Introduction
The Mediterranean basin is a large geographical region with a temperate climate comprising several countries that belong to the southern part of Europe and parts of Asia and Africa. It supports a diversified agricultural system comprised of a wealth of field and greenhouse crops. The Mediterranean countries are the main suppliers of many fresh products to Europe. The major products of this area include vegetables (solanaceous crops, cucurbits and brassicas), legumes (beans, peas, peanuts), citrus fruits, pome fruits (apples, pears), stone fruits (apricots, peaches, nectarines, plums, cherries), nuts (almonds, pistachios, hazelnuts, walnuts), cereals (maize, rice, wheat) as well as tubers and roots from temperate and subtropical plants. The basin is also famous worldwide for some special products specific to this area e.g. olives and olive oil, grapes (table and wine) and soft fruits (figs, strawberries, raspberries). Figs, raisins, apricots and dates are major dried fruits produced in this region. Because of the suitable environmental conditions, these host plants are very often infected with serious plant pathogens and pests. Both pathogens and saprophytes can additionally synthesise toxic secondary metabolites, which may lead to the accumulation of mycotoxins in the colonised tissues (Logrieco et al., 2003).
Mycotoxins are toxic low molecular weight natural products (secondary metabolites) produced by filamentous fungi grown on several crops. These toxic molecules significantly influence the quality and safety of food and feed products. Mycotoxins are capable of causing disease and death in humans and other animals. Mycotoxicoses are examples of “poisoning by natural means” and thus, are considered analogous to the pathologies caused by exposure to pesticides or heavy metal residues. Mycotoxins in plant products and in processed food and feed have a significant economic impact and pose a serious problem for animal and human health (IARC, 1993). Because of their pharmacological activity, some mycotoxins or their derivatives can also be used as antibiotics or other kinds of drugs and some others have also been implicated as chemical warfare agents (Bennett and Klich, 2003). Mycotoxin contamination is now one of the most insidious challenges to food safety. The importance of these compounds is evident from the constant international attention paid to them due to their harmful impacts on animal and human health and the economy.
The most prevalent toxigenic fungi belong to the genera Aspergillus, Fusarium, Penicillium and Alternaria. These fungi pose serious phytopathological and mycotoxicological risks at preharvest and postharvest stages, as well as in processed food products (Castoria et al., 2008). Fusarium and Alternaria species are often classified as ‘field fungi’, because they require high moisture content in the substrate for growth and mycotoxin synthesis (>20%) whereas the ‘storage fungi’, mainly species of Aspergillus and Penicillium, can also grow well at lower moisture contents. Thus, Fusarium and Alternaria usually pose a high mycotoxicological risk at pre-harvest level or in freshly harvested products that are drying, whereas toxigenic species of Aspergillus and Penicillium represent a higher risk for food and feed products in storage or other kind of processing (Logrieco et al., 2003). Approximately, 400 secondary metabolites with toxigenic potential are produced by more than 100 fungi and the Food and Agriculture Organization (FAO) has estimated that as much as 25% of the world’s agricultural commodities are contaminated with mycotoxins, leading to significant economic losses. The most important mycotoxins associated with human and veterinary diseases include aflatoxins (AF), ergot akaloids, fumonisins (FUM), deoxynivalenol (DON) and other trichothecenes, ochratoxin A (OTA), patulin, zearalenone and citrinin. Mycotoxins exhibit four basic kinds of toxicity: acute (often causing deterioration of liver or kidney function), chronic, mutagenic, and teratogenic. Naturally occurring aflatoxins were classified as being carcinogenic to humans (Group 1) according to International Agency for Research on Cancer (IARC). Most of the developed countries have adopted regulations stipulating the maximum permissible levels for many important mycotoxins in foods and feeds (Bennett and Klich, 2003; Kabak and Dobson, 2009).
The severity of crop contamination (i.e. cereals, maize, peanuts, grapes) varies from year to year based on weather and other environmental factors. Aflatoxin contamination, for example, is usually worst during drought years; plants are weakened and become more susceptible to insect damage and other insults. Mycotoxins can enter the food chain in the field, during storage, or at later points. Mycotoxin problems are exacerbated whenever shipping, handling, and storage practices are conducive to mold growth. The economic consequences of mycotoxin contamination are profound. Crops with unacceptable levels of mycotoxins often have to be destroyed. Alternatively, contaminated food crops are sometimes diverted into animal feed. Giving contaminated feeds to susceptible animals can lead to reduced growth rates, illness, and death. Moreover, animals consuming mycotoxin-contaminated feeds can produce meat and milk that contain toxic residues and biotransformation products (Bennett and Klich, 2003).
The most important mycotoxins that occur in the Mediterranean basin are aflatoxins (B1, B2, G1 and G2) in dried fruits and nuts (figs, pistachios, hazelnuts, peanuts), ochratoxin A in grapes and raisins as well as trichothecenes and fumonisins in cereals. Aflatoxin contamination of dried fruits and ochratoxin A contamination of raisins are mostly widespread in the southern and eastern parts of the basin (African and Asian countries). In the northern part of the basin (European countries) trichothecenes and fumonisins (cereals) as well as ochratoxin A in grapes and wine are the most prevalent mycotoxins. However, the type and level of the different mycotoxins vary in each country as well as in different regions of each country depending mainly on environmental conditions (temperature, humidity etc). Additionally, mycotoxin contamination is also associated with pest and pathogen damage e.g. it is well documented that the highest OTA levels occur when the pest and disease control in vineyards give insufficient plant protection (Battilani et al., 2006; Ozay and Ozer, 2008).
A variety of chemical, biological and physical methods have been developed to control mycotoxigenic pathogens and to minimize mycotoxin production at pre- or post- harvest stages; to contribute to decontamination and/or detoxification of mycotoxins from contaminated foods and feeds; or to inhibit mycotoxin absorption in the gastrointestinal tract. Despite the fact that specific control measures can reduce mycotoxin formation in different commodities, the complete elimination of mycotoxin contaminated foods and feeds is currently not realistically achievable. This article reviews research trials and developments in the biological control of both fungal infection and mycotoxin formation for different major crops of the Mediterranean region (Table 1).
Table 1. Examples of biological agents that control mycotoxigenic fungi and mycotoxins.
Biological control of plant pathogens
The use of many of the available physical and chemical methods for the detoxification of agricultural products contaminated with mycotoxins is restricted due to problems concerning safety issues, possible losses in the nutritional quality of treated commodities, coupled with limited efficacy and cost implications (Kohl et al., 2011). Additionally, the development of fungicide resistance in many fungal pathogens as well as rising public concern over risks associated with pesticide use has led to a significant interest in the development of alternative nonchemical and environmentally friendly methods of pest and disease control. Biological control using microbial antagonists either alone or as part of an integrated control strategy to reduce pesticide inputs, has emerged as a promising approach for control of aflatoxins as well as some other mycotoxins in both pre- and post-harvested crops (Table 1). Several organisms including bacteria, yeasts and non-toxigenic Aspergillus fungi have been tested for their ability to reduce mycotoxin contamination. Despite the fact that the use of biocontrol agents has often been successful in the lab, their commercialization largely depends on whether they can consistently control mycotoxigenic fungi in different locations and cultivars and reduce mycotoxin levels below the legislative limits.
Microorganisms as biocontrol agents have a relatively narrow spectrum of activity compared to most chemical fungicides. Biological control of pre- or postharvest diseases is complex and involves a number of biological, environmental and economic variables. New biocontrol products, in addition to efficacy towards the targeted plant pathogen, must be safe and cost-effective (Nunes, 2002; Kohl et al., 2011). Despite all these problems, a number of successful commercial products have been on the market in different countries worldwide to control mycotoxigenic fungi, including Aspire (Candida oleophila strain 182; Ecogen Inc., Langhorne, PA), Bio-Save 10 and 11 (Pseudomonas syringae strains ESC10 and ESC 11; EcoScience Corp., Worcester, MA), AF36 (atoxigenic strain of A. flavus; Cotty, 1992), and Afla-Guard (atoxigenic strain of A. flavus NRRL 21882; Dorner and Lamb, 2006).
Biological control of Aspergillus flavus, A. parasiticus and aflatoxins in nuts and maize
Several Aspergillus species are among the most notorious plant pathogens because of the production of the carcinogenic mycotoxins called aflatoxins (AF). AF were isolated and characterized after the death of more than 100,000 turkeys (turkey X disease) in the UK, attributed to the consumption of mold-contaminated peanut meal. The four major AF are called B1, B2, G1, and G2 based on their fluorescence under UV light (blue or green) and the relative chromatographic mobility during thin-layer chromatography. AFB1 is the most potent natural carcinogen known (Squire, 1981) and is usually the major aflatoxin produced by toxigenic Aspergillusflavus strains that occur worldwide in soil and air. AF contamination is usually a significant problem in tropical or sub-tropical areas and can occur during crop development when the crop is either damaged (e.g., by insects) or stressed by heat and drought, and after maturation when the crop is exposed to high moisture and high temperature either before harvest or in storage (Bennett and Klich, 2003). In the Mediterranean area, peanuts, pistachios and maize are the major crops contaminated with AF. AF outbreaks have occurred in the basin, such as in 2003, when environmental conditions (very hot and dry for several months) during maize cultivation in northern Italy led to AF levels 5-70 times higher than during the years 1995-2000. This outbreak led to milk contamination by AFM1 produced by dairy cattle that consumed contaminated maize and to the dumping of thousands of tons of contaminated milk. As the global climate changes to hotter and drier, the possibility that all of Southern Europe may face a constant threat of AF contamination increases dramatically (Logrieco and Moretti, 2008).
