Introduction
The intestinal spirochaete Brachyspira hyodysenteriae is the classical causative agent of swine dysentery (SD), a disease typically associated with severe colitis and bloody mucoid diarrhoea mainly seen in grower and finisher pigs [1]. Two related species, Brachyspira suanatina and “Brachyspira hampsonii”, also have been shown to occur in pigs and to cause SD on occasion [2,3]. SD is a common disease that occurs in all pig rearing countries, and it can cause major economic losses in rearing herds due to reduced production, mortalities and costs of treatment. In addition the disease can represent an animal welfare issue if it is not adequately controlled. The occurrence of B. hyodysenteriae in breeding and multiplier herds that supply improved genetic stock to production herds also can cause major disruptions to the industry by impeding the trade and movement of pigs.
Although infection with B. hyodysenteriae can lead to typical SD, isolates of the spirochaete also have been recovered from apparently healthy herds [4–6]. A lack of disease in a colonised herd could be associated with the presence of B. hyodysenteriae strains with reduced virulence [7], the use of an effective medication regimen, and/or the occurrence of diet-related changes in physical or microbiological conditions in the large intestine that make the environment unfavourable for spirochaete colonisation [8–12].
Concern about the presence of the spirochaetes in breeding and multiplier herds could be ameliorated if there was an easy and reliable way to recognise strains with low virulence potential that are unlikely to cause disease if transferred to production herds. Experimental infection of pigs with such strains can give an indication of their virulence potential [4–6,13], but this procedure is expensive and complicated, and as a result is not practical for routine use. Alternatively, development of methods for recognition of attributes of the strain that would render them less likely to cause disease would be highly useful. A number of potential virulence or virulence lifestyle factors promoting colonisation have been described in B. hyodysenteriae [14,15]. These include haemolysins, phospholipases and lipooligosaccharide (virulence factors), and those associated with chemotaxis, motility, accessory factors for substrate utilization, iron binding, aerotolerance, and cell surface lipoproteins (virulence lifestyle factors). In addition a block of six genes of uncertain function on the ~36 kB plasmid have been suggested to be virulence lifestyle factors, promoting colonisation [16,17]. Strains lacking virulence lifestyle genes may be less able to colonise, or to colonise to critical numbers, whilst those lacking genes associated with tissue damage may not be able to cause typical lesions in colonised pigs.
This study compared B. hyodysenteriae isolates from three apparently healthy German multiplier herds to isolates recovered from pigs with SD in production herds in the same country. The aim was to determine whether isolates from the multiplier herds had differences that might explain their apparent lack of virulence. No consistent genomic differences were found that might account for differences in disease expression by the isolates from the three multiplier herds, except in the case of weakly haemolytic isolates from one multiplier herd that had a disruption in the promoter region for the haemolysin gene hlyA, as well as changes in the haemolysin III and haemolysin activation proteins, and in the iron metabolism genes bitB and bitC. None of the isolates from the three multiplier herds possessed all six of the block of plasmid genes, but isolates with a similar lack of plasmid genes also were found in some herds with disease, indicating that even if these genes do enhance colonisation potential they are not essential for disease production.
Materials and Methods
Source of Brachyspira hyodysenteriae isolates
This study utilised 23 B. hyodysenteriae isolates obtained from the culture collection at the University of Veterinary Medicine, Hannover. The isolates originally had been recovered from diagnostic faecal samples received from 12 German pig herds, submitted in 2014. Thirteen isolates were from three healthy multiplier herds A, B and C (n = 6, 4 and 3 isolates, respectively) and were recovered during routine screening of faecal samples from healthy pigs to confirm the ongoing high health status of the herds. These herds supplied genetically improved stock to production herds. They did not regularly use antimicrobials that could mask the presence of disease in the herds. The other ten isolates were submitted from nine production herds that had a clinical history of SD, but that were not directly linked to the multiplier herds (Table 1).
Table 1. Origin, herd disease status, sequence type, strength of haemolysis and plasmid gene (ORF) profiles of the 23 B. hyodysenteriae isolates tested.
Unexpectedly, B. hyodysenteriae was isolated from multiplier herd A in 2014 during routine health screening. As the herd supplied pigs to other herds, an eradication program was performed in which the herd was depopulated for six weeks before being repopulated from the same high-health-status herd it had used previously as a source of animals. A thorough clean up, disinfection and pest control programme was undertaken by a professional company during the depopulated period; subsequently, routine sampling indicated that the farm was free of B. hyodysenteriae. Six months later the spirochaete again was isolated from faecal samples during routine screening. Isolates from before (n = 1) and after (n = 5) the eradication attempt were available for examination. A similar apparently subclinical colonisation subsequently was found in multiplier herd B located approximately 30 kilometres from herd A, and which received additional testing because it received piglets from the same source as herd A. As well as B. hyodysenteriae, an isolate of "B. hampsonii" that subsequently was identified as genetic group III in multilocus sequence typing [18] was recovered during surveillance. Herd C was a closed herd that had not receive any live pigs for 15 years, and was located in a different region of Germany to herds A and B. None of the three herds had direct contact with each other.
Culture and identification
Diagnostic faecal samples and colon contents were cultured on selective Trypticase Soy agar (TSA) supplemented with 0.1% yeast extract, 6 μg/ml vancomycin, 6.25 μg/ml colistin, 12.5 μg/ ml rifampicin, 15.25 μg/ml spiramycin, 200 μg/ml spectinomycin, and 5% bovine blood [19], and on Columbia blood agar (CBA), all supplied by Oxoid, Wesel, Germany, and were incubated anaerobically in an AnaeroJar with an AnaeroGen generator (Oxoid) at 42°C for six days. Suspected brachyspiral growth was confirmed by phase contrast microscopy and identified to the species level using nox-RFLP as described previously [20–22]. Isolates identified as B. hyodysenteriae subsequently had their identity confirmed using a B. hyodysenteriae speciesspecific PCR [23] and nox-gene sequencing using the same primers as for nox-RFLP. Interpretation of sequencing results was based on CLSI guideline MM18-A [24]. Prior to genomic sequencing the identity of the B. hyodysenteriae isolates again was independently confirmed using a modified nox-based PCR [25].
