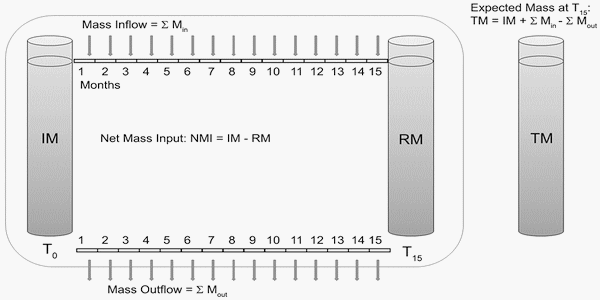
Figure 2 − Schematic diagram of the mass flow balance for phosphorus, copper, and zinc of the meso-scale lagoon columns. IM = Initial mass determined at T0; TM = Total mass estimated at the end of the study (T15); and RM = Recovered mass determined at the end of the 15-month study (T15).
Statistical analysis
Summary statistics were performed using the PROC MEANS procedure from SAS (Statistical Analysis System, version 9.4). Analysis of variance for the effect of pretreatments on lagoon liquid and sludge chemical compositions was conducted using the GLM model from SAS. Treatment means were compared using the least square difference (LSD) option and were considered different when the probability values were p < 0.05. Significant relationships between water quality parameters were conducted using the PROC REG linear correlation and regression analysis from SAS.
Results and Discussion
Effect of pretreatments on water quality
Concentrations of TSS, TP, SRP, Cu, and Zn in the liquid of the lagoon columns were monitored during the course of the study for the control and each pretreatment (Figure 3). Each data point is the monthly average of two lagoon columns. The criterion to determine improved water quality was the decline in concentration of TSS, TP, SRP, Cu, and Zn with respect to the control.
Total suspended solids
In particular, the TSS concentration of the control columns showed a seasonal variation along with the seasonal variation in water temperatures typical of conventional anaerobic lagoons (Figure 3A and B). Because of the particulate organic carbon (C) content in wastewater, TSS concentrations in conventional anaerobic lagoons usually increase in winter, with the highest concentration found at the beginning of the spring, followed by a decrease in the summer, with the lowest concentration found at the beginning of the fall. In previous studies, similar seasonal fluctuations were observed in the concentration of other water quality parameters linked to the organic C content in lagoon liquid, such as chemical oxygen demand and volatile solids (Vanotti and Szogi, 2008); their decrease in concentration during summer and early fall was attributed to favorable water temperatures for microbial degradation of organic C (Westerman et al., 2010). As a result of the effective removal of more than 90 % of the solids from the conventional lagoon inflows (Control - raw manure, Table 1), both SS and SS + NDN treatment showed a TSS trend different from the one described for the control. The concentration of TSS declined continuously from the beginning to the end of the study, with the decline being more prominent in the SS + NDN than in the SS pretreatment (Figure 3A). At the end of the study (T15), the TSS concentrations in the liquid fraction of the columns were significantly lower than the control for both SS and SS + NDN pretreatments (Table 2). Thus, the final TSS concentrations were 53 % and 79 % lower than the control for the SS and the SS + NDN pretreatments, respectively.
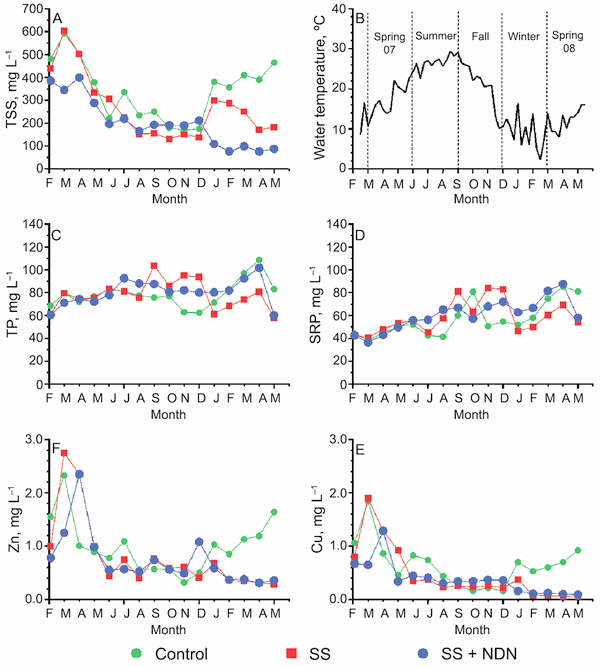
Figure 3 − Changes in concentration and temperature in the liquid of columns during the 15-month study: A: total suspended solids (TSS); B: lagoon water temperature; C: total phosphorus (TP); D: soluble reactive phosphorus (SRP); E: copper (Cu); and F: zinc (Zn). Each data point is the monthly average of two replicates. Values at the end of the study are summarized in Table 2. TSS, total suspended solids; SS, enhanced solid-liquid separation; SS + NDN, enhanced solid-liquid separation plus biological N.
