Introduction
Mycotoxins are a group of structurally diverse secondary fungal metabolites that occur as grain contaminants. They can cause serious problems in livestock resulting in substantial economic losses (Huwig et al. 2001). Two of the most common mycotoxins found in animal feed, aflatoxin B1 (AFB1) and zearalenone (ZEA), cause food- and feed-borne intoxications called mycotoxicoses (CAST 2003). Aflatoxin B1 is a carcinogenic metabolite produced primarily by Aspergillus flavus and A. parasiticus (IARC 2002). It is a potent liver toxin that can either be lethal when consumed at large doses or induce cancer after chronic exposure. (Khanafari et al. 2007). ZEA, produced by a number of Fusarium species mainly by F. graminearum, binds to oestrogen receptors producing functional and morphological alterations in reproductive systems. Among farm animals, pigs are most sensitive to ZEA intake, being reported clinical signs such as ovarian atrophy, prolonged oestrus intervals, persistent corpora lutea, decreased fertility and stillbirth (Fink-Gremmels and Malekinejad 2007).
Physical, chemical, physicochemical and biological approaches have been developed to reduce the impact of mycotoxins. One of the most efficient prevention strategies to prevent mycotoxicoses is the dietary supplementation with materials that reduce the toxin bioavailability in the digestive tract and, therefore, their adverse effects on animals. Basic ingredients and dietary supplements such as the yeast Saccharomyces cerevisiae may have functional properties in the diet and show satisfactory results when added to feedstuff either as active cells or as cell wall components (Shetty and Jespersen 2006). a-D-mannan and b-D-glucan are the two major polysaccharides present in S. cerevisiae. They constitute up to 90% of the cell wall dry weight and have remarkable properties to interact with the host immune system and constitute a good source of adsorbent (Shetty and Jespersen 2006; Kogan and Kocher 2007). Besides its excellent nutritional value, yeasts produce a high quantity of biomass that is used in a large variety of industrial processes. The ability of the S. cerevisiae cell wall to bind ZEA has been reported recently (Yianninkouris et al. 2003, 2004a,b). These authors tested different mathematical models to describe the ability of S. cerevisiae cell wall to adsorb ZEA. However, there is little information on the influence of the corn on the adsorption levels of AFB1 and ZEA by yeast cell walls (YCWs). The aim of the present study was to evaluate the influence of the corn on the adsorption levels of AFB1 and ZEA by YCWs.
Materials and methods
Yeast cell walls suspensions, corn and reagents
Two commercial YCWs were studied for toxins’ binding ability: YCW1 and YCW2. Their compositions are described in Table 1. Each YCW was resuspended in buffer solution at pH 2 (50 ml of potassium chloride 02 mol l1 and 13 ml of hydrochloric acid 02 mol l1 brought to 250 ml with distilled water) and pH 6 (100 ml of potassium phosphate bi acid 01 mol l1 and 112 ml of sodium hydroxide 01 mol l1 brought to 250 ml with distilled water) for the subsequent uses. The pH was adjusted by adding hydrochloric acid of 02 mol l1 or sodium hydroxide of 01 mol l1 using a pH meter (model 250A; Orion Research Inc., Boston, MA, USA).
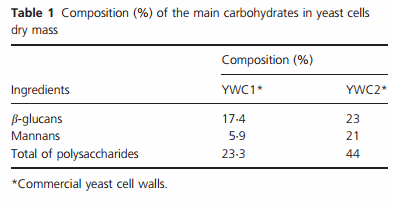
Corn, ground and sieved, was utilized to study the matrix influence on toxin–adsorbent interactions. The extraction and detection of AFB1 and ZEA corn samples in this test to discard the presence of the same in this substrate were performed. The AFB1 and ZEA levels were below the detection limit. The methodology for the detection and quantification of these mycotoxins was performed according to the proposed Trucksess et al. (1994) and Cervero et al. (2007). The detection limits of the used method were 04 and 3 ng g1 for AFB1 and ZEA, respectively.
Adsorption test
Different concentrations of each adsorbent were tested to obtain an adequate relation between adsorbent and toxin. Seven (7) suspensions of each YCW (2, 5, 10, 25, 50, 100 and 500 lg ml1 ) were tested against AFB1 (2 lg ml1 ), and five (5) suspensions of each YCW (10, 50, 75, 100 and 200 lg ml1 ) were used for ZEA (1 lg ml1 ) adsorption.