Strength of haemolysis
The strength of beta haemolysis around brachyspiral growth on the isolation plates was recorded for all isolates. The strength of haemolysis for all isolates was tested again both on TSA with 10% bovine blood and on CBA with 5% ovine blood, where a cut was made in the agar during inoculation to enhance any haemolysis (the “ring phenomenon” test). Haemolysin production also was induced for up to two hours from resting cells using RNA-core type XI-C (Sigma-Aldrich, St. Louis, USA), as previously described [26]. Briefly, spirochaete cells in exponential growth were harvested from TSA plates after 5 days growth. The cells were washed three times with sterile phosphate buffered saline (PBS) before being resuspended in PBS supplemented with 2mM glucose, 2mM MgSO4 and 0.2% (w/v) RNA-core. Spirochaetes were counted in a haemocytometer chamber viewed with a phase contrast microscope, numbers were adjusted to approximately 1 x 106 cells per ml with the same buffer, and 2ml of cell suspension was incubated at 37°C. A sample of the cells (0.5ml) was taken before incubation and after 30, 60 and 120 minutes of incubation at 37°C. The collected samples were immediately placed on ice and the supernatant collected after centrifugation at 10,000 g for 10 minutes. Undiluted supernatants (50μl) were added to 50μl of washed sheep red blood cells (RBC) that were diluted 100-fold to approximately 5 x 107 erythrocytes per ml in a 96-well V-bottom plate. Haemolysis was allowed to occur at 37°C for one hour. B. hyodysenteriae strain WA1 was included as the strong haemolysis control and Brachyspira innocens strain B256 was used as the weak haemolysis control. A positive control for the assay comprising 50μl of sterile distilled water and a negative control comprising 50μl sterile PBS also were included. Haemolysis was visually examined, with positive haemolysis identified as the absence of settled RBC in the bottom of the well, similar to that observed for the positive control. Negative (weak) haemolysis was identified by the presence of settled RBC at the bottom of the well, comparable to results for the weakly haemolytic B. innocens control. All samples were tested in triplicate.
Whole genomic sequencing
Bacterial DNA was extracted from five-day old cultures of B. hyodysenteriae using the DNeasy Blood and Tissue Kit (Qiagen, Hilden, Germany). Genome sequences of B. hyodysenteriae strains were generated on an Illumina MiSeq using v3 chemistry and 300 base pair (bp) pairedend reads using dual indexed Nextera XT libraries. The mean insert size was around 250–300 bp and the sequencing was performed at 70x depth of coverage. MiSeq reads were paired using the inward pointing orientation typical for Illumina paired end sequencing. De novo assembly was performed using Geneious R9 (Biomatters Ltd, Auckland, New Zealand) using a high sensitivity setting with reads not trimmed prior to assembly. Assembled contiguous sequences were mapped to the genome sequence of B. hyodysenteriae strain WA1 (accession number NC_012225) using CONTIGuator [27] with a Blast E-value of 1e-20. The assembled contigs were deposited at GenBank as a whole genome sequencing (WGS) project with BioProject identification PRJNA326554.
In silico multilocus sequence typing (MLST)
MLST was conducted using the previously described scheme [28], except that for each locus the first allele sequence for the B. hyodysenteriae MLST locus was used to identify the allele sequences for each sequenced B. hyodysenteriae strain using the BlastN function of the Geneious R9 software. Allele designations for each locus then were obtained by a query search from the PubMLST website. New sequence type (ST) designations were assigned and deposited at the PubMLST site (http://pubmlst.org/brachyspira/), and existing data concerning the STs of other B. hyodysenteriae isolates was downloaded.
The MLST allele sequences for each B. hyodysenteriae strain were concatenated in the order of adh-alp-est-gdh-glpK-pgm-thi and the concatenated sequences aligned with ClustalW. Dendograms were constructed from concatenated allelic sequences using the Unweighted-Pair Group Method with Arithmetic Mean (UPGMA) method with 1000 bootstrap replicates in MEGA6 [29]. Minimum spanning trees (MST) were constructed from the data matrix of allelic mismatches using the UPGMA method with 1000 bootstrap replicates using the PHYLOViZ software [30].
Virulence and virulence lifestyle genes
Gene sequences for the putative virulence and lifestyle genes of B. hyodysenteriae strain WA1 were obtained from previous publications [14,15]. A total of 332 genes that have been associated with virulence or virulence life style factors in B. hyodysenteriae were examined in detail (S1 Table). These included the 11 genes that previously were investigated by PCR in 121 German isolates from pigs with SD [31]. These were genes for inner membrane (clpX) and outer membrane proteins (OMPs: bhlp16, bhlp17.6, bhlp29.7, bhmp39f, bhmp39h) that potentially could be involved in adhesion or interactions with host cells, haemolysins (hlyA/ACP, tlyA), iron metabolism (ftnA, bitC), and aerotolerance (nox). Additional haemolysin genes tlyB and tlyC, and those predicted to encode haemolysin III, haemolysin activation protein, haemolysin and haemolysin channel protein also were investigated [14,15], as were bitA and bitB, genes associated with iron metabolism. Other genes examined included five rfb genes associated with rhamnose biosynthesis, which presumably are involved in O-antigen production, and members of a block of six plasmid-encoded genes that recently have been described as putative virulence-associated genes in B. hyodysenteriae [17], one of which is a second copy of an rfbC gene mentioned above.
Each B. hyodysenteriae genome sequence was searched using the BlastN function of Geneious R9 software for the presence of the putative virulence-associated genes. Sequences with a maximum E-value less than 1e-50 and having greater than 80% sequence length were extracted for further analysis. Homologous gene sequences were translated in Geneious R9 and both nucleotide and deduce amino acid sequences were aligned to the B. hyodysenteriae strain WA1 homolog using the MUSCLE alignment tool from Geneious R9.
The nucleotide and translated amino acid sequences of the virulence-associated genes also were examined to determine whether there were any consistent differences amongst the isolates from herds with and without disease.
Investigation of the genomic environment around the haemolysin genes
Genomic regions directly adjacent to the coding sequence of the eight haemolysin-associated genes were examined using the MUSCLE alignment tool from the Geneious R9 software to identify potential differences in the promoter regions amongst the isolates.
Results
Spirochaete isolation
Spirochaetal growth was recorded as being sparse or occasionally moderate from faecal samples from the three multiplier herds, whereas it was heavy for all the samples recovered from the nine production herds with SD. Twenty colon samples that were examined from pigs from herd B all showed chronic lymphoplasmacytic colitis with hyperplasia of the gut-associated lymphoid tissue. Samples from one animal also showed depletion of goblet cells, and Brachyspira murdochii was isolated from this sample. A heavy growth of “B. hampsonii” was recovered from one colon sample, and heavy and sparse growths of B. hyodysenteriae were recovered from two other colon samples. Three sows from herd C were necropsied and no gross or histological findings consistent with SD were observed, although moderate or heavy growth of B. hyodysenteriae was recovered from the colons. None of the clients receiving pigs from the three multiplier herds prior to them ceasing supplying stock reported subsequent occurrence of SD.