A number of environmental and agronomic factors influence pod and seed infection of peanuts by AF-producing fungi, and consequently the aflatoxin contamination of peanuts at the pre-harvest stage. These factors depend largely on the field location as well as the year or season within the same field. Some environmental conditions are particularly favourable to fungal growth in combination with AF contamination of peanuts. In many cases though, it is possible to design appropriate agricultural practices and apply biocontrol agents (mainly atoxigenic Aspergillus strains) that help to reduce AF contamination in peanuts.
The pistachio is another nut that is quite often highly contaminated with AF. The major problem with pistachios is the “early split” that appears at the pre-harvest stage. The shell of the pistachio partially splits, usually at least a month before maturity and harvest. The hull covering the shell usually remains intact, protecting the kernels from infection by fungi and insects. However, in some cases, depending on the environmental conditions and the cultivar, the hull ruptures in a percentage of pistachios (early splitting), a condition conducive to infection with the AF producing fungi A. flavus and/or A. parasiticus. The aflatoxin content in single “early split” pistachio nuts often exceeds 20 ppb and sometimes exceeds 1000 ppb (Codex Alimentarius Commission, 2002; Bonjar, 2004).
A number of biological (i.e. bacteria, yeasts, atoxigenic Aspergillus strains) and chemical control agents (i.e. fungicides) have been reported to inhibit growth of aflatoxigenic fungi and subsequent AF biosynthesis. Isolates of A. flavus vary greatly in AF production, with some producing copious amounts and others none. One of the most successful patented approaches (Cotty, 1992; Cole, 1994) is the application of competitive, atoxigenic strains of A. flavus or A. parasiticus that has clearly been shown to reduce the AF contamination of agricultural products such as peanuts (Dorner et al., 2003), pistachios, rice, maize (Dorner et al., 1999), and cottonseed (Cotty, 1994). The effect is believed to be mediated mainly through competition for substrate and through the potential production of inhibitory metabolites. It is well established that AF contamination of crops can be suppressed by atoxigenic biocontrol strains. The application of non-aflatoxigenic strains of A. parasiticus in field soils has been reported to result in lower AF levels in peanut crops, with levels decreasing from 531, 96 and 241 μg kg-1 in untreated soils to levels of 11, 1 and 40 μg kg-1 for treated soils in three consecutive years (1987, 1988, and 1989), respectively (Dorner et al., 1992). Cotty (1994) discovered that the application of non-aflatoxigenic strains of A. flavus (on grain seeds) to soils of cotton fields was very effective for controlling AF production in cottonseed. In another 2-year study, Dorner et al. (1998, 2003) found that the application of atoxigenic strains of A. flavus and A. parasiticus on soils of peanut fields at different doses reduced AF contamination of peanuts by 74.3-99.9%. In another study, a non-aflatoxigenic strain of A. flavus reduced AF contamination by 80 to 95% in maize (Brown, 1991). In Greece, Tsitsigiannis et al. (2010) are screening large numbers of Aspergillus strains from pistachio orchards from different parts of the country in order to isolate native non-aflatoxigenic Aspergillus strains for further tests in reducing aflatoxin levels in pistachios. The use of native atoxigenic isolates also alleviates some of the concerns about safety and environmental impacts that might be of greater concern for introduced organisms.
Several studies have shown that, in order to be effective in reducing levels of AF contamination, atoxigenic strains of Aspergillus spp. must be applied in a method and at a time that allows successful competition with AF-producing Aspergillus strains. In theory, the atoxigenic strain is applied once per growing season when the overall A. flavus inocula levels in the field are low, thereby providing the applied strain the time and preferential exposure to be established in the crop, as well as a subsequent advantage when competing for crop resources with the toxigenic strains (Cleveland et al., 2003; Cotty and Melon, 2006). Two atoxigenic A. flavus strains, AF36 and NRRL 21882, are currently being used in USA to minimize AF contamination in crops. AF36 has been registered as a biopesticide by the U.S. Environmental Protection Agency and is being used for the management of aflatoxin producing fungi in cotton fields whereas NRRL 21882 is being used to prevent AF contamination in peanut fields (Chang and Hua, 2007). Afla-guard is composed of hulled barley coated with conidia of the atoxigenic strain of A. flavus (NRRL 21882) that does not produce AF, cyclopiazonic acid, or known AF biosynthetic precursors (Dorner, 2010). Large-scale tests of peanuts showed that applications of Afla-guard reduced aflatoxins by 85.2% in farmers’ stock peanuts and by as much as 97.5% in shelled, edible grade peanuts (Dorner and Lamb, 2006).
Advances in biological control have been aided by recent advances in molecular biology, genetic engineering and microbial genomics coupled with the discovery of the very broad catabolic potential present within the microbial world (Bata and Laztity, 1999; Karlovsky, 1999). A. flavus has no known sexual stage; consequently, most studies on its genetic variability have been made mainly by characterizing isolates based on vegetative compatibility group (VCG). Complementary nitrate-nonutilizing (nit) mutants are commonly used to identify compatible isolates. VCG analysis becomes cumbersome for genetic analysis involving large populations because it requires pairing each new mutant strain with a representative of each VCG determined for that population while eliminating isolates that are self-incompatible. In an effort to explain the atoxigenicity of some Aspergillus strains, Donner et al. (2010) compared genetic loci across the 68 kb AF biosynthesis gene cluster among 18 atoxigenic and two aflatoxin-producing vegetative compatibility groups (VCGs) from Nigeria and an atoxigenic VCG used commercially in North America. Five of the atoxigenic VCGs had large deletions (37-65 kb) extending from the teleomeric side of the aflatoxin biosynthesis cluster. The remaining 12 atoxigenic VCGs, including the VCG used for aflatoxin management in North America, contained all the AF pathway genes, but with defects. Two observations support the long-term persistence of atoxigenicity within A. flavus: first, a comparison of pathway genes revealed more changes in atoxigenic than in aflatoxin-producing isolates compared to the AF-producing strain NRRL 3357; and second, several non-synonymous changes are unique to atoxigenics. Atoxigenic VCG diversity was assessed with phylogenetic analyses. Although some atoxigenics share relatively recent ancestry, several are more closely related to aflatoxin producers than to other atoxigenics (Donner et al., 2010).
Besides biological strategies using atoxigenic strains, there are numerous reports regarding the antifungal properties of various lactic acid bacteria, which can exhibit activities against several Aspergillus species and a broad range of other mycotoxigenic fungi. These lactic acid bacteria have a long history of use in food and have been regarded as safe. These bacteria produce antimicrobial compounds, e.g., lactic acid, acetic acids, hydrogen peroxide, bacteriocins, and low-molecular-weight proteinaceous compounds, during carbon source metabolism, and they compete with other species by acidifying the environment and rapidly depleting nutrients (De Muynck et al., 2004; Kabak and Dobson 2009). For instance, earlier work reported the antifungal activity of a Lactobacillus casei strain that inhibited both growth and aflatoxin production in A. parasiticus (ElGendy and Marth, 1981). Coallier-Ascah and Idziak (1985) also reported that aflatoxin production by A. flavus was significantly reduced in the presence of Streptococcus lactis cultures, while antifungal activity from a Leuconostoc mesenteroides strain was reported, but no specific substance was identified (Suzuki et al., 1991). Another isolate from a silage inoculant, Lactobacillus caseipseudoplantarum, has also been reported to inhibit production of AFB1 and AFG1 by 80 and 92%, respectively (Gourama and Bullerman, 1997). In another study, Corsetti et al., 1998 reported antifungal activity from Lactobacillus sanfrancisco CBI which was caused by the formation of short-chained fatty acids, including caproic acid, which inhibited spoilage moulds from the genera Monilia, Aspergillus, Penicillium, and Fusarium. In addition the inhibition of aflatoxins by metabolites such as benzoic acid, cyclo (Gly-L-leucyl) methylhycantoin, and nevalonactone produced by specific lactic acid bacteria has been reported (Kabak et al., 2006).
In addition to the use of biocontrol agents in the field or at post-harvest level, a number of different fungal or bacteria cultures have been shown to detoxify AFB1. Fungal taxa such as Trichoderma sp., Phoma sp., Rhizopus sp., Sporotrichum sp. ADA, Sporotrichum sp. SF, and Alternaria sp. have been shown to degrade AFB1 by 65 to 99% in 5 days at 28±2°C (Shantha, 1999). Some strains of Lactobacillus, Streptococcus, and Bifidobacterium have been shown to degrade AFB1 in milk by fermentation (Karlovsky, 1999). Flavobacterium aurantiacum can significantly remove AFB1 from a liquid medium and a variety of food products including milk, peanuts, and corn without leaving toxic by-products. F. aurantiacum strain NRRL-B-184 removed 79.9–98.9%, 92.6–99.8%, and 88.7–100% AFB1 from phosphate-buffered saline, peanuts and red pepper, respectively within 48 h. In another study by D’Souza and Brackett (2000), the divalent cations Ca+2 and Mg+2 were shown to stimulate AFB1 degradation by F. aurantiacum. However, the bright orange pigmentation of this microorganism limits its use in food or feed fermentations (Line, 1994; Kabak et al., 2006).
