Introduction
Animal-source feedstuffs are generally superior to plant-source ones for the growth and health of livestock, poultry, and fish (Wu 2018). This is due, in part, to the greater quantity of proteinogenic amino acids (AAs) and higher quality (the ratios and digestibilities of AAs) of the former than the latter. Traditionally, extensive research has focused on AAs that are not synthesized by animal cells and are known as nutritionally essential AAs (EAAs), including leucine, lysine, methionine, threonine, and tryptophan (Hou and Wu 2018a). However, little attention has been directed to AAs that are synthesizable in animal cells [so-called nutritionally nonessential AAs (NEAAs), such as glutamate, glutamine, glycine, proline, and 4-hydroxyproline] (e.g., Cummins et al. 2017; Dust et al. 2005; Myers et al. 2014). However, NEAAs have versatile nutritional and physiological functions and are generally more abundant in animal- than plant feedstuffs (Hou et al. 2015a). The high abundance of NEAAs in animal protein can cut the energetic and EAA costs of their de novo syntheses in animal cells and leads to improved feed efficiency in the production of animals, especially carnivorous species (Li and Wu 2018). In addition, animal-source feedstuffs contain taurine, creatine and creatine phosphate that are important anti-oxidants and participate in energy metabolism in tissues [particularly the brain, skeletal muscle, heart, and gonads of animals (Wu 2013)]. In contrast, plant-source feedstuffs lack these nitrogenous nutrients (Hou et al. 2019). Furthermore, animal-derived feedstuffs may contain polyamines that are essential for DNA and protein syntheses in mammals, birds, and other vertebrates (Kerr et al. 2017). Finally, the presence of oligopeptides [small peptides (containing 2–10 AA residues) and large peptides (containing 11–20 AA residues)] in animal-source feedstuffs may enhance their efficiency in promoting animal growth.
At present, little is known about the content of creatine, polyamines, and oligopeptides in animal-source feedstuffs, most of which are produced by the rendering and animal protein industries to convert wastes into high-quality protein sources, protect the global environment (Meeker and Hamilton 2006), and reduce carbon emissions from animal production (Wu 2018). In addition, the literature shows large variations of these substances and AAs in feedstuffs among different laboratories (e.g., Bryan 2018; Donadelli et al. 2019; Frikha et al. 2014; Kerr et al. 2017; Kim et al. 2000; Norberg et al. 2004; Renna et al. 2017; Shumo et al. 2019). These salient variations in published values might be attributable to the raw materials used (e.g., their sources, freshness and microbial contamination), processing technologies for ingredient production, and errors in AA analyses. A database on the composition of AAs and related nutrients in a variety of protein ingredients derived from terrestrial animals and fishes is much needed to guide the formulation of diets for feeding livestock, poultry, fish, shrimp and companion animals (Turchini et al. 2019; Wu 2018). To achieve this goal, we analyzed eleven common animal-source feedstuffs and, for comparison, three common plant-source feedstuffs for AAs in oligopeptides, protein, and the free pool as well as creatine, creatine phosphate, creatinine, agmatine, polyamines, and glutathione.
Materials and methods
Materials
Chicken by-product meal (CBPM), chicken visceral digest (CVD), hydrolyzed feather meal, poultry by-product meal (pet-food grade), and spray-dried poultry plasma (SDPP) were obtained from North American Renderers Association (Alexandria, VA, USA). Black soldier fly larvae meal (BSFM) was manufactured by Dr. Jefery Tomberlin (Department of Entomology, Texas A&M University, College Station, TX, USA). Fishmeal (US Menhaden), fishmeal (Peruvian anchovy), and fishmeal (Southeast Asian miscellaneous marine fishes) were obtained from Tongwei Research Institute (Chengdu, China). Spray-dried peptone from enzymes-treated porcine mucosal tissues (SDPM; ProPep WD) was obtained from International Nutrition Inc. (Omaha, NE, USA). SDPM was a by-product of heparin extraction under salt solution and was dried by a spray-dried technology without the presence of plant protein carriers. Spray-dried egg product was obtained from IsoNova Technologies LLC (Springfield, MO, USA). Plant-source feedstuffs [algae spirulina meal, soybean meal (SBM), and soy protein concentrate (SPC)] were obtained from Aquaculture Research and Teaching Facility of Texas A&M University (College Station, TX, USA).