Genome assembly
Sequencing depth of coverage of the Illumina 300 base paired end reads and assembly statistics for the 23 strains of B. hyodysenteriae is shown in S2 Table. The genome size of all strains was approximately 3 Mb (3,172,065 to 3,622,295 bp), which is similar to the B. hyodysenteriae reference strain WA1. The GC content was around 27.1%, which also is in agreement with values for the reference strain [14,15].
Mapping to reference strain WA1 genome and plasmid
The results for mapping the assembled contiguous sequences to the reference strain WA1 genome is shown in S3 Table. All strains had a genome sequence alignment coverage between 2.28% and 18.2% compared to the WA1 genome. Of the mapped sequences, the similarity to the WA1 genome ranged from 94.04% to 96.98%.
Multilocus sequence typing
The sequence types (STs) for the B. hyodysenteriae isolates are shown in Table 1, and the MLST dendrogram for these STs is shown in Fig 1. The 23 German isolates were assigned to 11 STs (Fig 1). The isolates from multiplier herds B and C were more closely related to each other than to those in the other nine STs, originating from multiplier herd A and the production herds. The isolates from multiplier herds A, B and C were of previously undescribed STs (ST132, ST133 and ST134, respectively), and all isolates from the same herd had the same ST. The isolates recovered from herd A before and after the eradication programme had the same ST (ST132), suggesting that the same strain had re-emerged. The two isolates from herd D also shared a common ST (ST138) and the single isolates from herds K and L both were of ST120, whilst all other isolates from the different herds had different STs. Three of the STs from the nine herds with SD were newly described whilst the other five had STs that previously have been described. The latter included strains from Germany (ST52, ST112, ST118 and ST120), Belgium and Italy (ST52), and the USA (ST104), recovered in the 1990s, 2000s and 2010s (Table 2). Weakly haemolytic strain D28 from Belgium belonged to a different ST (ST172) from the isolates in the current study [32].
Fig 1. MSLT dendrogram showing relationships between the 11 STs of German B. hyodysenteriae isolates from this study. The dendrogram was constructed from concatenated allelic sequences using the Unweighted-Pair Group Method with Arithmetic Mean (UPGMA) method with 1000 bootstrap replicates in MEGA6. Isolates originating from pig herds showing clinical signs of disease and the three asymptomatic pig herds are indicated. Evolutionary distances were computed using the Maximum Composite Likelihood method and are shown as the number of base substitutions per site.
Table 2. Name, origin and date of isolation for previous porcine isolates that had the same sequence type (ST) as those in this study.
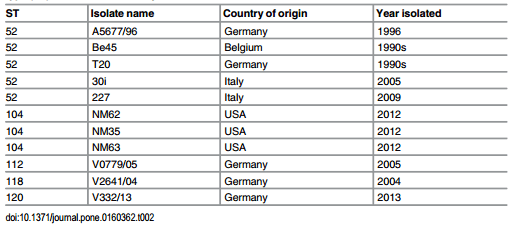
A Minimum Spanning Tree showing the relationships of the STs to each other and to previously described STs is presented as Fig 2. Although the isolates from herds A, B and C belonged to newly described STs, they were closely related to other STs (see http://pubmlst.org/ brachyspira/). For example, the ST of isolates from herd A (ST132) shared four MLST alleles with ST23 containing an Australian isolate from the 1980s, and four with ST53 containing a USA isolate from the 1970s; the ST of isolates from herd B (ST133) shared four alleles with ST87 containing an Italian isolate from 2006, but also shared three alleles with ST52 containing isolates from Germany, Belgium, Italy and Austria isolated between 1996 and 2009; the ST of isolates from herd C (ST134) shared three alleles with ST112 and with ST120 which included German isolates recovered in 2005 (ST112) and 2013 (ST120), and current isolates from herds G, and K and L respectively.
Isolates and haemolysis
The isolates from herd C were all weakly haemolytic, whilst all other isolates were strongly haemolytic. When resting cells were induced in the presence of RNA-core, haemolytic activity was detected in the supernatant of the strongly haemolytic isolates after 30 minutes incubation whereas no haemolytic activity was detected in the supernatant of the weakly haemolytic isolates, even after 120 minutes incubation.
Presence of genes encoding virulence and virulence lifestyle associated factors
In all cases isolates sharing an ST had the same virulence gene profiles and could be regarded as representing a single strain. Consequently the strain name used is that of the first isolate recovered from each herd (eg JR11 for herd C). Of the 332 genes investigated, 12 (3.8%) were absent in one or more isolates. The first six were membrane protein genes: bhlp16 (which was absent from isolates with STs 133, 134, 112 and 137 from herds B, C, G and H respectively), bhlp17.6 (which was only found in the isolate in ST137 from herd H), bhmp39e and bhmp39f (both genes absent from isolates with STs 133, 134 and 137 in herds B, C and H respectively), putative cysteine peptidase BHWA1_RS01825 (absent from isolates with STs 132, 120, 138, 118 and 137 in herds A, C, D, E, H and K respectively), and putative inner membrane protein BHWA1_RS02825 (absent from isolates with STs 132, 133, 134, 120, 138, 152 and 136 in herds A, B, C, D, I, J and K respectively).
Fig 2. Minimum Spanning Tree showing relationships of the B. hyodysenteriae isolates from this study (marked with an asterisk) and 349 previously described isolates that were obtained from PubMLST. The isolates shown originated from Australia, Austria, Belgium, Brazil, Canada, Germany, Italy, Japan, Korea, Serbia, Sweden, the UK and the USA. In the MST, each node represents a different ST (labelled) and the colour represents the country of origin. The size of the node indicates the number of strains having the same ST. The STs containing the isolates from the current study are indicated by an asterisk, which are coloured white for the new STs and yellow for previously described STs. The STs of the weakly haemolytic isolates from herd C in the current study (ST134) and of weakly haemolytic strain D28 from a previous study (ST172) [32] are shown with arrows.