Biological control of Aspergillus spp. and ochratoxins in grapes
Grapes are important for Europe, as European wine production represents about 70% of world production and thus is an important export commodity. Berry rot, known as sour rot or Aspergillus rot or black rot, is mainly caused by black Aspergilli which are very common in vineyards. Berry rot is particularly severe in the warmer grape-producing southern areas of Spain, Italy, France and Greece. Black Aspergillus spp. appear to be secondary invaders that infect berries only after they have been damaged by pre-harvest rain, other fungi, insects, mechanical impact or other factors (Leong et al., 2006). Soil and vine debris on soil are the primary sources of black Aspergillus spp. in vineyards (Kazi et al., 2008). Ochratoxin A (OTA) was first detected as a wine contaminant in 1996 and the role of Aspergillus section Nigri and A. carbonarius in OTA production discovered in Europe in 1999 (Abrunhosa et al., 2001; Battilani et al., 2001, 2003; Cabanes et al., 2002; Serra et al., 2003; Tjamos et al., 2004). OTA is a mycotoxin with nephrotoxic, nephrocarcinogenic, teratogenic and immunosuppressive properties (Battaglia et al., 1996) and EU has established a maximum level of 2.0 μg kg-1 (ppb) (European Commission 2007) in order to protect consumers. After the first detection of OTA in wine in several countries (Zimmerli and Dick, 1996), subsequent studies showed that A. carbonarius is predominantly responsible for OTA contamination of grapes, wine and vine fruits and detected OTA contamination of grapes and derived products such as raisins (Stefanaki et al., 2003; Tjamos et al., 2004), grape juices and wines (Zimmerli and Dick, 1996; Otteneder and Majerus, 2000; Soufleros et al., 2003; Stefanaki et al., 2003). Analyses of wine samples throughout Europe have shown that there is a gradient in OTA concentration with a decrease from red, to rose and to white wines (Visconti et al., 1999; Majerus et al., 2000; Soleas et al., 2001). Some parts of Southern Europe are more prone to contamination with the toxigenic Aspergillus species and OTA. OTA has also been found in much higher concentrations (max 53 μg kg-1) in dried vine fruit than in wine suggesting that A. carbonarius can dominate the drying vine fruit ecosystem. Additionally, in Greece a tendency of increasing OTA contamination in red dry wines from the northern to southern parts of the country was observed (Tjamos et al., 2006). There is a significant lack of knowledge in Europe on conducive pre-harvest climatic conditions and their relationship with risk of OTA contamination in grapes, and regarding the fate of OTA in wine production (Battilani et al., 2006).
Black Aspergilli, always present in the vineyard, colonize the berries at early veraison and surveys conducted in different countries have revealed that Aspergillus carbonarius and A. niger are the predominant Aspergilli in vineyards (Serra et al., 2003; Battilani et al., 2006; Bejaoui et al., 2006; Perrone et al., 2006; Tjamos et al., 2006). However, population studies of the black Aspergilli showed that although A. carbonarius was never dominant, its isolates were strong producers of OTA and A. niger was only occasionally the source of OTA in wine grapes (Serra et al., 2003; Battilani et al., 2004; Tjamos et al., 2004).
Biological control against Aspergillus rot in grapes has been tested by several research groups. The efficacy of microbial antagonists in combination with reduced rates of fungicide applications was also evaluated. The search for natural competitors has focused on the epiphytic organisms on leaves or fruits. Experimental results showed that phyllosphere yeast isolates are able to control sour rot caused by A. carbonarius in wine-producing vineyards. Guerzoni and Marchetti (1987) recorded the various genera of yeasts isolated from grape berries of different varieties, healthy and with sour rot symptoms. The most frequently occurring yeasts in the case of the infected berries were: Candida krusei, Kloeckera apiculata, Metschnikowia pulcherrima, Saccharomycopsis vini, Saccharomycopsis crataegensis and Issatchenkia occidentalis. In the case of healthy berries the frequently isolated yeasts were: Brettanomyces spp., Zygosaccharomyces spp., Pichia spp., Aureobasidium pullulans, Rhodotorula spp., Cryptococcus spp., and Geotrichum candidum. The yeasts Aureobasidium pullulans, Rhodotorula spp. and Cryptococcus spp. were the species with the highest incidences in most grape varieties.
Among the organisms reported to be effective were A. pullulans (Lima et al., 1997), Metschnikowia fructicola (Karabulut et al., 2003), and various other yeasts (Wilson et al., 1991; Zahavi et al., 2000; Bleve et al., 2006) and Bacillus subtilis (Pusey, 1989). In vitro experiments showed that some A. pullulans strains are highly suppressive to the rot caused by A. carbonarius on detached and wounded wine grape berries; i.e., under conditions that are favorable to the fungal pathogen. These strains are able to degrade OTA to the less toxic compound ochratoxin-a in vitro. In the few berries that were infected by Aspergillus sp. and were pretreated with the biocontrol agents, OTA contamination was lower than in infected nontreated berries (De Felice et al., 2007).
Zahavi et al., 2000, reported that Candida guilliermondii, strain A42 was effective in reducing incidence of decay caused by Botrytis cinerea and A. niger in wine-producing vineyards for two consecutive years. Schena et al., 1999 reported the activity of A. pullulans isolates against A. niger on table grapes along with their inhibitory activity against B. cinerea and Rhizopusstolonifer. The isolates used were L-47, known for its effectiveness, and two new isolates from a total of 41. These three isolates were applied in detached berry tests at three different concentrations of 106 ,107 and 108 cells per ml and evaluated after five days. Complete inhibition of Aspergillus niger on table grapes was achieved by all three isolates of A. pullulans with the concentration of 108 cells mL-1. The concentration of 107 cells mL-1 of L-47 gave 100% inhibition, while the other two were 5-60% effective. A concentration of 106 cells mL-1 was not satisfactory. Bleve et al., 2006, also identified several isolates of different species with antagonistic activity against A. niger and A. carbonarius on artificially wounded, infected berries.
The yeast-like fungus A. pullulans is one of the most widespread and well-adapted saprophytes, both in the phyllosphere (Blakeman and Fokkema, 1982) and in the carposphere. A. pullulans has a high tolerance to desiccation and irradiation (Elad et al., 1994; Kohl et al., 1995) and has been considered as a possible biological control agent of postharvest diseases on table grapes (Schena et al., 1999). Castoria et al. (2001), in experiments with grapes, showed that strain LS-30 of A. pullulans reduced A. niger infections by approximately 86% after storage at 20°C for six days. According to their experiments, competition for nutrients seems to be a possible mechanism of action of A. pullulans. Isolate LS-30 showed production of extracellular β-1,3-glucanase, but not chitinase, in vivo and in vitro. It does not seem to produce any kind of antibiotic substance nor to adhere to the hyphae of the pathogenic fungus. It has been described by other investigators that a possible mechanism of action of antagonists of postharvest pathogens is competition for nutrients, but it seems that high rates of extracellular β-1,3-glucanase production contribute to the possibility of the yeast to control post-harvest pathogens, considering that these enzymes cause disruption of the cell wall (as a carbon source).
Dimakopoulou et al. (2008) isolated another efficient A. pullulans strain (Y-1) and demonstrated that in detached berry tests (Figure 1) as well as in field experiments in Greece, it was as effective as the commercial fungicide mixture fludioxonil + cyprodinil in controlling sour rot, A. carbonarius infection and the consequent OTA contamination in must (Figure 2). The A. pullulans isolate Y-1 was applied twice, one week before veraison and two weeks before harvesting. It is noteworthy that neither the fungicide nor the yeast isolate reduced the percentage of A. niger infection on berries; however, a decrease in sour rot incidence and OTA level was observed in these treatments compared to the untreated control berries. This discrepancy between the population density of A. niger, sour rot incidence and OTA level can be explained by the fact that A. niger is considered as a minor contributor of sour rot and OTA contamination (Tjamos et al., 2004); the main species is A. carbonarius. Future work will focus on evaluating the antagonist’s ability to colonize berries either externally or internally by using molecular markers (Dimakopoulou, personal communication). Better understanding of its ecology may lead to an optimized formulation, time and mode of application; in order to be effective, Biological Control Agents (BCAs) must survive on the plant surface and consequently remain active against target pathogens during periods favourable to plant infection (Lo et al., 1998).