Table 2 − Initial and final mean composition and volume of liquid and sludge in the column experiment.
Total P and soluble reactive P
In North Carolina, swine lagoon liquid have a wide range (60 - 130 mg L−1) of TP concentrations (Bicudo et al., 1999), and a range of 15 - 133 mg TP L−1 have been reported in another study for swine lagoons of finishing farms (Westerman et al., 2010). In our study, monthly mean TP concentrations (62 - 109 mg L−1) for the control lagoon were within the TP ranges reported for North Carolina swine farms. As shown in Figure 3C, the TP and SRP concentrations in the liquid fraction along the 15-month of our study were variable but did not have a consistent seasonal trend like TSS for the control or any of the two pretreatments. Moreover, the TP concentration range of the SS pretreatment (58 - 104 mg L−1) and the SS + NDN pretreatment (60 - 102 mg L−1) were somewhat similar to the control (Figure 3C). Even though SS reduced TP in the inflow by 71 % and SS + NDN reduced TP by 84 % compared to the control, SRP was removed from the lagoon inflow only by 15 % and 28 % with SS and SS + NDN, respectively (Table 1). At the end of the 15-month (T15) study, both pretreatments showed significant reductions of TP and SRP concentrations compared to the control (Table 2). However, mean monthly concentrations of both TP and SRP in the liquid fraction were all negatively correlated with TSS for either the SS or the SS + NDN pretreatment (Table 3). These negative correlation results suggest that even though the SS and SS + NDN pretreatments reduced TP and SRP in the influent, dissolution of P from the sludge into the overlying lagoon liquid sustained the TP and SRP concentrations levels somewhat similar to the control (Figure 3C and D). Further reduction of P levels in the lagoons’ influent can be achieved by including a P removal process in the pretreatment (Szogi and Vanotti, 2009). Addition of a P removal treatment module after SS + NDN has been shown to reduce both the TP (38 %) and SRP (42 %) compared to their initial concentration levels in the liquid of anaerobic lagoon receiving pretreated influents (Vanotti and Szogi, 2008).
Table 3 − Linear correlation coefficients (r) of monthly concentration of total suspended solids (TSS) versus total phosphorus (TP), soluble reactive phosphorus (SRP), Cu, and Zn in the liquid of the 15-month column study (n = 16) of the control, enhanced solid-liquid separation (SS), and enhanced solid-liquid separation plus biological nitrogen (SS + NDN) treatments.
Total Cu and Zn
With solid-liquid separation, effective removal of Cu and Zn (> 87 %) in the liquid fraction has been previously reported (Vanotti et al., 2009; Sommer et al., 2015). In our study, Cu and Zn concentrations in the inflow were reduced compared to the control by 89 % with the SS, and 99 % with the SS + NDN pretreatment (Table 1). In North Carolina, conventional lagoons in finishing farms have a wide range of concentrations of Cu (0.12 - 2.62 mg L−1) and Zn (0.28 - 13.2 mg L−1) because of varying levels in Cu and Zn included in the animal feed (Westerman et al., 2010). In our study, the control lagoon had concentration levels in the lower end of Cu (0.7 mg L−1) and Zn (1.2 mg L−1) reported for North Carolina lagoons (Table 2). The trend of monthly mean Cu and Zn concentrations in the liquid fraction are shown in Figure 3E and F. The concentrations of Cu and Zn in the control column showed a seasonal variation consistent with the seasonal variation of TSS and water temperatures typical of conventional anaerobic lagoons (Figure 3A and B). However, the trend of Cu and Zn in the liquid of the SS and the SS + NDN pretreatment columns were markedly different for the control (Figure 3E and F). The concentration of both Cu and Zn declined continuously from the beginning until the end of the study along with the decline in concentration in TSS. Unlike P, there were strong positive correlations of the mean monthly concentrations of TSS with Cu and Zn concentrations in the liquid of both SS and SS + NDN pretreatment columns (Table 3). These results show that both SS and SS + NDN were effective in reducing Cu and Zn levels in lagoon liquid at the end of the 15-month study. Different from P, once Cu and Zn are removed from the influent liquid, little dissolution of these elements occurs from the sludge into the liquid fraction.