High-performance liquid chromatography (HPLC)-grade water and methanol were purchased from Fisher Scientific (Houston, TX, USA). Balenine (β-alanyl-3-methylhistidine; ophidine), which has been reported to be present in the skeletal muscles of snakes, whales and pigs (Boldyrev et al. 2013; Carnegie et al. 1982; Wolf et al. 1968), was a gift of Dr. J. Wolf (National Institute of Health, Bethesda, MD, USA). Other materials, including proteases, HPLC columns, AA standards, agmatine, carnosine (β-alanyl-L-histidine), and anserine (β-alanyl-1-methylhistidine) were products of Sigma Chemicals (St. Louis, MO, USA).
Chemical analyses
Each food was finely ground to 0.5 mm in size before use for analysis. Dry matter (DM) content was determined by drying approximately 100 mg samples to a constant mass in a 105 °C oven. The content of crude protein, crude fat, ash (minerals), and carbohydrate in feedstuffs was determined as described by Wu et al. (2016a). Creatine and creatinine were analyzed by HPLC, as previously described by Kai et al. (1983). Creatine phosphate was converted into creatine by creatine kinase (Lamprecht and Stein 1965), followed by the HPLC analysis of creatine. The detection limits for creatine, creatinine and creatine phosphate were 3.5, 3.5, and 7 ng/g feed (as-fed basis), respectively.
For the determination of polyamines, agmatine, small peptides (carnosine, anserine and balenine), and free AAs, each feed sample (~ 200 mg) was homogenized in 2 ml of 1.5 M HClO4 (perchloric acid, PCA). The homogenate was neutralized with 1 ml of 2 M K2CO3, followed by addition of 2 ml HPLC-grade water. The whole solution was centrifuged at 600 g for 10 min, and the supernatant fluid was analyzed for polyamines and agmatine (Dai et al. 2014b), free AAs (Hou et al. 2019; Wu and Meininger 2008), carnosine and anserine (Wu et al. 2016a), and balenine (Hou et al. 2015b) using HPLC methods involving precolumn derivatization with o-phthaldialdehyde. Polyamines, agmatine, carnosine, anserine, balenine, and free AAs in samples were quantified on the basis of known amounts of standards using the Millenium-32 Software (Waters, Milford, MA, USA). The detection limits for carnosine, anserine and balenine were 0.35 ng/g feed (as-fed basis).
For determining peptide-bound plus free AAs (i.e., total AAs) in feeds (except for peptide-bound tryptophan), approximately 200 mg samples were hydrolyzed in 10 mL of 6 N HCl at 110 °C for 24 h under N2 (Dai et al. 2014a). For tryptophan analysis, approximately 100 mg samples were hydrolyzed at 110 °C for 20 h in 10 mL of 4.2 M NaOH plus 0.1 mL of 25% thiodiglycol (an antioxidant), as previously described (Dai et al. 2014a). Free AAs were extracted from each feed as described previously for the determination of polyamines. The differences in AA content between the acid or alkaline hydrolysis and the free AA fraction were taken to indicate the content of peptide-bound AAs (i.e., AAs in proteins and small plus large peptides). The PCAsoluble fraction (containing small plus large peptides and free AA) was neutralized with 1 M NaOH and the supernatant fluid (0.5 ml) was hydrolyzed in 10 mL of 6 N HCl at 110 °C for 24 h under N2. The differences in AA content before and after the acid hydrolysis were used to calculate the content of small plus large peptides. Glutamine, glutamate, asparagine, and aspartate in the proteins and peptides of feedstuffs were determined using proteases after samples were dried in an oven (105 °C) for 20 h, as we described previously (Hou et al. 2019; Li et al. 2011). Briefly, the working solution of enzymes contained the following per 1 ml of phosphate-buffered saline (pH 7.5): 2 mg pronase E (from Streptomyces