Figure 1. Biological control of Aspergillus carbonarius using the epiphytic yeast Aureobasidium pullulans on grape berries (cultivar “Fraoula”). A. Protection of berries treated with Aureobasidium pullulans strain Y1 (immersion in yeast suspension of 107 cfu mL-1) and 24h later infection with Aspergillus carbonarius. B. Control berries infected with Aspergillus carbonarius (25 μl of 104 conidia mL-1 per berry) (Dimakopoulou et al., 2008)
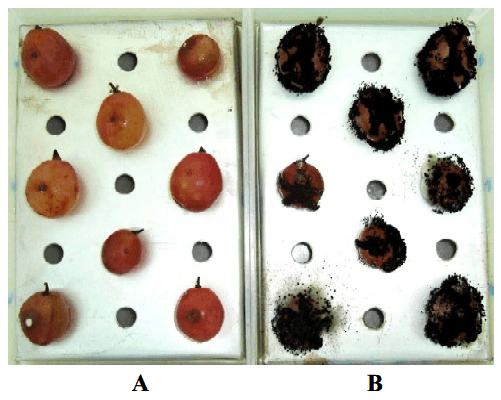
A. pullulans, in addition to being an effective epiphytic biocontrol microorganism, has been reported as an endophyte, found in plant parts without symptoms. Endophytic organisms usually develop within the tissues of the host without causing symptoms and often produce substances with antifungal or antibacterial activity. There are several cases where the existence of endophytic fungi or bacteria leads to induced resistance of the host. The endophytic organisms compared with epiphytic ones have advantages because they develop in conditions of reduced nutritional requirements and to some extent protected, but at the same time they can provide protection to the host. Schena et al. (2003) obtained 51 endophytic isolates of A. pullulans from cherry fruit flesh in order to assess their effectiveness to control post-harvest rots of cherry and table grapes. From the 51, two were selected, 533 and 547, which significantly reduced (32-80% in sour cherries and 59-64% in table grapes) post harvest infection by Botrytis cinerea. In particular it was demonstrated that 547 was able to survive under field conditions, proliferate in storage at cold temperatures and penetrate and colonize the flesh of the cherry or grape fruit if the application was made at the time of flowering.
Figure 2. Effect of fludioxonil+cyprodinil and Aureobasidium pullulans Y1 treatments on A) Sour Rot Incidence; B) Aspergillus carbonarius populations in grape berries and C) on ochratoxin A content in must. Bars represent the mean percentages for 2005 and 2006 and letters on the top of each bar indicate the results of the Duncan's test (P<0.05); values with different letters in each year are statistically different (Dimakopoulou et al., unpublished data; Dimakopoulou et al., 2008)
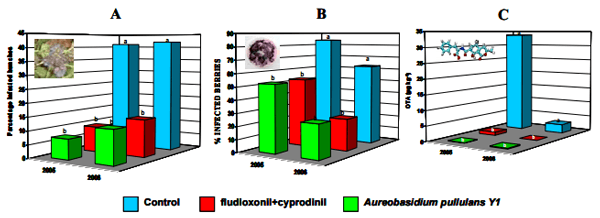
The efficacy of biological control methods that rely on the use of a single BCA can be variable depending upon the pathogen’s inoculum level and local environmental conditions (O’Neill et al., 1996, Guetsky et al., 2002). One potential way to reduce this variability and improve efficacy is to combine compatible biocontrol treatments that occupy different environmental niches and/or have different modes of action (Elmer and Reglinski, 2006). Therefore, a combination of the most efficacious yeast isolates in the in vivo detached berry test can be screened under field conditions.
Besides BCAs, Varga et al. (2000) reported the ability of various Aspergillus strains such as A. fumigatus, A. japonicus, and A. niger to degrade OTA in liquid YES media, with A. niger degrading OTA to ochratoxin-α and phenylalanine in solid media and liquid cultures. Additionally, Acinetobacter calcoaceticus has been shown to degrade 100% of OTA in ethanol minimal salts medium at an initial OTA concentration of 10 μg mL-1 at 30°C within 120 h (Hwang and Draughon, 1994).
Biological control of Fusarium spp. and associated mycotoxins in maize and wheat
The occurrence of Fusarium mycotoxins, particularly in cereal grains is of great concern in Mediterranean area, and their presence in processed feeds and foods is often associated with mycotoxicoses in humans or domesticated animals. The most common Fusarium mycotoxins are trichothecenes, zearalenone and fumonisins. Trichothecenes can cause a variety of toxic effects in animals including haemorrhagic syndrome in internal organs, immunosuppression (leukopenia) and disturbance of the nervous system, skin inflammation, digestive and blood disorders. Zearalenone (ZEA) is among the most widely distributed Fusarium mycotoxins in agricultural commodities and is often found at relatively high concentrations, especially in maize. ZEA is responsible for recurring toxicoses in livestock. ZEA has estrogenic effects causing reproductive disorders in farm animals (IARC, 1993).
Fumonisins are produced by a number of Fusarium species, notably Fusarium verticillioides (formerly Fusariummoniliforme = Gibberella fujikuroi), Fusariumproliferatum, Fusarium nygamai, as well as Alternariaalternata f. sp. lycopersici (Pitt, 1986; Marasas, 2001) and Aspergillus niger. Fumonisins B1 (FB1) and B2 (FB2) present the greatest mycotoxicological concern. Feeds contaminated by FB1 cause altered hepatic and immune function in cattle, poor performance in poultry, leukoencephalomalacia in horses and pulmonary oedema in swine. Maize contaminated by FB1 has also been associated with oesophageal cancer of humans in Africa, China, and the United States. The structural similarity of fumonisins with sphingosines suggests a role for these toxins as depletion agents of the complex sphingolipids from biological membranes accounting for their toxicity and, perhaps, their carcinogenicity. The evidence that cultures of F. verticillioides and samples of FB1 can promote liver cancer in rats, led to the classification of fumonisins as carcinogenic to animals and possibly to humans (Group 2B) (IARC, 1993). The major species with an economic importance is Fusariumverticillioides, which grows as an endophyte in both vegetative and reproductive maize tissues, often without causing any clear disease symptoms in the plant. However, if the appropriate fungal and plant genotype are present under favourable weather conditions and in the presence of wounds caused by insect damage, the fungus can cause seedling blight, stalk rot, and ear rot (Nelson, 1993). The fungus is present in virtually all maize samples (Marshall, 1983, Marasas et al., 2001). The fungus is transmitted vertically and horizontally to the next plant generation via mainly plant debris and to a less extent by seed infection. Horizontal infection is the manner by which this fungus is dispersed in the field by rain and water and disease occurs through external local infection. Horizontal infection can be reduced by the application of certain fungicides and seed treatments can also greatly reduce seed transmission. The endophytic phase can result from vertical transmission but fumonisin contamination is mostly due to local and not endophytic infection (Plumlee and Galey, 1994). Although it is phytotoxic, fumonisin B1 is not required for plant pathogenesis (Marasas, 1996; Desjardins and Plattner, 2000).
A biological control system using an endophytic bacterium, Bacillus subtilis, has been developed that shows great promise for reducing mycotoxin accumulation during the endophytic (vertical transmission) growth phase. It was shown that this isolate is able to occupy the same ecological niche as F. verticillioides within the plant, and it was postulated to act competitively to the pathogen. Additionally to B. subtilis, an isolate of Trichoderma gave also promising results in postharvest control of the development and toxin accumulation of F. verticillioides on maize in storage conditions (Bacon et al., 2001).
Studies by Etcheverry et al. (2009) investigated the antagonistic activity of bacterial and yeast isolates (Bacillus amyloliquefaciens BA-S13, Microbacterium oleovorans DMS 16091, Enterobacter hormomaechei EM-562T, and Kluyveromyces spp. L14 and L16 isolates) on mycelial growth of toxigenic F. verticillioides and A. flavus strains. The experiments carried out on 3% maize meal extract agar at different water activities (0.99, 0.97, 0.95, and 0.93) showed that the antagonistic isolates M. oleovorans, B. amyloliquefaciens and Kluyveromyces sp. (L16) led to an increase of lag phase of growth and a decrease of the growth rate of all fungal strains in vitro. In vitro experiments using biocontrol and pathogenic strains in co-inoculated cultures in non-rhizospheric maize soil (centrally or sprayed inoculated) and in vitro infected maize (ears apex- and base- inoculated) showed that B. amyloliquefaciens significantly reduced F. verticillioides and A. flavus numbers in maize soil inoculated centrally whereas Kluyveromyces sp. L16 reduced F. verticillioides and A. flavus colonies in maize soil that was spray inoculated. Among the strains tested, Kluyveromyces sp. L16 was the most effective biocontrol agent limiting F. verticillioides infections on maize ears (Etcheverry et al., 2009).
The species predominantly found associated with Fusarium head blight (FHB) of wheat and other small-grain cereals in the Mediterranean region are F. graminearum and its widespread teleomorph G. zeae, F. culmorum and F. avenaceum (Logrieco et al., 2003). FHB causes severe damage to wheat and other cereals. The disease causes significant losses by reducing grain yield and quality in many wheat production regions in the Mediterranean basin, especially in areas with warm temperatures and high relative humidity or frequent precipitation during the heading and blossoming periods. The fungus survives and reproduces in crop residues and is dispersed by wind or rain to the developing wheat or barley. Wheat is most susceptible during the flowering growth stages, but some infection can still occur during kernel development. F. graminearum is known to produce two important mycotoxins, deoxynivalenol (DON) (a type of trichothecene) and zearalenone, which can contaminate the diseased grain. The mycotoxin DON can cause reduced feed intake and lower weight gain in animals at levels as low as 1–3 ppm. Vomiting and feed refusal can occur when levels of DON exceed 10 ppm. Humans are also sensitive to DON (Yuen and Schoneweis, 2007).