Effect of pretreatment on lagoon sludge
The mean of TSS, TP, SRP, and Cu concentrations, pH, and volume at the beginning and the end of the 15-month column study are shown in Table 2 for the sludge in the control and the two lagoon pretreatments. Typically, lagoon liquid has lower concentrations of most water quality parameters than the lagoon sludge (Bicudo et al., 1999). With the exception of pH, similar trends were found in our study with lower concentrations of TSS, TP, SRP, Cu, Zn in the lagoon liquid than in the lagoon sludge (Table 2). Unlike the lagoon liquid, the concentration of all chemical parameters determined in the sludge were not different between treatments (p > 0.05) because the source of sludge added to the columns at the beginning of the experiment was a lagoon with a legacy of accumulating sludge for over 10 years of operation. However, the average sludge volumes of both pretreatments were different from the control at the end of the 15-month study (T15, Table 2): 7.0 L in the control lagoon, 4.5 L in the SS, and 3.6 in the SS + NDN (p < 0.05). These differences in sludge volume resulted in volumes of SS and SS + NDN that were 35 % and 50 %, respectively, less than the sludge volume of the control. The TP, SRP, Cu, and Zn concentrations and sludge volumes were used to estimate their respective mass flows for each treatment, which are further discussed in the following section.
Mass flow balance
The mass flow balance is an important part of the study for understanding the effect of pretreatment on the dynamics of P, Cu, and Zn in anaerobic lagoons. In an anaerobic lagoon environment, P, Zn, and Cu were considered conservative elements in the mass balance because they do not contribute to gaseous mass losses like non-conservative elements such as N (Szogi and Vanotti, 2014). The mass flow balances adequately determined the effect of the control and the SS and SS + NDN pretreatments on TP, Cu and Zn buildup in the lagoon columns (Table 4). In the last column of Table 4, the percent mass recovery efficiency is presented for all mass flow balances. High mass recovery efficiencies (TM/RM > 87 %) indicate that the total elemental mass expected at the end of the study (estimated from average monthly mass records) was consistent with the final elemental mass recovered. Overall, results in Table 4 reveal a build up of P, Cu and Zn mass in the control columns loaded with untreated liquid manure during the 15-month period compared to the initial mass when comparing RM versus IM. However, the continuous high-rate removal of solids with the SS and the SS + NDN pretreatments reduced the overall accumulation of P, Cu and Zn in the pretreated lagoon columns (Table 4). The net mass input (NMI) of P (Table 4), Cu and Zn during the 15-month study was estimated as the difference between the initial mass and total mass accumulated at the end of the study (RM - IM). Compared to the control, the NMI during the study declined in the lagoon columns which received both pretreatments: the TP net input declined 79 % with SS, and 100 % with SS + NDN; the Cu NMI declined 91 % with SS, and 98 % with SS + NDN; and the Zn NMI declined 91 % with SS, and 97 % with SS + NDN. Therefore, these reductions in net inputs of P, Cu, and Zn resulted in smaller recovered masses (RM) in pretreated columns than in the control (Table 4). The RM of TP were 26 % and 34 % less than the control with SS and SS + NDN pretreatments, respectively. The RM of Cu were less than the control by 30 % with SS and 42 % with SS + NDN. The RM of Zn reduction with respect to the control followed a trend to that of Cu, with RM 31 % and 44 % less than the RM of the control with SS and SS + NDN, respectively.