griseus; Sigma Cat #P5147), 2 mg prolidase (from porcine kidney; Sigma Cat #P6675), 2 mg pyroglutamate aminopeptidase (from Bacillus amyloliquefaciens; Sigma Cat #P4669), 2 mg carboxypeptidase A (from bovine pancreas; Sigma Cat #C0261), and 2 mg aminopeptidase M (from porcine kidney; Sigma Cat #L0632). A finely grounded feed sample (~50 mg) was incubated at 25 °C for 6 h with 250 µl of phosphate-buffered saline (pH 7.5) and 50 µl of the enzyme solution. At the end of the incubation, 50 µl of 1.5 M HClO4 was added to the solution, followed by the addition of 25 µl of 2 M K2CO3 and 5 ml of HLPC water. Blanks were prepared by adding 50 µl of 1.5 M HClO4 to the assay mixture before the addition of 50 µl of the enzyme solution. All analyses of AAs were performed in triplicate for each sample using HPLC methods involving precolumn derivatization with o-phthaldialdehyde (Dai et al. 2014a). Amino acids in samples were quantified on the basis of known amounts of standards (Sigma Chemicals, St. Louis, MO, USA) using the Waters Millenium-32 Software (Zhang et al. 2019). Peptide-bound AAs were calculated as total AAs minus free AAs. The content of true proteins plus peptides was calculated on the basis of the molecular weights of AA residues (i.e., the molecular weight of an intact AA–18; Hou et al. 2019).
Statistical analysis
Values (means ± SEM) are expressed on the as-fed basis, because animal diets are generally formulated on this basis. Sample size (n=6/feed) was chosen on the basis of known variations of AAs among feeds (Li et al. 2011) and statistical power calculation with a probability of 0.9 (Jobgen et al. 2008). Log transformation of variables was performed when the variance of data was not homogenous among treatment groups, as assessed by the Levene’s test (Fu et al. 2010). Data on AA composition were analyzed by one-way analysis of variance and the Student–Newman–Keuls multiple comparison (Assaad et al. 2014). Probability values<0.05 were taken to indicate statistical significance.
Results
Content of nutrients in animal- and plant-derived feedstuffs
Results of the approximate analyses of nutrients in animal and plant-derived feedstuffs (as-fed basis) are summarized in (Table 1). The DM content of the feedstuffs varied from 88.7% for SDPM to 98.0% for chicken by-product meal. The content of crude protein was the highest in feather meal (91.3%; P<0.05) and the lowest in BSFM (42.0%; P<0.05). Spray-dried egg product had the highest content of crude fat (33.5%; P<0.05) but algae spirulina meal contained only 0.25% crude fat. The content of minerals was the highest in Southeast Asian fishmeal (21.9%; P<0.05), followed by SDPM, Menhaden fishmeal, spray-dried poultry plasma, Peruvian fishmeal, chicken visceral digest, and poultry by-product meal (pet-food grade) in descending order. The content of carbohydrate was the highest in soybean meal (36.2%; P<0.05) and the lowest in feather meal (0.15%; P<0.05).
Content of total AAs, total free AAs, total peptides, and total proteins in feedstuffs
Data on the content of total AAs (peptide-bound plus free AAs), total free AAs, total peptides, total small plus large peptides, and total proteins in feedstuffs are summarized in Table 2. Feather meal and BSFM contained the highest and lowest content of total AAs, respectively. Among animal source feedstuffs, the content of total AAs was the second-highest in spray-dried poultry plasma, followed by chicken visceral digest, Southeast Asian fishmeal, chicken by-product meal, and Peruvian fishmeal in descending order. Algae spirulina meal and soy protein concentrate also contained high content of total AAs, whereas soybean meal contained the lowest content of total AAs.