An aliquot of 500 ll of the corresponding toxin (2 lg ml1 AFB1 or 1 lg ml1 ZEA) was added to each microtube containing 500 ll of each YCW suspension. The microtubes were introduced into a shaker at 37°C with mechanical agitation for 30 min. Microtubes were then centrifuged for 10 min at 16 873 g in a Labor 2K15 centrifuge (Sigma Aldrich Chemie GmbH, Taufkirchen, Germany), and the supernatant was taken and evaporated to dryness under gentle stream of nitrogen gas. Each adsorption test was performed in duplicate, and control tests were performed. The extracts were quantified by high-pressure liquid chromatography (HPLC). Calibration curves were plotted in a range from 0125 to 2 lg ml1 of each toxin (AFB1 and ZEA). This methodology allowed obtaining greater reproducibility in the results.
Corn–adsorbent suspension preparation
Considering that, in general, the adsorbent is commercially used at 2% weight of finished feed and that preliminary test suspensions were prepared 50 lg ml1 for each YCW 1 mg of YCW 50 mg of ground corn were dissolved in 20 ml of the buffer solution at pH 2 or pH 6.
In vitro adsorption capacity
An aliquot of 500 ll of YCW suspension was added to each microtube containing 500 ll of 01, 025, 05, 1, 25 and 5 lg ml1 of AFB1 and 05, 5, 10, 20 and 50 lg ml1 of ZEA. Microtubes were kept at 37°C with mechanical agitation for 30 min and centrifuged for 10 min at 16 873 g (Labor 2K15 centrifuge, Sigma-Aldrich Chemie GmbH, Taufkirchen, Germany), and the supernatants were taken and evaporated to dryness under gentle stream of nitrogen gas. Each adsorption test was performed in duplicate, and control tests were also performed. The extracts were quantified by HPLC.
The study of the in vitro corn influence on toxin– adsorbent interaction was developed in the same way as previously described. The concentration of toxins was the same. The corn–adsorbent suspension used was performed as previously described.
Detection and quantification of aflatoxin B1
Aflatoxin B1 detection and quantification were performed by HPLC according to the methodology proposed by Trucksess et al. (1994). An aliquot (200 ll) was derivatized with 700 ll trifluoroacetic acid/acetic acid/water (20 : 10 : 70 v/v). Chromatographic separations were performed on a reverse-phase column (OmniSpher, C18, 150 9 46 mm i.d., 5-l particle size; Varian, Inc., Palo Alto, CA, USA). Acetonitrile/methanol/water (1 : 1 : 4 v/v) was used as mobile phase at a flow rate of 15 ml min1 . Fluorescence of AF derivative was recorded at excitation and emission wavelengths of 360 and 460 nm, respectively. Quantification of AFB1 was performed measuring the area and interpolating on a calibration curve obtained using standards of AFB1. The detection limit was 04 ng g1 .
Detection and quantification of zearalenone
The used methodology was described by Cervero et al. (2007). Extracts were resuspended in mobile phase methanol/water (70 : 30 v/v) and injected into the HPLC. Detection and quantification of ZEA was performed using a fluorescence detection system on Hewlett Packard 1100 Series. The chromatographic separations were carried out on a Luna C18 reverse-phase column (150 9 46 mm, 5-lm particle size; Phenomenex, Inc., Torrance, CA, USA), connected to a guard column. The flow of mobile phase was 1 ml min1 . The wavelengths of excitation and emission used were 280 and 460 nm, respectively. Quantification of ZEA was performed by measuring the area and its interpolation on a calibration curve obtained using solutions of ZEA standards. The limit of detection of the technique was 3 ng g1 .
Curve fitting and data processing
The amount of bounded toxin (AFB1 and ZEA) per unit weight of biomass was plotted as a function of the residual toxin concentration in solution at equilibrium according to the mathematical expressions proposed by three theoretical models (Langmuir, Frumkin-Fowler-Guggenheim (FFG) and Hill) and selected according to the form of isotherms. Mathematical equations and parameters of Langmuir, FFG and Hill models are shown in Table 2. The adjustment was made with ORIGIN® ver. 61 software (Microcal Software, Inc., One Roundhouse Plaza Northampton, MA, USA). The errors that affected the estimated parameters were calculated by error propagation. The quantity of adsorbed toxin was determined by the following equation:
Results
Yeast cell wall concentrations
A concentration 50 lg ml1 of each YCW was used to determine the best toxin/adsorbent relationship for the isotherms (data not shown). At this concentration, the YCW maintains the equilibrium conditions where coexist free and occupied sites on the adsorbent and toxin in the supernatant.