Biological control experiments showed that a strain of Fusariumequiseti (G9) has been consistently effective in controlling FHB and reducing DON formation (by more than 70%) on wheat (Dawson et al., 2004). Yeasts of the genus Cryptococcus also are very effective against FHB (up to 59% reduction) on durum wheat in the field (Khan et al., 2001). Among the factors that influence the successful application and colonization of FHB antagonists in the field include the variable and sporadic arrival of pathogen inoculum on wheat heads over an extended period of head susceptibility, the phylloplane environment with significant fluctuations in temperature, moisture, and nutrient availability as well as the potential deleterious effect of UV light (Schisler et al., 2002). In addition, four yeasts (NRRL Y-30213, NRRL Y-30214, NRRL Y-30215, and NRRL Y-30216) and a bacterium (Bacillus sp. NRRL β-30212) have been identified as excellent antagonists that are capable of suppressing FHB in cereals, particularly wheat and barley (Schisler, 2006). In another study, 4 out of 54 microbial strains that were isolated from wheat anthers and that utilized tartaric acid in vitro, were effective against Gibberella zeae, whereas only 3 out of 170 tested isolates that did not utilize tartaric acid were effective against this pathogen, showing the potential benefit of pre-screening candidate FHB antagonists for their ability to utilize tartaric acid (Khan et al., 2001). Another report showed that three bacterial isolates, Bacillus subtilis H-08-02, Bacillus cereus L-07- 01, and Bacillus mycoides S-07-01, inhibited Fusarium graminearum by 60, 52, and 55%, respectively (Fernando et al., 2002), whereas Lysobacter enzymogenes strain C3 significantly reduced FHB severity in a particular wheat cultivar but had little or no effect on disease development on some other wheat cultivars. Treatment of heads of FHB susceptible wheat with a Streptomyces sp. reduced both FHB disease severity and associated loss in grain weight by approximately 50% under glasshouse conditions (Nourozian and Khodakaramian 2006). Khan and Doohan (2009) found that the use of Pseudomonas fluorescens strains MKB 158 and MKB 249 and Pseudomonas frederiksbergensis strain 202 reduced the severity of FHB disease symptoms caused by F. culmorum in wheat and barley grown under both greenhouse and field conditions, and Pseudomonas sp. AS 64.4 isolated from wheat anthers was as effective as the fungicide tebuconazole for controlling FHB disease severity under field conditions (Schisler et al., 2006). Treatment with either of the two P. fluorescens strains (MKB 158 and MKB 249) also resulted in a 74 to 78% reduction in DON levels in wheat and barley grains in the F. culmorum - inoculated field trails (Kabak and Dobson, 2009; Khan and Doohan, 2009).
Transgenic strategies to ear mold/mycotoxin resistance are now feasible as well. These potentially include genetically increased fungal resistance, enhanced resistance to insect feeding, and detoxification/prevention of mycotoxins in the grain. An example of these strategies the transgenic maize expressing Bacillus thuringiensis (Bt) toxin that is already on the market and is targeted several maize insects. Studies have shown that Bt maize hybrids have the potential to reduce Fusarium ear rot levels in field-harvested grain, presumably by reducing feeding of Bt susceptible insects in ear tissues. The plant though will still be vulnerable to non-insect routes of entry to Fusarium. However, because of the European legislation these transgenic plants are not widely used in the Mediterranean region. A second strategy that has been carried out in several laboratories is to interfere with the ability of Fusarium to infect and colonize the maize ear. This could potentially be achieved by incorporation of resistance genes in elite varieties or by overexpression of specific antifungal proteins or metabolites, or by enhancement of the plant’s own innate immune system in kernel tissues. The challenge though in these approaches is to achieve reproducible and stable maize lines with enhanced and durable ear mold resistance under field conditions. A third strategy aims to prevent mycotoxin biosynthesis, or to detoxify mycotoxins in planta, creating transgenic lines with genes that code for instance for enzymes that degrade fumonisins (Duvick et al., 2001).
Biological control of patulin and ochratoxin producing fungi in pears and apples
Penicilliumexpansum, the blue mold that causes soft rot of apples, pears and other fruits, is recognized as one of the most common producers of the mycotoxin patulin. Patulin has been found as a contaminant in many mouldy fruits, vegetables, cereals, and other foods; however, the major sources of contamination are apples and apple products. Patulin occurs mainly in damaged fruits, although the presence of mould does not necessarily indicate the presence of patulin. Patulin is also regularly found in unfermented apple juice, although it does not survive the fermentation into cider products. Patulin is quite toxic at high concentration in laboratory settings, but evidence for natural poisoning is indirect and inconclusive. Nevertheless, a provisional maximum tolerable daily intake has been established in many countries worldwide (Janisiewicz et al., 2002; Bennett and Klich, 2003).
The use of fungicides or other chemicals is the most common procedure to prevent P. expansum soft rots at the postharvest stage, but legislation is becoming more and more restrictive. The use of biocontrol agents as an alternative tool has been investigated in several studies. Several epiphytic microorganisms isolated from fruits and leaf surfaces of apples have been screened for antagonistic activity against P. expansum (Ippolito et al., 2000; Nunes et al,. 2007; Morales et al., 2008; Robiglio et al., 2011).
The biocontrol activity of the yeast A. pullulans on decay of apple fruit caused by P. expansum and B. cinerea and its ability to induce biochemical defense responses were investigated in apple tissues. In apple wounds, A. pullulans multiplied rapidly and controlled decay caused by either B. cinerea or P. expansum. At the end of the storage period, A. pullulans reduced the incidence of gray and blue mold of apples by 89% and 67%, respectively. In addition to controlling the disease, A. pullulans led to a transient increase in β-1,3- glucanase, chitinase, and peroxidase activities starting 24 h after treatment and reaching maximum levels 48 h and 96 h after treatment, indicating the likely basis of its biocontrol activity (Ippolito et al., 2000).
Among several bacterial isolates that have been tested, strain CPA-5 of Pseudomonas syringae, isolated from an organic orchard, was very effective against Botrytis cinerea, P. expansum and Rhizopusstolonifer at various concentrations on ‘Golden Delicious’ apple, and ‘Blanquilla’, ‘Rocha’ and ‘Conference’ pears. Additionally, under cold storage conditions and in semi-commercial trials, P. syringae (CPA-5) significantly reduced development of P. expansum and B. cinerea on ‘Golden Delicious’ apple, and ‘Blanquilla’ and ‘Rocha’ pears. Control of P. expansum obtained with CPA-5 at 108 cfu mL-1 on ‘Golden Delicious’ apple and ‘Rocha’ pear, was equal to the fungicide imazalil. Population ecology experiments showed that P. syringae CPA-5 increased more than 100-fold during the first 50 days after application, and then remained stable on apple fruits, and slightly decreased on pears, indicating the high capacity of this biocontrol agent to colonize wound surfaces of pome fruits under cold storage conditions (Nunes et al., 2007).
In another study, Morales et al. (2008) evaluated the effect of two biocontrol agents (Candida sake CPA- 2 and Pantoea agglomerans CPA-1) on P. expansum development and patulin accumulation in cold storage and ambient storage. A cell suspension of a combination of C. sake or P. agglomerans with a P. expansum conidial suspension was applied on wounded apples. Both BCAs controlled blue rot and patulin accumulation during cold storage. C. sake-treated apples showed a significant reduction of P. expansum growth. On the other hand, P. agglomerans-treated apples were lower in patulin accumulation. However, BCA treatment could not control blue rot and patulin accumulation during further storage at room temperature (Morales et al., 2008).
Preliminary assays in vitro and in vivo on the combination of some nutrients with Candida sake strain CPA-1 showed that combination of the antagonist with ammonium molybdate was most effective for reducing blue mold decay caused by P. expansum on apples and pears. In laboratory trials, improved control of blue and gray molds was obtained with the application of ammonium molybdate (at concentrations of 1, 5, 10, and 15 mM) alone or in combination with C. sake at 2×106 or 2×107 cfu mL-1 on “Blanquilla” pears stored at 20°C. In semi-commercial trials, the efficacy of C. sake at 2×106 cfu mL-1 on reducing P. expansum and B. cinerea decay was enhanced more than 88% with the addition of 5 mM ammonium molybdate in the 1999-2000 season. In these two seasons, the performance of C. sake at 2×106 cfu mL-1 with the addition of ammonium molybdate was similar to or greater than that of C. sake at 2×107 cfu mL-1. Similar control of blue mold was obtained on pears stored under low oxygen conditions. The preharvest application of ammonium molybdate did not reduce postharvest blue mold decay. The population of C. sake on pear wounds significantly decreased though in the presence of ammonium molybdate 1 and 5 mM at 20°C and 1°C. Studies have shown that ammonium molybdate affects metabolic processes in several organisms. The basis of its biological activity was reported to be its ability to inhibit acid phosphatase, which interferes with phosphorylation and dephosphorylation, one of the most important processes of cell regulation (Teixidó et al., 1998b; Nunes et al., 2002).