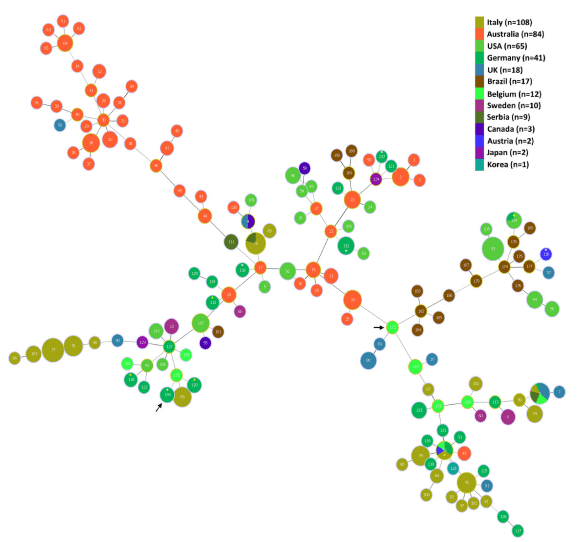
In addition there were differences between isolates in members of the block of six plasmid genes (ORFs 11–16; Table 1). Isolates with STs 138, 118 and 104 from herds D, E and F (all with SD) had all six plasmid genes present; isolates with ST132 (no disease) and ST112 (with SD) lacked two genes (ORFs 11 and 12); isolates with STs 133 and 134 (no disease), and STs 137 and 52 (with SD) only had one of the genes present (ORF15); and isolates with STs 136 and 120 (with SD) had none of the six genes.
Sequence similarity amongst the genes studied
Generally the between-strain comparisons of the sequences of the genes and their translated amino acid sequences showed that these were highly conserved, having greater than 90% similarity at the nucleotide level and greater than 92.1% similarity at the amino acid level.
Sequences of bitB and bitC. The predicted bitB and bitC sequences from the strains from herds C and I both had a 9-nucleotide insertion after position 90 which resulted in three amino acids inserted after residue 26 of the translated protein sequence. There also was a high degree of variation at positions 4 to 32 and positions 93 to 120 of the translated protein sequences. The bitB and bitC proteins both had 11 of 29 (37.9%) amino acid substitutions between positions 4 and 32, and 13 of 28 (46.4%) substitutions between positions 93 and 120. Outside these regions of variation the nucleotide and amino acid sequences of the two genes were well conserved. The bitA sequence was almost identical between strains, except in the region between base 104 and 392 where the similarity was 73% (211 out of 289 nucleotides). When translated the protein was 65.6% (63 out of 96 nucleotides) similar.
Sequences of haemolysis-associated genes. A summary of nucleotide and predicted amino acid substitutions in the eight haemolysis-associated genes from the weakly haemolytic strain J11 from herd C compared to strongly haemolytic reference strain WA1, and to weakly haemolytic B. hyodysenteriae strain D28 isolated from pigs in Belgium, as described by Mahu et al [32], is shown in Table 3. The greatest difference between strain J11 and WA1 occurred in the haemolysin III protein (10 amino acid substitutions), with the haemolysin activation protein having five substitutions. D28 had five different amino acid substitutions in the haemolysin III protein, and five amino acid substitutions in the haemolysin activation protein that were identical to those in strain J11. It is important to note that in the paper of Mahu et al [32] the labelling for haemolysin III and haemolysin activation protein in their Tables 2 and 3 and in their text appear to have been inadvertently transposed.
Promoter regions. Analysis of the nucleotide sequences around all eight genes linked to haemolytic activity identified a five-nucleotide insertion in the -10 promoter element for the hlyA gene in the weakly haemolytic strain J11 from herd C (Fig 3). There was a nucleotide substitution (C to T) 28 bp upstream from the ATG start position, a single base insertion (T) 29 bp upstream from the start position, a substitution (A to G) 45 bp upstream and a seven base deletion starting 66 bp upstream. No changes in the promoter sites for the other genes were identified.
Discussion
The first important finding in this study was the confirmation that each of the three multiplier herds was colonised by strains of B. hyodysenteriae that were not genetically atypical of the species (Fig 2). The second was that each of the multiplier herds had its own new strain of B. hyodysenteriae, and consequently that they were unlikely to have been infected from a common source. This was particularly important for herds A and B as they both received piglets from the same breeding herd. As the isolates from herd A that were recovered before and after the eradication program shared the same ST and virulence gene profile, the most likely explanation is that this is a single strain that persisted in the farm environment following the destocking and cleaning procedure.
Table 3. Nucleotide and amino acid differences for eight haemolysis-associated genes between strongly haemolytic B. hyodysenteriae reference strain WA1, weakly haemolytic strain J11 from herd C, and previously described weakly haemolytic strain D28 [32].
It was unclear how long the subclinical colonisation had been present in the multiplier herds, but as they were found almost by chance they may have been present for some time— and this leads to an implication that other apparently healthy multiplier herds potentially could be similarly colonised, but remain unidentified. The herds were attended by expert veterinary consultants and had a routine of submitting normal faecal samples for examination every six months, as well as samples from grower and finisher pigs with diarrhoea. Unfortunately this screening regimen apparently is not sufficiently sensitive for detection, as initially only herd A was identified as being colonised through this route. A scenario of subclinical colonisation could have major consequences for the spread of infection through the German pig industry. Indeed, the problem is not limited to Germany as recently an infected Swiss multiplier herd was identified [33], as have similar apparently healthy herds in Australia [7,34]. The latter infected herds initially were detected through serological screening, with confirmation of colonisation being obtained by culturing the colonic walls of apparently healthy pigs at the abattoir. It seems likely that the problem of unrecognised colonisation also may occur in other pig rearing countries, but may not have been investigated adequately.
Fig 3. Position of the five-nucleotide insertion found in the promoter site of hlyA in the weakly haemolytic strain J11 from herd C. The ribosome binding site (RBS) and Pribnow box (-10 element) are indicated. Also note the seven base deletion further upstream in the weakly haemolytic isolates.