Aflatoxin B1 adsorption
Figure 1 shows AFB1 adsorption isotherms on YCW1 and YCW2 at both tested pH values. Isotherms, slightly sigmoid, were adjusted by both the Hill and FFG models. The setting parameters for both models are shown in Tables 3 and 4. Both YCWs adsorbed similar amounts of AFB1 at pH 2 and pH 6. Values of the adsorption constant were similar for both YCWs. Furthermore, the cooperativity indexes (n) obtained for both adsorbents were higher at pH 2.
Zearalenone adsorption
Figure 2 shows ZEA adsorption isotherms for YCW1 and YCW2 at both tested pH values. The curves correspond to the Hill fittings. The adjusting parameters obtained by the Hill and FFG models are shown in Tables 3 and 4. The fittings were comparable (R2 ), although the Hill model appears to represent better the adsorption process.
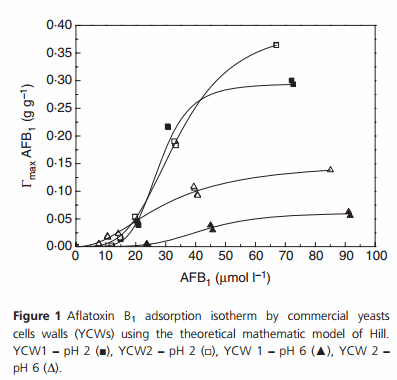
At pH 2, the YCW2 had a cooperativity index (n) of approximately 1, and in these conditions, there was no cooperativity. This fact agrees with a reasonably good fit using the Langmuir model. In relation to the effect of pH on YCW1, the results showed that at pH 2 there was a much more pronounced cooperative effect than at pH 6, where the cooperativity index (n) tended to be 1. It is the opposite for the YCW2. Considering the influence of pH on the maximun adsorption capacity (Γmax) for each YCW, it was observed to twice at pH 6 than at pH 2. At pH 2, the adsorption capacity for YCW1 was 010 (g g1 ) and the YCW2 was 014 (g g1 ), while at pH 6, it was 025 and 026 (g g1 ), respectively. There were no appreciable differences in the adsorption capacity for both adsorbents. The affinity of ZEA measured by the association constant (b) was greater for YCW2 (312 lmol l1 ) 1 at pH 2.
Adsorption of aflatoxin B1 in the presence of the corn
None of the tested adsorbents were able to adsorb AFB1 at appreciable amounts in the presence of corn.
Adsorption of zearalenone in the presence of the corn
Figure 3 shows ZEA adsorption isotherms of YCW1 and YCW2 at both tested pH values in the presence of corn. The graph shows that the isotherms were sigmoid, more pronounced at pH 6 and were adjusted with the Hill model, revealing a cooperative adsorption. The adjusting parameters are shown in Table 5. The fittings with the Hill model were comparable (R2 ). With respect to the pH effect on both YCWs, result shows that at pH 6, the cooperative effect was much more pronounced than at pH 2, where the cooperativity index (n) tended to be 1.
The effect of pH on the association constants was similar for both YCWs. Nonsignificant differences were observed in the adsorption capacity of both YCWs. ZEA affinity connected to a guard column was greatest for the YCW1 at pH 2 with a value of 112 052 (lbmol l1 ) 1 .
In the presence of corn, the maximum adsorption capacity (Γmax) for each YCW was similar at both pH values. The presence of the corn decreases the adsorption capacity of ZEA at pH 2 compared with pH 6.
Discussion
The present study evaluated the influence of the corn on the adsorption levels of AFB1 and ZEA by YCWs, and the results were analyzed by three different mathematical models.