Table 4 − Total initial mass, mass flow, and recovered mass of P, Cu, and Zn for the treatments applied in the lagoon column experiment (liquid + sludge).
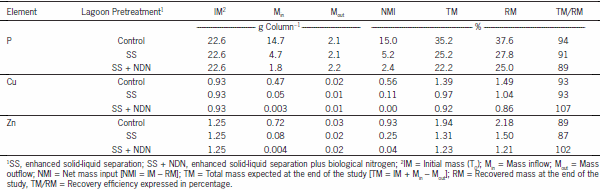
As part of the mass flow balance, the mass of P, Cu, and Zn in the liquid and sludge fractions were determined and expressed per lagoon surface area as shown in Figure 4. The mass of P, Cu, and Zn per square meter of lagoon (g m−2) in the liquid fraction at the beginning of the study (IM T0) and recovered at the end of the 15-month study (RM) for each pretreatment were a small fraction of the total mass (liquid + sludge) fractions in the lagoon columns (< 16 % for P and < 3 % for Cu or Zn). Compared to the sludge fraction that made > 84 % of the total mass of P and > 97 % of the total mass of Cu or Zn in the columns, the SS and SS + NDN pretreatments reduced P, Cu and Zn mass accumulation as compared to the control. The mass reduction of Cu and Zn with the SS + NDN pretreatment was such that it stopped the accumulation of Cu and Zn in the sludge. This is illustrated in Figure 4 where the initial sludge mass of Cu and Zn (IM - T0) for the SS + NDN pretreatment were almost the same as their masses found in the sludge recovered at the end of the study (RM - T15).
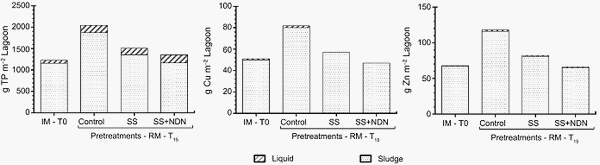
Figure 4 − Mass of phosphorus, copper, and zinc per lagoon surface area at the beginning of the study (IM - T0) and recovered at the end of the 15-month study (RM – T15) in the lagoon liquid and sludge. SS, enhanced solid-liquid separation; SS + NDN, enhanced solid-liquid separation plus biological N.
In summary, the results on concentration changes of P, Cu, and Zn, and mass flow analysis of our lagoon column study show that SS and SS + NDN pretreatments differ significantly in their efficiency in reducing P, Cu and Zn in anaerobic lagoons. Although SS and SS + NDN pretreatments were less effective in reducing soluble P in lagoon liquid, both pretreatments stopped the accumulation of P in the sludge fraction, which comprises most of the mass of P in a lagoon. The significant decrease in Cu and Zn concentrations in the influent was also a major factor in reducing their concentrations in the lagoon liquid and stop their accumulation in the lagoon sludge. Because conventional anaerobic lagoons require sludge management (Schmidt, 2013), the SS treatment using enhanced flocculation would reduce the frequency of sludge removal from lagoons retrofitted with this technology, as well as the land requirements and transportation for sludge disposal. The SS + NDN can offer similar benefits as SS in terms of sludge management with the added advantage of providing biological N treatment followed by P recovery with the potential for using treated water low in N, P, Cu and Zn to irrigate cash crops.
Acknowledgement
Mention of trade names or commercial products in this article is solely for the purpose of providing specific information and does not imply recommendation or endorsement by the U.S. Department of Agriculture. This research was part of USDA-ARS National Program 214: Manure and By-Product Utilization; ARS Project 6082- 13630-005-00D “Innovative Animal Manure Treatment Technologies for Enhanced Environmental Quality.”
This article was originally published in Journal of Environmental Quality. 43:1219–1226 (2014) doi:10.2134/jeq2013.08.0330. This article is reproduced with permission from the author Ariel Szogi, who wrote it as part of his official duties as an Employee of the United States Government and is, therefore, a work of the United States Government. In accordance with 17 U.S.C 105, no copyright protection is available under such law.