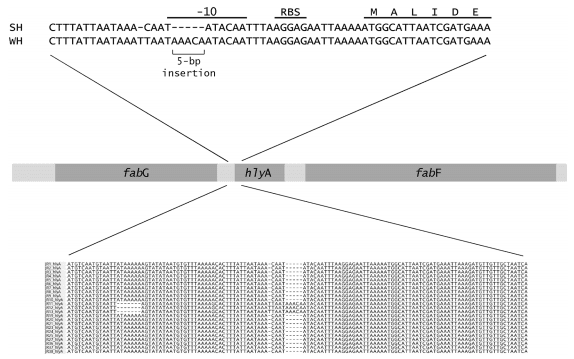
A potential explanation as to why the strains in the multiplier herds were not causing disease is that they were of reduced virulence compared to typical B. hyodysenteriae isolates, being either less able to colonise to numbers great enough to cause disease and/or be detected, and/or lacking virulence factors able to induce typical lesions [4,6,13,35]. Given that different strains (STs) were identified in the three multiplier herds, intuitively such a lack of virulence is less likely to be the case for all three herds. However, in support of a possible reduced colonisation potential was the finding that in all three herds only relatively low numbers of spirochaetes were recovered from the faeces, whereas high numbers were recovered from the production herds with disease. On the other hand spirochaete numbers in the colon tended to be higher than in the faeces. In relation to an ability to produce lesions, in herd B the diagnostic colonic samples that were examined displayed chronic lymphoplasmic colitis, which is not consistent with acute SD where pigs typically display mucohaemorrhagic colitis [1]. B. murdochii and “B. hampsonii” also were isolated from herd B, and potentially persistent colonisation by members of these species could contribute to a chronic lymphoplasmic colitis. In contrast, lesions were not found in three sows from herd C despite them having a heavy growth of B. hyodysenteriae in their colons. These findings are consistent with the isolates from these two herds potentially having different but relatively reduced virulence potential compared to isolates from the production herds. Strain J11 from herd C seemed to be particularly innocuous.
The isolates from the nine herds with SD belonged to three newly identified STs and to five that previously have been recorded in European countries or the USA (Table 2). Consequently these isolates also could be considered as being typical of those in circulation in herds with SD in Europe, and so should represent a good set to compare to the isolates from the three multiplier herds where disease was not seen. This comparison involved genes that previously have been associated with factors that may influence the ability to colonise (virulence life style genes) and those thought to encode factors that generate tissue damage (virulence genes) [31]. As it has proved very difficult to genetically manipulate B. hyodysenteriae, most of these associations between genes and their function have not been tested experimentally, and represent assumptions. Furthermore there are many genes of unknown function in the genome, and some of these also are likely to be important for disease production [14,15].
In a previous genomic analysis of 20 strains of B. hyodysenteriae substantial conservation was found in the presence and sequence of virulence-associated genes [15], and the same relative conservation was found amongst the isolates examined here.
Various studies have shown that lipooligosaccharide (LOS) extracted from B. hyodysenteriae has a variety of effects that can induce local inflammation and tissue damage that may contribute to the lesions of SD [36,37]; however, in this study there were no consistent differences between the German isolates in the number or distribution of rfb genes or others associated with LOS production. Previously B. hyodysenteriae isolates from pigs, ducks and rodents in Sweden also were shown to have conserved rfb genes [38]. Other attributes such as motility and chemotaxis are extremely important for the spirochaetes to allow them to colonise the large intestine [39,40], but again the genes associated with these processes were highly conserved in the strains investigated. The bitABC gene cluster potentially can influence the ability of the spirochaetes to bind iron, and hence alter their capacity to survive in the colon, but again these were conserved apart from in the isolates from herd C and isolate JR19 from herd I (with SD) which had insertions resulting in changes in their sequence. Unfortunately there is no specific information around the predicted active sites/binding sites of these proteins [41], so it is difficult to determine how important these changes are likely to be in relation to gene function.
The only substantial differences in distribution of putative virulence gene across the full range of isolates were found for some of the membrane proteins and lipoproteins, and in the block of six plasmid genes; however, no clear associations between these gene profiles and virulence phenotype were noted. These findings indicate that most of the previously described virulence-associated genes form part of the core genome of the species.
Differences in the presence of genes encoding outer membrane proteins in the various isolates may result from selection pressure exerted by the immune system. Compared to the profiles previously reported for other German isolates, a notable difference was that bhlp29.7 was present in all isolates in the current work, whilst it was only amplified by PCR in around half the isolates in the previous study [31]. This has some practical implications because Bhlp29.7 (formerly BmpB) has been proposed for use as an antigen for a serological ELISA for detection of evidence of B. hyodysenteriae infection [42], and conservation and distribution of the protein across all strains is important for its use in detecting infected herds.
The greater variation in the block of plasmid genes between isolates compared to that found in most other genes was interesting, and may suggest that their conservation and function is less critical for spirochaete survival than other potential virulence lifestyle genes. The three STs with isolates possessing the full block of six plasmid genes came from herds with SD, and this is consistent with these genes potentially being involved in enhancing colonisation capacity. On the other hand, the isolates in two STs lacking all these genes also came from herds with SD. In the case of the latter herds it is possible that other isolates with a full set of plasmid genes also were present in lower numbers, but were not isolated and tested, or that there were other predisposing conditions in these herds that overcame any disadvantage arising from an absence of plasmid genes in these isolates, in terms of their capacity to colonise. The isolates from the three asymptomatic multiplier herds did lack members of the block of plasmid genes, but this was only ORF11 and ORF12 (encoding two of the three Radical SAM domain proteins) for isolates from herd A, whilst the isolates from herds B and C lacked all but ORF15 (encoding NAD dependant epimerase). To date the exact role of the individual plasmid genes is unknown, and any sub-classifications based on the number and identification of the absent genes and their functions have not been investigated experimentally. Overall, this study suggests that an absence of plasmid genes cannot be used to predict that a given strain of B. hyodysenteriae will not cause disease, even though strains lacking these genes may be less likely to produce disease.
Of the virulence factors, the haemolytic activity of B. hyodysenteriae is believed to be particularly important for lesion production, and eight genes encoding proteins predicted to be involved in haemolytic activity have been described [14,15,43,44]. The number and the sequences of all these genes generally were well conserved across the isolates in the current study. In relation to the weakly haemolytic strain J11 from herd C, the haemolysin III gene and protein were the most dissimilar from those of WA1 (Table 3), having10 amino acid substitutions that were predicted to occur in both transmembrane and topological domain regions of the channel-forming protein, based of the structure of yqfA in Escherichia coli. In comparison weakly haemolytic strain D28 from Belgian pigs [32] only had five substitutions in this protein, although potentially they also could influence function. Strain J11 from herd C also had five amino acid substitutions in the haemolysin activation protein, and it was very interesting that the same substitutions occurred in this protein in D28, even though the two strains were not otherwise closely related genetically. Other changes in haemolysin-associated genes in the strain from herd C were relatively minor, but included three amino acid substitutions in the haemolysin gene (not examined by Mahu et al [32]). Potentially any of these changes could affect the function of the proteins and might influence haemolytic activity, which in turn is likely to be linked to virulence. Unfortunately there have been no detailed functional/structural studies of the haemolysis-associated genes in B. hyodysenteriae, so it is difficult to predict how an amino acid change might influence function.