SDPM and spray-dried egg product contained the highest and lowest content of free AAs, respectively, which accounted for 39.4% and 0.37% of total AAs, respectively. The content of free AAs was the second-highest in chicken visceral digest (7.6% of total AAs), followed by Southeast Asian fishmeal (6.8% of total AAs), Peruvian fishmeal (4.9% of total AAs), BSFM (2.9% of total AAs), and Menhaden fishmeal (2.2%) in descending order. All the plant-source feedstuffs contained a low content of free AAs.
Feather meal and SDPM contained the highest and lowest content of peptide-bound AAs (i.e., AAs in proteins and small plus large peptides), respectively, which accounted for 99.5% and 60.6% of total AAs, respectively. The content of peptide-bound AAs was the second-highest in spray-dried poultry plasma (85.4%), followed by chicken visceral digest (73.7%), chicken by-product meal (67.8%), Southeast Asian fishmeal (65.6%), and Peruvian fishmeal (63.3%) in descending order. The content of peptide-bound AAs accounted for 99.0%, 98.6%, 99.1%, and 99.7% of total AAs in algae spirulina meal, soybean meal, and soy protein concentrate, respectively.
Chicken visceral digest and feather meal contained the highest and lowest content of AAs in PCA-soluble peptides (i.e., small plus large peptides), respectively, which accounted for 62.4% and 4.3% of AAs in total peptides, respectively. The content of AAs in PCA-soluble peptides was the second-highest in SDPM (50.3% of total peptide-bound AAs), followed by Southeast Asian fishmeal (20.1% of total peptide-bound AAs), chicken by-product meal (14.2% of total peptide-bound AAs), and Menhaden fishmeal (13.2% of total peptide-bound AAs) in descending order. The content of AAs in PCA-soluble peptides accounted for 7.1%, 11.0%, 7.3%, and 2.8% of total peptide-bound AAs in algae spirulina meal, soybean meal, and soy protein concentrate, respectively.
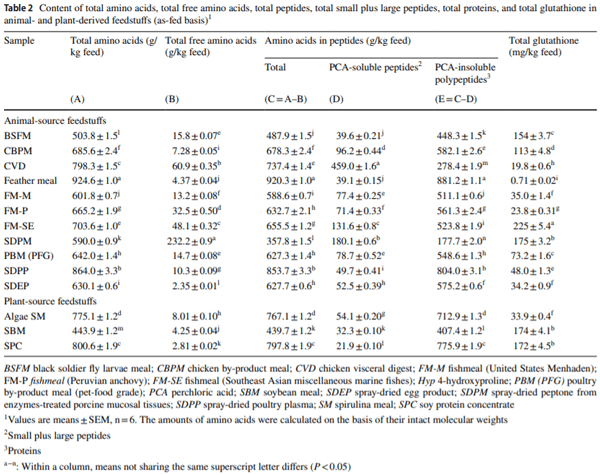
Feather meal and chicken visceral digest contained the highest and lowest content of AAs in PCA-insoluble polypeptides (i.e., proteins), respectively, which accounted for 95.7% and 37.83% of AAs in total peptides, respectively. The content of AAs in proteins was the second-highest in spray-dried poultry plasma (94.2% of total peptide-bound AAs), spray-dried egg product (91.6% of total peptide-bound AAs), Peruvian fishmeal (88.7% of total peptide-bound AAs), and chicken by-product meal (85.8% of total peptide-bound AAs) in descending order. The content of AAs in protein accounted for 92.9%, 89.0%, 92.7%, and 97.3% of total peptide-bound AAs in algae spirulina meal, soybean meal, and soy protein concentrate, respectively.