Langmuir is the simplest theory for the adsorption and assumes both the equivalence of a finite number of sites on the adsorbent surface and the lack of lateral interactions between adsorbed molecules. The FFG theory assumes adsorption on a heterogeneous surface and is applied to either sigmoid, Langmuir or high-affinity isotherms. The adsorption model proposed by FFG assumes cooperative, repulsive or not interacting adsorption mechanisms depending on the sign of the adjusting parameter ‘a’. It must be considered that b values obtained by FFG model represent the adsorption constant extrapolated to zero coating. In such case, all sites are equivalent and no cooperativity is observed in the system. The adsorption constant b differs from the true thermodynamic constant of the adsorption equilibrium, which includes the activity of displaced solvent from sites at the adsorbent surface (b = Kas aH2O1 ). The Hill mathematical expression includes the Hill constant (KD), the maximum adsorption (Γmax) and the minimum number (n) of binding sites required for cooperative adsorption. The inverse of KD is the adsorption constant was called b. Both theoretical models can explain S-type isotherms through cooperative adsorption; however, curve fitting will provide either n > 1 (Hill equation) or ‘a’ > 0 (FFG equation).
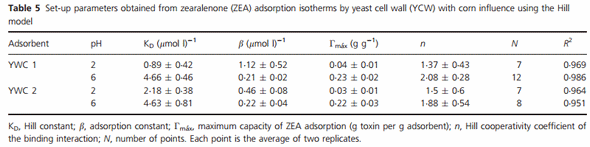
In this work, in spite of that comparable adjustments were obtained with different models, for comparison purposes, Hill’s model was chosen for further discussions. This model has been previously proposed to explain the shape of adsorption isotherms on YCWs and extracts derived from them (Yiannikouris et al. 2003, 2004a, 2004b). In one of the scarce studies on the adsorption of AFB1 using YCW, Yiannikouris et al. (2006) found that 6177 lg ml1 of AFB1 was adsorbed by 100 lg ml1 of YWC. Regarding the maximum coating (Γmax) obtained by both YCWs with AFB1, the values were similar to those obtained by Galvano et al. (1997) with activated carbon (012 g g1 ) and Dakovic et al. (2008) with copper modified montmorillonite (0066 g g1 ) as adsorbents.
Devegowda and Castaldo (2000) explained that the interaction of AFB1 with glucomannan of YCW was presumably through hydrogen bonds. On the other hand, the adsorption capacity in this study was found greater than those observed by Decker and Corby (1980), Phillips et al. (1990), Natour and Yousef (1998), Schall et al. (2000), Howes and Newman (2000), Desheng et al. (2005), Dakovic et al. (2008), who worked with different adsorbents such as activated carbon, aluminosilicates, diatomaceous earth, bentonite, modified extracts of yeast wall, modified montmorillonites and montmorillonites, respectively. The toxin adsorption mechanism was mainly cooperative and pH independent.
In the present work, both YCWs adsorbed ZEA at the studied pH conditions. These results agree with those reported by Yiannikouris et al. (2004a), who attributed ZEA adsorption to the presence of b-glucans in the walls. Values of the association constants in our assay were high, indicating the high affinity of these adsorbents for ZEA. The effect of pH on the adsorption constant was systematic, and similar for both YCWs.
Yiannikouris et al. (2004b) studied the influence of pH on the b-glucans–ZEA complex and found that under acidic and neutral conditions, the affinity was higher than under alkaline conditions, which could make the active participation of the conformation of b-glucans difficult. It is known that the three-dimensional structure of the polysaccharides that constitute the YCW allows the adsorption of mycotoxins or its metabolic derivatives. The tested YCWs differ in their chemical compositions. YCW2 contains a higher percentage of mannans (21%) and b-glucans (23%), while the YCW1 contains 59 and 174% of mannans and b-glucans, respectively. Yeasts cell wall are composed mainly by polysaccharides, proteins and lipids that offer numerous functional groups for the interaction, such as carboxyl, hydroxyl, phosphate and amine groups, as well as hydrophobic adsorption sites, such as aliphatic chains and aromatic carbon rings (Jouany et al. 2005; Ringot et al. 2005). For these reasons, the efficiency to adsorb mycotoxins is a complex function of the following three factors: chemical structure of the toxin, adsorbent composition and the pH of the medium. Spectroscopic studies with surface techniques of the toxin complexes with YCW or YCW derivatives could contribute to the explanation of the molecular level interaction.