Besides gene distribution and sequence, the control of gene expression is likely to be critical in influencing the phenotype of the strains. This was seen in the case of hlyA, where although the gene was present in all strains examined, there was a disruption to the promoter region of hlyA in the strain from herd C (Fig 3). Induction of haemolytic activity in the presence of RNA-core has been associated with the production of hlyA [44], and this induction failed to occur with the weakly haemolytic strain. Haemolytic activity, particularly associated with hlyA, is considered integral to the pathogenesis of SD by causing disruptions to the colonic epithelium [44], and hence the disruption to the gene may be useful as a predictive marker for lack of virulence. This same strain from herd C also had substitutions in haemolysin III and haemolysin activation proteins that could influence its haemolytic properties, and it also had insertions in bitB and bitC, which potentially could affect iron binding. To further investigate the functional significance of these and other such genetic changes it will be important to undertake transcriptomic and proteomic analyses, to investigate gene function by using targeted gene mutagenesis and complementation experiments, and to undertake in vitro functional assays for iron binding, as well as other assays for chemotaxis, motility, and haemolysis. Cell or tissue cultures potentially also could be used to look for variation in cellular responses to different isolates [45,46].
Overall it seems likely that strain J11 from herd C (ST134) had a reduced virulence potential, since it was weakly haemolytic, had insertions in bitB and bitC, and lacked five of the block of six plasmid genes. Furthermore colonised sows did not show any colonic lesions. Ultimately it would be necessary to undertake experimental infection of pigs with such strains under controlled conditions to confirm their true virulence potential [4–6,13,35]. This process requires specialised facilities, and it is expensive, time consuming and difficult; furthermore, it is unlikely to provide a definitive result in all cases as the expression of virulence by different strains is likely to be relative rather than absolute. To demonstrate a complete lack of virulence it is necessary to optimise the infection protocol and to include control strains that have been identified as being highly virulent and of reduced virulence, respectively. Other evidence for a lack of virulence of the strains from the multiplier herds in this and other studies would be the identification of the same strain(s) in other healthy herds that received pigs from these herds. This was not possible in this case as they all stopped supplying pigs to other herds once they were found to be carrying B. hyodysenteriae, and none of the herds that previously had received pigs reported developing SD.
If isolates from some or all of the multiplier herds from the current study were shown to cause disease in experimentally infected pigs, the question would arise as to why did pigs in these herds remain healthy? Previously an isolate of B. hyodysenteriae that was recovered from a healthy multiplier herd in Australia was shown to induce SD when used to experimentally infect pigs, and it was assumed that this might reflect differences in the gastrointestinal ecology in pigs from different sources [5]. It is known that under experimental conditions the expression of SD can be influenced by dietary ingredients that are fed to pigs: these different substrates have been shown to influence the microbiota and/or the physicochemical environment in the colon (eg viscosity, hydration, pH), which in turn may influence the ability of B. hyodysenteriae to colonise [8–12,47]. In the current study the pigs in the various herds were all fed standard commercial diets, so diet is not an obvious explanation for any differences in disease occurrence. Another difference is that in multiplier and breeding herds there is likely to be less crowding, stress and concurrent disease compared to production herds, due to their superior management practices. It is known that the hormone norepinephrine is released into the intestinal tract in times of stress, and under experimental in vitro conditions norepinephrine has been shown to increase proliferation and attachment to cell monolayers by the related spirochaete Brachyspira pilosicoli [48]: it seems likely that the same may apply to B. hyodysenteriae. The likelihood of such circumstances occurring in the multiplier herds is supported by the finding of an isolate of “B. hampsonii” in herd B, suggesting that it too may not have had an optimised colonic environment to allow it to induce disease, even though there was a heavy growth in the colon. In the case of the B. hyodysenteriae isolates, the lack of members of the block of six plasmid genes also may have reduced their colonisation fitness, which together with a lack of other predisposing factors in the multiplier herds could have resulted in a level of colonisation that was insufficient to induce signs of disease.
Unfortunately breeding and multiplier herds with pigs that do not show signs of disease but that harbour strains of B. hyodysenteriae are placed in a difficult position when it comes to selling stock. In the case of the three herds described here they ceased selling live pigs. In the current work it was not possible to definitively use the genotype to identify strains that had reduced virulence, but strain J11 in herd C had significant differences with respect to having weak haemolysis, and potentially diminished iron binding and lack of plasmid genes that suggest a reduced potential to cause disease. Strains with this sort of profile could be regarded as presenting a low risk of causing disease, although further work is required to confirm this. It is important to note that strains that lack some or all of the block of six plasmid genes still may be able to cause disease under conditions of poor husbandry, stress or dietary changes that can enhance colonisation and predispose to disease development.
Supporting Information
S1 Table. Identity of the 332 virulence-associated genes investigated for their distribution in the German B. hyodysenteriae isolates. (DOCX)
S2 Table. Quality statistics for mapping the 23 strains of B. hyodysenteriae with the reference strain WA1. (DOCX)
S3 Table. Sequence information and assembly statistics for the 23 sequenced B. hyodysenteriae strains. (DOCX)
Acknowledgments
The authors wish to acknowledge the veterinarians and pig producers who submitted the diagnostic samples, and who agreed to this analysis being published.
This article was published in PLoS ONE 11(8): e0160362. doi:10.1371/ journal.pone.0160362. This is an Open Access article distributed under the terms of the Creative Commons Attribution License.
References
1. Hampson DJ. Brachyspiral colitis. In: Zimmerman JJ, Karriker LA, Ramirez A, Schwartz KJ, Stevenson GW, (editors). Diseases of swine. Chichester: Wiley-Blackwell; 2012. p. 680–96.
2. Mushtaq M, Zubair S, Råsbäck T, Bongcam-Rudloff E, Jansson DS. Brachyspira suanatina sp. nov., an enteropathogenic intestinal spirochaete isolated from pigs and mallards: genomic and phenotypic characteristics. BMC Microbiol. 2015; 15(1):208.