Content of total individual AAs (peptide-bound plus free AAs) in feedstuffs
Data on the content of total individual AAs (peptide-bound plus free AAs) in feedstuffs are summarized in Table 3. Tyrosine was the most abundant AA in the peptides plus the free AA pool in BSFM; glutamate in Menhaden fishmeal, Southeast Asian fishmeal, SDPM, and spray-dried egg product; glycine in chicken by-product meal, chicken visceral digest, and poultry by-product meal (pet-food grade); leucine in Peruvian fishmeal and spray-dried poultry plasma; and proline in feather. Glutamate was the second most abundant AA in the peptides plus the free AA pool in BSFM and chicken by-product meal; glycine in feather meal, Southeast Asian fishmeal, and SDPM; leucine in Menhaden fishmeal; lysine in Peruvian fishmeal and spray-dried poultry plasma; and serine in chicken visceral digest, and spray-dried egg product. Glutamate was the third most abundant AA in the peptides plus the free AA pool in chicken visceral digest, Peruvian fishmeal, poultry by-product meal, and spray-dried poultry plasma; leucine in BSFM, chicken by-product meal, SDPM, and spray-dried egg product; lysine in Menhaden fishmeal; proline in Southeast Asian fishmeal; and serine in feather meal. Alanine was the fourth most abundant AA in the peptides plus the free AA pool in BSFM; arginine in chicken by-product meal; aspartate in spray-dried poultry plasma; glycine in Menhaden fishmeal and Peruvian fishmeal; leucine in chicken visceral digest, feather meal, Southeast Asian fishmeal, and poultry by-product meal; and lysine in SDPM and spray-dried egg product. In all animal-source feedstuffs except for BSFM and Southeast Asian fishmeal, tryptophan and cysteine were the least and second least abundant AA, respectively, but the opposite was true for BSFM and Southeast Asian fishmeal, whereas 4-hydroxyproline, histidine and methionine were among the third, fourth or fifth least abundant AAs depending on feedstuffs. Similar results were obtained when values were calculated as the percentage of total AAs in the feedstuffs (Supplemental Table 1).
Glutamate was the most abundant AA in the peptides plus the free AA pool in algae spirulina meal, soybean meal, and soy protein concentrate. In algae spirulina meal, leucine was the second most abundant AA in the peptides plus the free AA pool, followed by alanine, arginine, valine and serine in descending order. In both soybean meal and soy protein concentrate, glutamine was the second most abundant AA in the peptides plus the free AA pool, followed by either leucine or arginine as the third or fourth most abundant AA.
Content of free AAs in feedstuffs
Data on the Content of free AAs in feedstuffs are summarized in Table 4. The first, second, third, fourth, and fifth most abundant free AAs in descending order were as follows: tyrosine, glutamate, glutamine, leucine, and proline in BSFM; taurine, alanine, glycine, aspartate, and leucine in chicken by-product meal; leucine, serine, proline, alanine, and arginine in chicken visceral digest; cysteine, proline, alanine, leucine, and glutamate in feather meal; taurine, alanine, leucine, lysine, and glutamate in Menhaden fishmeal; taurine, histidine, alanine, leucine, and arginine in Peruvian fishmeal; taurine, alanine, leucine, valine, and proline in Southeast Asian fishmeal; leucine, lysine, alanine, valine, and aspartate in SDPM; taurine, alanine, glycine, glutamate, and aspartate in poultry by-product meal (pet-food grade); glutamate, taurine, alanine, arginine, and glycine in spray-dried poultry plasma; and alanine, taurine, glutamate, arginine, and aspartate in spray-dried egg product.
Glutamate was the most abundant free AA in algae spirulina meal and arginine in both soybean meal and soy protein concentrate. Alanine was the second most abundant free AA in algae spirulina meal and glutamate in both soybean meal and soy protein concentrate. The content of other free AAs in plant-derived feedstuffs was very low, compared with animal-derived feedstuffs.