The influence of a solid matrix such as ground corn on AFB1 and ZEA adsorption on the surface of the YCWs was studied. In this study, the presence of the corn impedes the adsorption of AFB1; however, in its absence YCWs adsorbed large amounts of toxin. Some authors such as Karaman et al. (2005), Raju and Devegowda (2000), Aravind et al. (2003) and Dvorska et al. (2003) reported that yeast cell wall derivatives were able to in vivo counteract the effects of AFB1. On the other hand, Janse van Rensburg et al. (2006) reported slight to moderate improvement during in vivo studies carried with broiler chickens under induced acute aflatoxicosis. Baptista et al. (2004) studied the ability of mannooligosaccharides, thermolysed yeast and active yeast to reduce the toxic effects in rats fed diets contaminates with AFB1. These authors reported that mannooligosaccharides and thermolysed yeast did not suppress the effect of AFB1, while active yeast reduced the aflatoxin symptoms.
In relation to ZEA, both YCWs adsorbed ZEA at simulated gastrointestinal pH conditions and under the presence of ground corn. These results suggest that both YCWs are future candidates for ZEA adsorption. Several in vitro assays have informed the ability to adsorb ZEA by different substrates including YCW; however, none of them evaluated for the ability to adsorb ZEA in the presence of corn.
In vitro evaluations of the corn influence could be useful as a screening method. They provide a better idea of the affinity for the toxins under simulated in vivo conditions, in a relatively short time and with a very small cost. Future in vivo studies should be conducted in vivo to determine the YCWs detoxification on animal production.
This article was originally published in Journal of Applied Microbiology 114, 655-662.
References
Aravind, K.L., Patil, V.S., Devegowda, G., Umakantha, B. and Ganpule, S.P. (2003) Efficacy of esterified glucomannan to counteract mycotoxicosis in naturally contaminated feed on performance and serum biochemical and hematological parameters in broilers. Poultry Sci 82, 571–576.
Baptista, A., Horii, J., Calori-Domingues, M., Micottida, G., Mastrodi Salgado, J. and Vizioli, M. (2004) The capacity of manno-oligosaccharides, thermolysed yeast and active yeast to attenuate aflatoxicosis. World J Microbiol Biotechnol 20, 475–481.
CAST (2003) Mycotoxins, Risks in Plant, Animal, and Human Systems. Task force report, ISSN 0194-4088; no. 139.
Ames, IA: Council for Agricultural Science and Technology. Cervero, M.C., Castillo, M.A., Montes, R. and Hern andez, E. (2007) Determination of trichothecenes, zearalenone and zearalenols in commercially available corn-based foods in Spain. Rev Iberoam Micol 24, 52–55.
Dakovic, A., Matijasevic, S., Rottinghaus, G.E., Ledoux, D.R., Butkeraitis, P. and Sekuli, Z. (2008) Aflatoxin B1 adsorption by natural and copper modified montmorillonite. Colloids Surf B 66, 20–25.
Decker, W.J. and Corby, D.G. (1980) Activated charcoal adsorbs aflatoxin B1. Vet Human Tox J 22, 388–389.
Desheng, Q., Fan, L., Yanhu, Y. and Niya, Z. (2005) Adsorption of Aflatoxin B1 on montmorillonite. Poultry Sci 84, 959–961.
Devegowda, G. and Castaldo, D. (2000). Mycotoxins: hidden killers in pet foods. Is there a solution. Technical Symposium on Mycotoxins. Nicholasville: Alltech. Dvorska, J.E., Surai, P.F., Speake, B.K. and Sparks, N.H. (2003) Protective effect of modified glucomannans against aurofusarin-induced changes in quail egg and embryo. Comp Biochem Phys Tox Pharmacol 135, 337–343.
Fink-Gremmels, J. and Malekinejad, H. (2007) Clinical effects and biochemical mechanisms associated with exposure to the mycoestrogen zearalenone. Anim Feed Sci Technol 137, 326–341.
Galvano, F., Pietri, A., Bertuzzi, T., Bognanno, M., Chies, L., De Angelis, A. and Galvano, M. (1997) Activated carbons. In vitro affinity for fumonisin B1 and relation of adsorption ability to physicochemical parameters. J Food Prot 60, 985–991.
Howes, A.D. and Newman, K.E. (2000). Compositions and methods for removal of mycotoxins from animal feed. U. S. US 6045834. Huwig, A., Freimund, S., Kappeli, O. and Dutler, H. (2001) Mycotoxin detoxication of animal feed by different adsorbents. Toxicol Lett 122, 179–188.