3. Chander Y, Primus A, Oliveira S, Gebhart CJ. Phenotypic and molecular characterization of a novel strongly hemolytic Brachyspira species, provisionally designated “Brachyspira hampsonii”. J Vet Diagn Invest. 2012; 24(5):903–10. doi: 10.1177/1040638712456975 PMID: 22914820
4. Lysons RJ, Lemcke RM, Bew J, Burrows MR, Alexander TJL. 1982. An avirulent strain of Treponema hyodysenteriae isolated from herds free of swine dysentery. In: Proceedings of the 7th International Pig Veterinary Society Congress; 1982; Mexico City, Mexico. p. 40.
5. Hampson DJ, Cutler R, Lee BJ. Virulent Serpulina hyodysenteriae from a pig in a herd free of clinical swine dysentery. Vet Rec. 1992; 131(14):318–9. PMID: 1441137
6. Thomson JR, Murray BP, Henderson LE, Moore L, Meikle CS. Virulence studies in porcine Brachyspira species by experimental challenge of pigs. In: Proceedings of the Second International Conference on Colonic Spirochaetal Infections in Animals and Humans; 2003; Eddleston, Scotland. abstract 10.
7. Hampson DJ, La T, Phillips ND. Emergence of Brachyspira species and strains: reinforcing the need for surveillance. Porc Health Manag. 2015; 1:8.
8. Siba PM, Pethick DW, Hampson DJ. Pigs experimentally infected with Serpulina hyodysenteriae can be protected from developing swine dysentery by feeding them a highly digestible diet. Epidemiol Infect. 1996; 116(2):207–16. PMID: 8620913
9. Pluske JR, Siba PM, Pethick DW, Durmic Z, Mullan BP, Hampson DJ. The incidence of swine dysentery in pigs can be reduced by feeding diets that limit the amount of fermentable substrate entering the large intestine. J Nutr. 1996; 116(2):2920–33.
10. Thomsen LE, Knudsen KE, Jensen TK, Christensen AS, Møller K, Roepstorff A. The effect of fermentable carbohydrates on experimental swine dysentery and whip worm infections in pigs. Vet Microbiol. 2007; 119(2–4):152–63. PMID: 17049759
11. Hansen CF, Phillips ND, La T, Hernandez A, Mansfield J, Kim JC, et al. Diets containing inulin but not lupins help to prevent swine dysentery in experimentally infected pigs. J Anim Sci. 2010; 88(10):3327– 36. doi: 10.2527/jas.2009-2719 PMID: 20525927
12. Hansen CF, Hernandez A, Mansfield J, Hildago A, La T, Phillips ND, et al. A high dietary concentration of inulin is necessary to reduce the incidence of swine dysentery in pigs experimentally challenged with Brachyspira hyodysenteriae. Br J Nutr. 2011; 106(10):1506–13. doi: 10.1017/S000711451100208X PMID: 21736788
13. Achacha M, Messier S, Mittal KR. Development of an experimental model allowing discrimination between virulent and avirulent isolates of Serpulina (Treponema) hyodysenteriae. Can J Vet Res. 1996; 60(1):45–9. PMID: 8825993
14. Bellgard MI, Wanchanthuek P, La T, Ryan K, Moolhuijzen P, Albertyn Z, et al. Genome sequence of the pathogenic intestinal spirochete Brachyspira hyodysenteriae reveals adaptations to its lifestyle in the porcine large intestine. PLoS One. 2009; 4(3):e4641. doi: 10.1371/journal.pone.0004641 PMID: 19262690
15. Black M, Moolhuijzen P, Barrero R, La T, Phillips ND, Hampson DJ, et al. Analysis of multiple Brachyspira hyodysenteriae genomes confirms that the species is relatively conserved but has potentially important strain variation. PLoS One. 2015; 10(6):e0131050. doi: 10.1371/journal.pone.0131050 PMID: 26098837
16. La T, Phillips ND, Wanchanthuek P, Bellgard MI, O’Hara AJ, Hampson DJ. Evidence that the 36kb plasmid of Brachyspira hyodysenteriae contributes to virulence. Vet Microbiol. 2011; 153(1–2):150–5. doi: 10.1016/j.vetmic.2011.02.053 PMID: 21458173
17. La T, Phillips ND, Thomson JR, Hampson DJ. Absence of a set of plasmid-encoded genes is predictive of reduced pathogenic potential in Brachyspira hyodysenteriae. Vet Res. 2014; 45:131. doi: 10.1186/ s13567-014-0131-6 PMID: 25512147
18. Mirjakar NS, Bekele AZ, Chander YY, Gebhart CJ. Molecular epidemiology of novel pathogen “Brachyspira hampsonii” reveals relationships between diverse genetic groups, regions, host species, and other pathogenic and commensal Brachyspira Species. J Clin Microbiol. 2015; 53(9):2908–18. doi: 10. 1128/JCM.01236-15 PMID: 26135863
19. Dünser M, Schweighart H, Pangerl R, Awad-Masalmeh M, Schuh M. Swine dysentery and spirochaetal diarrhea—investigations into enteritis caused by Serpulina species. Wiener Tierärztl Monatsschr. 1997; 84:151–61.
20. Rohde J, Rothkamp A, Gerlach GF. Differentiation of porcine Brachyspira species by a novel nox PCRbased restriction fragment length polymorphism analysis. J Clin Microbiol. 2002; 40(7):2598–600. PMID: 12089283
21. Rohde J, Habighorst-Blome K. An up-date on the differentiation of Brachyspira species from pigs with nox-PCR-based restriction fragment length polymorphism. Vet Microbiol. 2012; 158(1–2):211–5. doi: 10.1016/j.vetmic.2012.02.008 PMID: 22386675
22. Rohde J, Habighorst-Blome K. Corrigendum to “An up-date on the differentiation of Brachyspira species from pigs with nox-PCR-based restriction fragment length polymorphism” [Vet Microbiol. 2012; 158:211–5] Vet Microbiol. 2013; 162(1):303.
23. Atyeo RF, Stanton TB, Jensen NS, Suriyaarachichia DS, Hampson DJ. Differentiation of Serpulina species by NADH oxidase gene (nox) sequence comparisons and nox-based polymerase chain reaction tests. Vet Microbiol. 1999; 67(1):47–60. PMID: 10392777
24. CLSI. Interpretive Criteria for Identification of Bacteria and Fungi by DNA Target Sequencing; Approved Guideline. CLSI document MM18-A. Wayne, PA: Clinical and Laboratory Standards Institute; 2008.