Since infection of fruits by postharvest pathogens often occurs in the field prior to harvest it would be a great advantage for the farmer to apply biocontrol agents before harvest, which would reduce initial infection and then remain active and suppress the pathogens in storage. Leibinger et al. (1997), using mixtures of yeasts and bacteria for control of apple postharvest diseases, obtained a level of control of P. expansum and B. cinerea that was similar to that obtained with a fungicide. For this strategy to be successful, biocontrol inocula need tolerance to environmental stresses, especially high temperature, low water activity (aw), low nutrient conditions, and UV light, for effective establishment and disease control (Deacon, 1991). Physiological studies with C. sake CPA-1 demonstrated that growth conditions can be modified so that specific endogenous compounds such as sugar alcohols and trehalose accumulate in cells, resulting in improved viability over a wider range of relative humidity with a retained biocontrol efficacy. Accumulation of low- (glycerol and erythritol) and high- (arabitol and mannitol) molecularweight sugar alcohols occurs in many fungi grown under conditions of environmental stress. Intracellular accumulation of these polyols reduces cytoplasmatic water activity (aw) and enables enzymes to remain active during periods of water stress (Teixidó et al., 1998b).
Conclusions
It is well established that the preferred strategy for reducing the concentration of mycotoxins in foods and animal feeds is the prevention of mycotoxin formation during pre-harvest and/or post-harvest stages of the various susceptible crops. Several biocontrol agents have been tested in laboratory and field experiments to effectively reduce colonization and mycotoxin contamination by Aspergillus, Fusarium and Penicillium species in the Mediterranean basin, an area with significant problems with mycotoxins in several crops. In this respect, atoxigenic fungal strains are being widely used to prevent pre-harvest AF contamination of crops such as peanuts, pistachios, maize, and cottonseed by Aspergillus spp. in several parts of the world including the Mediterranean area. Recent advancements in the use of biocontrol strategies involving atoxigenic strains has led to registration of commercial products with increased practical applications for the benefit of growers. On the other hand, genetic and molecular approaches aimed at preventing mycotoxin biosynthesis have not yet reached commercial application in the field and require substantial further development. More studies are required to evaluate the potential efficacy of various biological agents, including studies focusing on the dose, formulation and timing of the applications as well as molecular studies that elucidate impacts of the biocontrol agents in alterations of plant physiology or plant innate immune system. Additionally, all the biocontrol agents or GMOs that are planned to be developed for the control of mycotoxigenic fungi have to pass all the necessary safety tests and stages in order to be safe for the consumers.
Prevention strategies at the post-harvest stage can only be effective for those mycotoxins that are formed during this stage of the food production. Natural fungal contamination that occurs pre-harvest can only be minimized post-harvest by application of processing techniques which will minimize subsequent entry into the food and feed chain where possible by inhibition, detoxification or degradation of the mycotoxin. Many scientists are working on this area trying to discover appropriate and cost effective formulations that can effectively detoxify or decontaminate food products.
We also need to point out that in addition to biocontrol methods, key management tools and traceability procedures must be followed that will facilitate effective preservation of stored commodities with minimum loss in quality and quantity. These measures include a) accurate and regular moisture measurements to ensure safe thresholds are not breached b) efficient and rapid drying of wet commodities for medium and long term storage in disinfected and hygienic silos or warehouses that are free of pests and fungal inocula c) traceability during storage and transport for processing d) representative sampling and effective diagnostic tools that can be used to monitor and quantify mycotoxins rapidly (Magan and Aldred, 2007).
Current research needs to utilise the existing sound control measures in order to develop integrated strategies that can counteract the effects of mycotoxigenic fungi and prevent the production of the associated mycotoxins in human and animal food chains.
Literature cited
Abrunhosa L., R.R.M. Paterson, Z. Kozakiewicz, N. Lima and A. Venancio, 2001. Mycotoxin production from fungi isolated from grapes. Letters in Applied Microbiology 32, 240-242.
Bacon C.W., I.E. Yates, D.M. Hinton and F.Meredith, 2001. Biological control of Fusarium moniliforme in maize. Environmental Health Perspectives 109, 325-332 (Supplement 2).
Bata A. and R. Laztity, 1999. Detoxification of mycotoxincontaminated food and feed by microorganisms. Trends in Food Science and Technology 10, 223-228.
Battaglia R., Hatzold T., Kroes R., 1996. Conclusion from the workshop on ochratoxin in food, organized by ILSI Europe in Aixen-Provence, Food Additives and Contaminants 13, Supplement, 1–3.
Battilani P., P. Giorni, L. Languasco, A. Pietri and T. Bertuzzi, 2001. Dynamic of fungi responsible for ochratoxin A in grape. Results from a survey on their presence in Italy in 2000. In: Book of Abstracts `Bioactive Fungal Metabolites- Impact and Exploitation’, Swansea (Wales), UK, 2001, 47 (abstract).
Battilani P., P. Giorni and A. Pietri, 2003. Epidemiology of toxin-producing fungi and ochratoxin A occurrence in grape. European Journal of Plant Pathology 109, 715-722.
Battilani P., N. Magan and A. Logrieco, 2006. European research on ochratoxin A in grapes and wine. International Journal of Food Microbiology 111, S2-S4.
Bennett J. W. and M. Klich, 2003. Mycotoxins. Clinical Microbiology Reviews 16(3), 497–516.
Blakeman J.P., and N.J. Fokkema 1982. Potential for biological control of plant diseases on the phylloplane. Annual Review of Phytopathology 20, 167-192.
Bleve G., F. Grieco G .Cozzi A. Logrieco,A. Visconti, 2006. Isolation of epiphytic yeasts with potential for biocontrol of Aspergillus carbonarius and A. niger on grape. International Journal of Food Microbiology 108, 204-209 (abstract).
Bonjar G.H.S., 2004. Incidence of aflatoxin producing fungi in early split pistachio nuts of Kerman, Iran. Journal of Biological Sciences 4, 199-202.
Brown R.L., P. J. Cotty, and T. E. Cleveland. 1991. Reduction in aflatoxin content of maize by atoxigenic strains of Aspergillus flavus. Journal of Food Protection 54, 623–626.
Cabanes F.J., Accensi, M.R. Bragulat, M.L., Abarca G., Castella, S. Minguez and A. Pons, 2002. What is the source of ochratoxin A in wine- International Journal of Food Microbiology 79, 213–215.
Castoria R, F. De Curtis, G. Lima, L. Caputo, S. Pacifico and V. De Cicco, 2001. Aureobasidium pullulans (LS-30) an antagonist of postharvest pathogens of fruits: study on its modes of action. Postharvest Biology and Technology 22, 7–17.
Castoria R., S.A.I. Wright, and S. Droby, 2008. Biological control of mycotoxigenic fungi in fruits. In: Mycotoxins in Fruits and Vegetables. (R. Barkai-Golan, N. Paster, ed.), 311– 333.
Chang P-K. and S.S. Hua, 2007. Nonaflatoxigenic Aspergillus flavus TX9-8 competitively prevents aflatoxin accumulation by A. flavus isolates of large and small sclerotial morphotypes. International Journal of Food Microbiology 114, 275–279.
Cleveland T., P.F. Dowd, A.E. Desjardins, D. Bhatnagar and P. J. Cotty, 2003. United States Department of Agriculture– Agricultural Research Service research on pre-harvest prevention of mycotoxins and mycotoxigenic fungi in US crops. Pest Management Science 59, 629–642.
Coallier-Ascah, E.S. and J. Idziak, 1985. Interaction between Streptococcus lactis and Aspergillus flavus on production of aflatoxin. Applied and Environmental Microbiology 49, 163-167.
Codex Alimentarius Commission, 2002. Discussion paper on aflatoxins in pistachios Joint FAO/WHO Food Standards Programme, Rotterdam, The Netherlands - CX/FAC 02/22.
Cole R.J., J.W. Dorner and P.D. Blankenship, 1994. Nonaflatoxigenic Aspergillus parasiticus strains and their use in controlling aflatoxin contamination. U.S. Patent 5, 292, 661.
Corsetti A., M.J. Gobbetti and P. Rossiand Damiani, 1998. Antimould activity of sourdough lactic acid bacteria: identification of a mixture of organic acids produced by Lactobacillus sanfrancisco CB1. Applied Microbiology and Biotechnology 50, 253-256.
Cotty P.J., 1992. Use of native Aspergillus flavus strains to prevent aflatoxin contamination. U.S. Patent 5, 171, 686.
Cotty P.J., 1994. Influence of field application of an atoxigenic strain of Aspergillus flavus on the populations of Aspergillus flavus infecting cotton bolls and on the aflatoxin content of cottonseed. Phytopathology 84, 1270–1277.
Cotty P.J. and J.E. Mellon, 2006. Ecology of aflatoxin producing fungi and biocontrol of aflatoxin contamination. Mycotoxin Research 22 (2), 110-117.
Dawson W.A.J.M, M. Jestoi, A. Rizzo, P. Nicholson and G.L. Bateman. 2004. Field evaluation of fungal competitors of Fusarium culmorum and F. graminearum, casual agents of ear blight of winter wheat, for the control of mycotoxin production in grain. Biocontrol Science Technology 14, 783–799.
De Felice D.V., M. Solfrizzo, F. De Curtis, G. Lima, A. Visconti, and Castoria, R., 2008. Strains of Aureobasidium pullulans can lower ochratoxin A contamination in wine grapes. Phytopathology 98, 1261-1270.
De Muynck C, A.I. Leroy, S. De Maeseneire, F. Arnaut, W. Soetaert, E.J. Vandamme, 2004. Potential of selected lactic acid bacteria to produce food compatible antifungal metabolites. Microbiological Research 159, 339-346.