Content of peptide-bound AAs in feedstuffs
Data on the content of peptide-bound AAs (i.e., AAs in proteins plus small and large peptides) in feedstuffs are summarized in Table 5. Tyrosine was the most abundant peptide-bound AA in BSFM; glutamate in Menhaden fishmeal, SDPM, and spray-dried egg product; glycine in chicken by-product meal, chicken visceral digest, Southeast Asian fishmeal, and poultry by-product meal (pet-food grade); leucine in spray-dried poultry plasma; lysine in Peruvian fishmeal; and proline in feather. Glutamate was the second most abundant peptide-bound AA in BSFM, chicken by-product meal, Menhaden fishmeal, Southeast Asian fishmeal, and spray-dried egg product; glycine in feather meal and SDPM; leucine in Menhaden fishmeal, and Peruvian fishmeal; lysine in spray-dried poultry plasma; proline in poultry by-product meal (pet-food grade); and serine in chicken visceral digest and egg product. Lysine was the third most abundant peptide-bound AA in BSFM and Menhaden fishmeal; glutamine in SDPM; glutamate in chicken visceral digest, Peruvian fishmeal, SDPM, poultry by-product meal, and spray-dried poultry plasma; leucine in chicken by-product meal and egg product; lysine in BSFM and Menhaden fishmeal; proline in Southeast Asian fishmeal. Leucine was the fourth most abundant peptide-bound AA in BSFM, chicken visceral digest, and poultry by-product meal (pet-food grade); arginine in chicken by-product meal, feather meal, and SDPM; glycine in Menhaden fishmeal and Peruvian fishmeal; lysine in Southeast Asian fishmeal and egg product; and valine in spray-dried poultry plasma. In all animal source feedstuffs except for BSFM and Menhaden fishmeal, tryptophan and cysteine were the least and second least abundant AA, respectively, but the opposite was true for BSFM and Menhaden fishmeal, whereas 4-hydroxyproline, histidine and methionine were among the third, fourth or fifth least abundant peptide-bound AAs depending on feedstuffs.
Leucine was the most abundant peptide-bound AA in algae spirulina meal and glutamate in soybean meal and soy protein concentrate. In algae spirulina meal, glutamate was the second most abundant AA in their peptide-bound AA, followed by alanine, arginine, valine, serine, aspartate and glutamine in descending order. In both soybean meal and soy protein concentrate, glutamine was the second most abundant AA in their peptide-bound AA, followed by leucine, arginine, aspartate, and lysine in descending order.
Content of AAs in the PCA-soluble peptides of feedstuffs
Data on the content of AAs in the PCA-soluble peptides (i.e., AAs in small and large peptides called oligopeptides) of feedstuffs are summarized in Table 6. The patterns of AAs in oligopeptides were very different from those in total peptides (i.e., oligopeptides plus proteins, Table 7). The most noticeable differences were that, in contrast to total peptides (oligo- plus poly-peptides), (a) phenylalanine and tyrosine were the first and second least abundant AA in the oligopeptides of BSFM; (b) the content of 4-hydroxyproline was greater than that of proline in the oligopeptides of chicken by-product meal; (c) 4-hydroxyproline was barely detectable in the oligopeptides of both spray-dried poultry plasma and egg product; (d) methionine was the least abundant AA in the oligopeptides of feather meal and SDPM and was the third least abundant AA in the oligopeptides of chicken visceral digest, father meal, Southeast Asian fishmeal, SDPM, poultry by-product meal (pet-food grade), and spray-dried poultry plasma; (e) histidine was the second least abundant AA in the oligopeptides of feather meal; and (f) methionine was the least abundant AA in the oligopeptides of soybean meal and soy protein concentrate and was the fourth least abundant proteinogenic AA in the oligopeptides of algae spirulina meal.
The PCA-soluble fraction in all of the animal-source feedstuffs contained various amounts of carnosine (Table 8). Except for the three fishmeal feedstuffs and BSFM, all of the analyzed animal-source feedstuffs contained various amounts of anserine (Table 8). Among the ten animal source feedstuffs, the content of carnosine was the highest (P<0.05) in chicken by-product meal, followed by spray-dried poultry plasma, SDPM, Peruvian fishmeal, and poultry by-product meal (pet-food grade) in descending order. The content of anserine was the highest (P<0.05) in chicken by-product meal, followed by poultry by-product meal (petfood grade), spray-dried poultry plasma, SDPM, and chicken visceral digest in descending order. Carnosine and anserine were absent from the plant-source feedstuffs (Table 8). All of the animal- and plant-source feedstuffs analyzed in the present study did not contain balenine.