25. La T, Phillips ND, Hampson DJ. Development of a duplex PCR assay for detection of Brachyspira hyodysenteriae and Brachyspira pilosicoli in pig feces. J Clin Microbiol. 2003; 41(7):3372–5. PMID: 12843096
26. Lemcke RM, Burrows MR. Studies on a haemolysin produced by Treponema hyodysenteriae. J Med Microbiol. 1982; 15(2):205–14. PMID: 6754943
27. Galardini M, Biondi EG, Bazzicalupo M, Mengoni A. CONTIGuator: a bacterial genomes finishing tool for structural insights on draft genomes. Source Code Biol Med. 2011; 6(11) doi: 10.1186/1751-0473- 6-11
28. La T, Phillips ND, Harland BL, Wanchanthuek P, Bellgard MI, Hampson DJ. Multilocus sequence typing as a tool for studying the molecular epidemiology and population structure of Brachyspira hyodysenteriae. Vet Microbiol. 2009; 138(3–4):330–8. doi: 10.1016/j.vetmic.2009.03.025 PMID: 19369014
29. Tamura K, Stecher G, Peterson D, Filipski A, Kumar S. MEGA6: Molecular Evolutionary Genetics Analysis Version 6.0. Mol Biol Evol. 2013; 30:2725–9. doi: 10.1093/molbev/mst197 PMID: 24132122
30. Francisco AP, Vaz C, Monteiro PT, Melo-Cristino J, Ramirez M, Carriço JA. PHYLOViZ: phylogenetic inference and data visualization for sequence based typing methods. BMC Bioinform. 2012; 13:87.
31. Barth S, Gömmel M, Baljer G, Herbst W. Demonstration of genes encoding virulence and virulence lifestyle factors in Brachyspira spp. isolates from pigs. Vet Microbiol. 2012; 155(2–4):438–43. doi: 10. 1016/j.vetmic.2011.09.032 PMID: 22047713
32. Mahu M, De Pauw N, Vande Maele L, Verlinden M, Boyen F, Ducatelle R, et al. Variation in hemolytic activity of Brachyspira hyodysenteriae strains from pigs. Vet Res. 2016; 47:66. doi: 10.1186/s13567- 016-0353-x PMID: 27338265
33. Löbert S, Zimmermann W, Bürki S, Frey J, Nathues H, Scheer P, et al. Occurrence of Brachyspira hyodysenteriae in multiplier pig herds in Switzerland. Tierärztl Prax. 2016; 44:13–8.
34. Hampson DJ, La T, Phillips ND, Holyoake PK. Brachyspira hyodysenteriae isolated from apparently healthy pig herds following an evaluation of a prototype commercial serological ELISA. Vet Microbiol. 2016; 191:15–9. doi: 10.1016/j.vetmic.2016.05.016 PMID: 27374902
35. Jensen NS, Stanton TB. Comparison of Serpulina hyodysenteriae B78, the type strain of the species, with other S. hyodysenteriae strains using enteropathogenicity studies and restriction fragment length polymorphism analysis. Vet Microbiol. 1993; 36(3–4):221–31. PMID: 7903832
36. Nuessen ME, Joens LA, Glock RD. Involvement of lipopolysaccharide in the pathogenicity of Treponema hyodysenteriae. J Immunol. 1983; 131(2):997–9. PMID: 6863941
37. Nibbelink SK, Sacco RE, Wannemuehler MJ. Pathogenicity of Serpulina hyodysenteriae: in vivo induction of tumor necrosis factor and interleukin-6 by a serpulinal butanol/water extract (endotoxin). Microb Pathog. 1997; 23(3):181–7. PMID: 9281475
38. Backhans A, Jansson DS, Aspán A, Fellström C. Typing of Brachyspira spp. from rodents, pigs and chickens on Swedish farms. Vet Microbiol. 2011; 153(1–2):156–62. doi: 10.1016/j.vetmic.2011.03.023 PMID: 21497461
39. Kennedy MJ, Yancey RJ Jr. Motility and chemotaxis in Serpulina hyodysenteriae. Vet Microbiol. 1996; 49(1–2):21–30. PMID: 8861640
40. Naresh R, Hampson DJ. Attraction of Brachyspira pilosicoli to mucin. Microbiol. 2010; 156(Pt 1):191– 7.
41. Dugourd D, Martin C, Rioux CR, Jacques M, Harel J. Characterization of a periplasmic ATP-binding cassette iron import system of Brachyspira (Serpulina) hyodysenteriae. J Bacteriol. 1999; 181 (22):6948–57. PMID: 10559160
42. La T, Phillips ND, Hampson DJ. Evaluation of recombinant Bhlp29.7 as an ELISA antigen for detecting pig herds with swine dysentery. Vet Microbiol. 2009; 133(1–2):98–104. doi: 10.1016/j.vetmic.2008.06. 003 PMID: 18619744
43. ter Huurne AA, Muir S, van Houten M, van der Zeijst BA, Gaastra W, Kusters JG. Characterization of three putative Serpulina hyodysenteriae hemolysins. Microb Pathog. 1994; 16(4):269–82. PMID: 7968456
44. Hsu T, Hutto DL, Minion FC, Zuerner RL, Wannemuehler MJ. Cloning of a beta-hemolysin gene of Brachyspira (Serpulina) hyodysenteriae and its expression in Escherichia coli. Infect Immun. 2001; 69(2):706–11. PMID: 11159958
45. Gömmel M, Barth S, Heydel C, Baljer G, Herbst W. Adherence of Brachyspira hyodysenteriae to porcine intestinal epithelial cells is inhibited by antibodies against outer membrane proteins. Curr Microbiol. 2013; 66(3):286–92. doi: 10.1007/s00284-012-0267-4 PMID: 23192304
46. Naresh R, Song Y, Hampson DJ. The intestinal spirochete Brachyspira pilosicoli attaches to cultured Caco-2 cells and induces pathological changes. PLoS One. 2009; 4(12):e8352. doi: 10.1371/journal. pone.0008352 PMID: 20020053
47. Durmic Z, Pethick DW, Pluske JR, Hampson DJ. Changes in bacterial populations in the colon of pigs fed different sources of dietary fibre, and the development of swine dysentery after experimental challenge. J Appl Microbiol. 1998; 85(3):574–82. PMID: 9750287
48. Naresh R and Hampson DJ. Exposure to norepinephrine enhances Brachyspira pilosicoli growth, attraction to mucin and attachment to Caco-2 cells. Microbiol. 2011; 157(Pt 2):543–7.