Deacon J.W., 1991. Significance of ecology in the development of biocontrol agents against soil-borne plant pathogens. Biocontrol Science Technology 1, 5-20.
Desjardins A.E. and R.D. Plattner, 2000. Fumonisin B(1)-nonproducing strains of Fusarium verticillioides cause maize (Zea mays) ear infection and ear rot. Journal of Agricultural and Food Chemistry 48 (11), 5773-5780.
Dimakopoulou M., S.E Tjamos, P.P. Antoniou, A. Pietri, P. Battilani, N. Avramidis, E.A. Markakis and Tjamos E.C., 2008. Phyllosphere grapevine yeast Aureobasidium pullulans reduces Aspergillus carbonarius (sour rot) incidence in wine-producing vineyards in Greece. Biological Control 46, 158-165.
Donner M., J. Atehnkeng, R.A. Sikora, R. Bandyopadhyay and P.J. Cotty, 2010. Molecular characterization of atoxigenic strains for biological control of aflatoxins in Nigeria. Food Additives & Contaminants: Part A: Chemistry, Analysis, Control, Exposure & Risk Assessment 27 (5), 576-590.
Dorner J.W. and M.C. Lamb, 2006. Development and commercial use of afla-guard, an aflatoxin biocontrol agent. Mycotoxin Research 21, 33–38.
Dorner J.W., R.J. Cole and P.D. Blankenship, 1992. Use of a biocompetitive agent to control preharvest aflatoxin in drought stressed peanuts. Journal of Food Protection 55, 888-889.
Dorner J.W., R.J. Cole and P.D. Blankenship, 1998. Effect of inoculum rate of biological control agents on preharvest aflatoxin contamination of peanuts. Biological Control 12, 171–176.
Dorner J.W., R.J. Cole, and D.T. Wicklow, 1999. Aflatoxin reduction in corn through field application of competitive fungi. Journal of Food Protection 62, 650–656.
Dorner J.W., R J. Cole, W.J. Connick, D.J. Daigle, M.R. McGuire, and B.S. Shasha, 2003. Evaluation of biological control formulations to reduce aflatoxin contamination in peanuts. Biological Control 26, 318–324.
D’Souza, D.H. and R.E. Brackett, 2000. The influence of divalent cations and chelators on aflatoxin B1 degradation by Flavobacterium aurantiacum. Journal of Food Protection 63, 102-105.
Duvick J., 2001. Prospects for reducing fumonisin contamination of maize through genetic modification. Environmental Health Perspectives 109 (Supplement 2), 337-342.
Elad Y., J. Köhl and N.J. Fokkema, 1994. Control of infection and sporulation of Botrytis cinerea on bean and tomato by saprophytic bacteria and fungi. European Journal of Plant Pathology 100, 315-336.
El-Gendy, S.M. and E.H. Marth, 1981. Growth of aflatoxin production by Aspergillus parasiticus in the presence of Lactobacillus casei. Journal of Food Protection 44, 211-212.
Elmer, P.A.G. and T. Reglinski, 2006. Biosuppression of Botrytis cinerea in grapes. Plant Pathology 55, 155-177.
Etcheverry M.G., A. Scandolara, A. Nesci, M.S.V. Boas Ribeiro, P. Pereira and P. Battilani, 2009. Biological interactions to select biocontrol agents against toxigenic strains of Aspergillus flavus and Fusarium verticillioides from maize. Mycopathologia 167, 287–295.
Fernando W.G.D., Y. Chen and P. Parks, 2002. Effect of three Bacillus sp. from wheat on FHB reduction. In: National Fusarium Head Blight Forum Proceedings. U.S. Wheat and Barley Scab Initiative, East Lansing, MI, USA, 73–76.
Gourama, H. and L.B. Bullerman, 1997. Anti-aflatoxigenic activity of Lactobacillus casei subsp. pseudoplantarum. International Journal of Food Microbiology 34, 131-143.
Guerzoni, E. and R.Marchetti, 1987. Analysis of yeast flora associated with grape sour rot and the chemical disease markers. Applied and Environmental Microbiology 53 (3), 571-576.
Guetsky R., D. Shtienberg, Y. Elad and E. Fischer, A. Dinoor, 2002. Improving biological control by combining biocontrol agents each with several mechanisms of disease suppression. Phytopathology 92 (9), 976-985.
Hwang, C-A., and F.A. Draughon, 1994. Degradation of ochratoxin A by Acinetobacter calcoaceticus. Journal of Food Protection 57, 410–414 IARC, 1993.
IARC Monographs on the evaluation of carcinogenic risks to humans. Some naturally occurring substances: Food items and constituents, heterocyclic aromatic amines and mycotoxins, International Agency for Research on Cancer, Lyon, France, 56, 397–444; 445–466 and 467–488.
Ippolito A., A.E. Ghaouth, C.L. Wilson and M. Wisniewski, 2003. Control of postharvest decay of apple fruit by Aureobasidium pullulans and induction of defense responses. Phytopathology 93 (3), 344-348.
Janisiewicz, W.J. and L. Korsten, 2002. Biological control of postharvest diseases of fruits. Annual Review of Phytopathology 40, 411-441.
Kabak B. And Dobson A.D., 2009. Biological strategies to counteract the effects of mycotoxins. Food Protection 72 (9), 2006-2016.
Kabak B, A.D. Dobson and Isl Var, 2006. Strategies to prevent mycotoxin contamination of food and animal feed: A Review. Critical Reviews in Food Science and Nutrition, 593-618.
Karabulut, O.A., S. Lurie and S. Droby, 2003. Evaluation of the use of sodium bicarbonate, potassium sorbate and yeast antagonists for decreasing postharvest decay of sweet cherries. Postharvest Biology and Technology 23, 233-236.
Karlovsky P., 1999. Biological detoxification of fungal toxins and its use in plant breeding, feet, and food production. Natural Toxins 7, 1-23.
Kazi, B.A., R.W. Emmet, N. Nancarrow, D.L. Partington, 2008. Berry infection and the development of bunch rot in grapes caused by Aspergillus carbonarius. Plant Pathology 57, 301-307.
Khan, M.R., and F.M. Doohan, 2009. Bacterium-mediated control of Fusarium head blight disease of wheat and barley and associated mycotoxin contamination of grain. Biological Control 48, 42–47.
Khan, N.I., D.A. Schisler, M.J. Boechm, P.J. Slininger, and R.J. Bohast, 2001. Selection and evaluation of microorganisms for biocontrol of Fusarium head blight of wheat indicated by Gibberella zeae. Plant Disease 85, 1253–1258.
Köhl, J., W.M.L Molhoek, C.H. van der Plas and N.J. Fokkema, 1995. Suppression of sporulation of Botrytis spp. as a valid biocontrol strategy. European Journal of Plant Pathology 101, 251-259.
Köhl, J., J. Postma, P. Nicot, M. Ruocco and B. Blum, 2011. Stepwise screening of microorganisms for commercial use in biological control of plant-pathogenic fungi and bacteria. Biological Control, 57, 1-12
Leibinger, W., Breuker, B., Hahn, M., and Mengden, K. 1997. Control of postharvest pathogens and colonization of the apple surface by antagonistic microorganisms in the field. Phytopathology 87,1103-1110.
Leong, S.L., A.D. Hocking, J.I. Pitt, B.A Kazi,., R.W. Emmett, E.S. Scott, 2006. Australian research on ochratoxigenic fungi and ochratoxin A. International Journal of Food Microbiology 111, S10–S17.
Lima, G., A. Ippolito, F. Nigro, M. Salerno, 1997. Effectiveness of Aureobasidium pullulans and Candida oleophila on postharvest strawberry rots. Postharvest Biology and Technology 10, 169–178.
Line, J.E., R.E. Brackett, and R.E. Wilkinson, 1994. Evidence for degradation of aflatoxin B1 by Flavobacterium aurantiacum. Journal of Food Protection 57, 788-791
Lo, C.-T., E.B., Nelson, C.K., Hayes, and G.E. Harman, 1998. Ecological studies of transformed Trichoderma harzianum strain 1295-22 in the rhizosphere and on the phylloplane of creeping bentgrass. Phytopathology 88, 129-136.
Logrieco A. F. and A. Moretti, 2008. Between emerging and historical problems: An overview of the main toxigenic fungi and mycotoxin concerns in Europe. In: Mycotoxins: detection methods, management, public health, and agricultural trade (J.F. Leslie, R Bandyopadhyay and A. Visconti, ed.), C.A.B. International, Wallingford, UK, 139-154.
Logrieco A, A. Bottalico, G. Mulé, A. Moretti and G. Perrone, 2003. Epidemiology of toxigenic fungi and their associated mycotoxins for some Mediterranean crops, European Journal of Plant Pathology 109 (7), 645-667.
Magan N., 2006. Mycotoxin contamination of food in Europe: early detection and prevention strategies. Mycopathologia 162 (3), 45-253.
Magan N, D. Aldred, 2007. Post-harvest control strategies: minimizing mycotoxins in the food chain. International Journal of Food Microbiology 119(1-2), 131-139.