IARC (2002) International Agency for Research on Cancer. Lyon, France: IARC. Jansen van Rensburg, C., Van Rensburg, J., Van Ryssen, J., Casey, N. and Rottinghaus, G. (2006) In vitro and in vivo assessment of humic acid as an aflatoxin binder in broiler chickens. Poultry Sci 85, 1576–1583.
Jouany, J.P., Yiannikouris, A. and Bertin, G. (2005) The chemical bonds between mycotoxins and cell wall components of Saccharomyces cerevisiae have been identified. Arch Zoot 8, 26–50.
Karaman, M., Basmacioglu, H., Ortatatli, M. and Oguz, H. (2005) Evaluation of the detoxifying effect of yeast glucomannan on aflatoxicosis in broilers as assessed by gross examination and histopathology. Brit Poultry Sci 46, 394–400.
Khanafari, A., Soudi, H. and Miraboulfathi, M. (2007) Biocontrol of Aspergillus flavus and aflatoxin B1 production in corn. Iranian J Environ Health Sci Eng 4, 163–168.
Kogan, G. and Kocher, A. (2007) Role of yeast cell wall polysaccharides in pig nutrition and health protection. Livestock Sci 109, 161–165.
Natour, R.M. and Yousef, S.M. (1998) Adsorption efficiency of diatomaceous earth for mycotoxin. Arab Gulf J Scien Res 16, 113–127.
Phillips, T.D., Clement, B.A., Kubena, L.F. and Harvey, R.B. (1990) Detection and detoxification of aflatoxins. Prevention of aflatoxicosis and aflatoxin residues with hydrated sodium calcium aluminosilicate. Vet Human Toxicol 32, 15–19.
Raju, M.V. and Devegowda, G. (2000) Influence of esterifiedglucomannan on performance and organ morphology, oserum biochemistry and haematology in broilers exposed to individual and combined mycotoxicosis (aflatoxin, ochratoxin and T-2 toxin). Brit Poultry Sci 41, 640–650.
Ringot, D., Lerzy, B., Bonhoure, J.P., Auclair, E., Oriol, E. and Lanondelle, Y. (2005) Effect of temperature on in vitro ochratoxin A biosorption onto yeast cell wall derivates. Process Biochem 40, 3008–3016.
Schall, N., Simmler-Hubenthal, H. and Feldhaus-Hermann, G. (2000) Mykotoxin-adsorbens. Ger Offen. DE 19900813 A1 13.07.2000, available on: http://www.patent-de.com accessed 15 December 2012.
Shetty, P.H. and Jespersen, L. (2006) Saccharomyces cerevisiae and lactic acid bacteria as potential mycotoxin decontaminating agents. Trends Food Sci Tech 17, 48–55.
Trucksess, M.W., Stack, M.E., Nesheim, S., Albert, R.H. and Romer, T.R. (1994) Multifunctional column coupled with liquid chromatography for determination of aflatoxins B1, B2, G1, G2 in corn, almonds, Brazil nuts, peanuts and pistachio nuts: collaborative study. J AOAC Int 6, 1512–1521.
Yiannikouris, A., Francois, J., Poughon, L., Dussap, C.G., Bertin, G., Jeminet, G. and Jouany, J.P. (2004b) Alkali extraction of b-D-glucans from Saccharomyces cerevisiae cell wall and study of their adsorptive properties towards Zearalenone. J Agri Food Chem 52, 3666–3673.
Yiannikouris, A., Andre, G., Poughon, L., Francois, J., Dussap, C., Jeminet, G., Bertin, G. and Jouany, J. (2006) Chemical and conformational study of the interactions involved in mycotoxin complexation with b-D-glucans. Biomacromolecules 7, 1147–1155.
Yiannikouris, A., Poughon, L., Cameleyre, X., Dussap, C.G., Francois, J., Bertin, G. and Jouany, J.P. (2003) A novel technique to evaluate interactions between Saccharomyces cerevisiae cell wall and mycotoxins: application to zearalenone. Biotechnol Lett 25, 783–789.
Yiannikouris, A., Francois, J., Poughon, L., one Dussap, C.G., Jeminet, G. and Jouany, J.P. (2004a) Adsorption of zearalenone by beta-D-glucans in the Saccharomyces cerevisiae cell wall. J Food Prot 67, 1195–1200.