Majerus P, H. Bresch and Otteneder, H., 2000. Ochratoxin A in wines, fruit juices and seasonings. Archiv fur Lebensmittelhygiene 51, 95-97.
Marasas W.F.O., 1996. Fumonsins: history, world-wide occurrence and impact. In: Fumonosins in food (L.S. Jackson, J.W. DeVries and L.B. Bullerman ed.), Plenum Press, New York, NY, USA, 1-17.
Marasas W.F.O., J.D. Miller, R.T. Riley, and A. Visconti, 2001. Fumonisins—occurrence, toxicology, metabolism and risk assessment. In: Fusarium (B.A. Summerell, J.F. Leslie, D. Backhouse, W.L. Bryden, and L.W. Burgess ed.), Paul E. Nelson Memorial Symposium. APS Press, St. Paul, MN, USA 332-359.
Marshall E., 1983. Yellow rain experts battle over corn mold. Science 221, 526-529.
Morales H., V. Sanchis, J. Usall, A.J.Ramos, S. Marín, 2008. Effect of biocontrol agents Candida sake and Pantoea agglomerans on Penicillium expansum growth and patulin accumulation in apples. International Journal of Food Microbiology 29, 122(1-2), 61-67.
Nelson P.E., A.E. Desjardins, and R.D. Plattner, 1993. Fumonisins, mycotoxins produced by Fusarium species: biology, chemistry and significance. Annual Review of Phytopathology 31, 233-252.
Nourozian, J., H.R. Etebarian, and G. Khodakaramian, 2006. Biological control of Fusarium graminearum on wheat by antagonistic bacteria. Journal of Science Education and Technology 28, 29–38.
Nunes C., J. Usall, N. Teixidó, M. Abadias, I. Viñas, 2002. Improved control of postharvest decay of pears by the combination of Candida sake (CPA-1) and ammonium molybdate. Phytopathology 92(3), 281-7.
Nunes C., J. Usall, N. Teixido, I. Abadias, A. Asensio; I. Vinas, 2007 Biocontrol of postharvest decay using a new strain of Pseudomonas syringae CPA-5 in different cultivars of pome fruits. Agricultural and Food Science 16, 1, 56-65.
O’ Neill T.M., Y. Elad, D. Shtienberg and A. Cohen, 1996. Control of grapevine grey mould with Trichoderma harzianum T39. Biocontrol Science and Technology 6,139-146.
Ozay G. and H. Ozer, 2008. Mycotoxin problems in nuts and dried fruits from the Mediterranean basin. In: Mycotoxins: detection methods, management, public health, and agricultural trade (J.F. Leslie, R Bandyopadhyay, A. Visconti, ed.), C.A.B. International, 133-138.
Perrone G., G. Mule, A. Susca, P. Battilani, A. Pietri and A. Logrieco, 2006. Ochratoxin A production and amplified fragment length polymorphism analysis of Aspergillus carbonarius, Aspergillus tubingensis, and Aspergillus niger strains isolated from grapes in Italy. Applied and Environmental Microbiology 72, 680–685.
Pitt J.I., R.H. Cruickshank and L. Leistner, 1986. Penicillium commune, P. camembertii, the origin of white cheese moulds, and the production of cyclopiazonic acid. Food Microbiology 3, 363-371.
Plumlee K.H. and F. D. Galey, 1994. Neurotoxic mycotoxins: A review of fungal toxins that cause neurological disease in large animals. Journal of Veterinary Internal Medicine 8, 49-54.
Pusey P.L. 1989. Use of Bacillus subtilis and related organisms as biofungicides. Pesticide Science 27, 133-140.
Robiglio A, M.C. Sosa, M.C. Lutz, C.A. Lopes and M.P. Sangorrín, 2011. Yeast biocontrol of fungal spoilage of pears stored at low temperature. International Journal of Food Microbiology (in press 13 April).
Shantha T., 1999. Fungal degradation of aflatoxin B1. Natural Toxins 7, 175–178.
Schena L., F. Nigro, I. Pentimone, A. Ligorio and A. Ippolito, 2003. Control of postharvest rots of sweet cherries and table grapes with endophytic isolates of Aureobasidium pullulans. Postharvest Biology and Technology 30, 209-220.
Schena L., A. Ippolito, T. Zahavi, L. Cohen, F. Nigro and S. Droby, 1999. Genetic diversity and biocontrol activity of Aureobasidium pullulans isolates against postharvest rots. Postharvest Biology and Technology 17, 189–199.
Schisler D.A., N.I. Khan and M.J. Boehm, 2006. Bacillus species NRRL B-30212 for reducing Fusarium head blight in cereals. U.S. Patent 7, 001, 755.
Schisler D.A., N.I. Khan, M.J. Boehm and P.J. Slininger, 2002. Greenhouse and field evaluation of biological control of Fusarium head blight on durum wheat. Plant Disease 86, 1350–1356.
Schisler D.A., N.I. Khan, M.J. Boehm, P.E. Lipps, P.J. Slininger and S. Zhang, 2006. Selection and evaluation of the potential of choline-metabolising microbial strains to reduce Fusarium head blight. Biological Control 39, 497–506.
Serra R., L. Abrunhosa, Z. Kozakiewicz and A.Venâncio, 2003. Black Aspergillus species as ochratoxin A producers in Portuguese wine grapes. International Journal of Food Microbiology 88, 63-68.
Snowdon A.L., 1990. A Colour Atlas of Post-harvest Diseases and Disorders of Fruits and Vegetables. 1. General Introduction and Fruits. Wolfe Scientific, London, UK, 302 pp.
Soleas G., J.J. Yan and D.M. Goldberg, 2001. Assay of Ochratoxin A in wine and beer by High-Pressure Liquid Chromatography photodiode array and Gas Chromatography Mass selective detection. Journal of Agricultural and Food Chemistry 49, 2733-2740.
Soufleros E.H., Ch. Tricard and E.C. Bouloumpasi, 2003. Occurrence of ochratoxin A in Greek wines. Journal of the Science of Food and Agriculture 83, 173-179.
Squire R.A., 1981. Ranking animal carcinogens: a proposed regulatory approach. Science 214, 877-880.
Stefanaki I., E. Foufa, A. Tsatsou-Dritsa and Ph. Dais, 2003. Ochratoxin A concentrations in Greek domestic wines and dried vine fruits. Food Additives and Contaminants 20 (1), 74-83.
Suzuki I., M. Nomuraand and T. Morachi, 1991. Isolation of lactic acid bacteria which suppress mold growth and show antifungal action. Milchwissenschaft 70, 635-639.
Teixidó N., I. Viñas, J. Usall and N. Magan, 1998a. Control of blue mold of apples by preharvest application of Candida sake grown in media with different water activity. Phytopathology 88 (9), 960-964.
Teixidó N., I.Viñas, J. Usall, V. Sanchis and N. Magan, 1998b. Ecophysiological responses of the biocontrol yeast Candida sake CPA-1 to water temperature and pH stress. Journal of Applied Microbiology 84, 192-200.
Tjamos S.E., P.P. Antoniou, A. Kazantzidou, D.F. Antonopoulos, I. Papageorgiou and E.C. Tjamos, 2004. Aspergillus niger and Aspergillus carbonarius in Corinth raisin and wineproducing vineyards in Greece: population composition, ochratoxin A production and chemical control. Journal Phytopathology 152, 250-255.
Tjamos S.E., P.P. Antoniou and E.C. Tjamos, 2006. Aspergillus spp., distribution, population composition and ochratoxin A production in wine producing vineyards in Greece. International Journal of Food Microbiology 111, S6 -S66.
Tsitsigiannis D.I., M. Georgiadou, S. Agoritsis, G.Zakynthinos, T.H. Varzakas, S.E. Tjamos, P.P. Antoniou, M. Dimakopoulou, G. Karnavas, E.Paplomatas, S. Gianniotis and E.C. Tjamos, 2010. Ecology, epidemiology and control of Aspergillus spp. in pistachio orchards in Greece. Petria 20, 95-96.
Varga J., K. Rigo and J. Teren, 2000. Degradation of ochratoxin A by Aspergillus species. International Journal of Food Microbiology 59, 1–7.
Visconti A., M. Pascale and G. Centonze, 1999. Determination of ochratoxin A in wine by means of immunoaffinity column clean-up and high-performance liquid chromatography. Journal of Chromatography 864, 89–101.
Wilson C.H., M.E. Wisniewski, C.L. Biles, R. McLaughin, E. Chalutz, and S. Droby, 1991. Biological control of postharvest diseases of fruits and vegetables: alternatives to synthetic fungicides. Crop Protection 10, 172-177.
Yuen G.Y., S.D. Schoneweis, 2007. Strategies for managing Fusarium head blight and deoxynivalenol accumulation in wheat. International Journal of Food Microbiology 119 (1-2), 126-30.
Zahavi T., L. Cohen, B. Weiss, L. Schena, A. Daus, T. Kaplunov, J. Zutkhi, R. Ben-Arie and S. Droby, 2000. Biological control of Botrytis, Aspergillus and Rhizopus rots on table and wine grapes in Israel. Postharvest Biology and Technology 20, 115-124.
Zimmerli B. and R. Dick, 1996. Ochratoxin A in table wine and grape-juice: occurrence and risk assessment. Food Additives and Contaminants 13, 655-668.