Alanine was the most abundant AA in the oligopeptides of Menhaden fishmeal; glutamate in the oligopeptides of BSFM and SDPM; glycine in chicken by-product meal, chicken visceral digest, Peruvian fishmeal, Southeast Asian fishmeal, poultry by-product meal (pet-food grade), and egg product; and serine in feather meal and spray-dried poultry plasma. Glutamine was the second most abundant AA in the oligopeptides of BSFM; glutamate in the oligopeptides of chicken by-product meal, chicken visceral digest, Peruvian fishmeal, Southeast Asian fishmeal, poultry by-product meal (pet-food grade), and spray-dried poultry plasma; and glycine and proline in the oligopeptides of SDPM and feather meal, respectively. Glycine was the third most abundant AA in the oligopeptides of BSFM, feather meal and Menhaden fishmeal; serine in the oligopeptides of chicken visceral digest and Peruvian fishmeal; as well as alanine, arginine, glutamate, leucine, and proline in the oligopeptides of chicken by-product meal, poultry by-product meal (pet-food grade), egg product, spray-dried poultry plasma, and Southeast Asian fishmeal, respectively. Serine was the fourth most abundant AA in the oligopeptides of BSFM and Menhaden fishmeal; arginine in the oligopeptides of chicken by-product meal and feather meal; alanine in the oligopeptides of Peruvian fishmeal, Southeast Asian fishmeal, poultry by-product meal (pet-food grade), and spray-dried poultry plasma; leucine in the oligopeptides of chicken visceral digest and egg product; and aspartate in the oligopeptides of SDPM. In all animal-source feedstuffs except for Southeast Asian fishmeal and poultry by-product meal (pet-food grade), the content of tryptophan in the oligopeptides was lower (P<0.05) than the content of cysteine, but the opposite was true for Southeast Asian fishmeal and poultry by-product meal (pet-food grade).
Glutamate was the most abundant AA in the oligopeptides of algae spirulina meal, and serine in the oligopeptides of soybean meal and soy protein concentrate. In algae spirulina meal, serine was the second most abundant AA in their oligopeptides, followed by aspartate, alanine, glutamine, threonine, asparagine, and leucine in descending order. In both soybean meal and soy protein concentrate, glutamate was the second most abundant AA in their oligopeptides, followed by glutamine, glycine, and aspartate in descending order.
Content of AAs in the PCA-insoluble peptides of feedstuffs
Data on the content of AAs in the PCA-insoluble polypeptides (i.e., proteins) of feedstuffs are summarized in Table 7. For all animal-source feedstuffs except for BSFM, chicken by-product meal, chicken visceral digest, and SDPM, the patterns of AA in proteins of the analyzed feedstuffs were generally similar to those in the total peptides (i.e., oligopeptides plus proteins, Table 7). Tyrosine was still the most abundant AA in the proteins of BSFM, lysine, leucine, alanine, and valine were the second, third, fourth, and fifth most abundant AA in the proteins of this feedstuff, respectively. Leucine, glutamate, glycine, proline, and lysine were the first, second, third, fourth, and fifth most abundant AA in the proteins of chicken by-product meal, respectively. Lysine, glycine, leucine, valine and proline were the first, second, third, fourth, and fifth most abundant AA in the proteins of chicken visceral digest, respectively. Finally, glycine, lysine, leucine, glutamine, and arginine were the first, second, third, fourth, and fifth most abundant AA in the proteins of SDPM, respectively.
Content of agmatine and polyamines in feedstuffs
Data on the content of agmatine and polyamines in feedstuffs are summarized in Table 9. BSFM, chicken visceral digest. Per gram basis, Peruvian fishmeal, Southeast Asian fishmeal, and SDPM contained much greater (P<0.05) amounts of agmatine than algae meal, soybean meal, and soy protein concentrate. For animal-source feedstuffs, the total content of polyamines was the highest (P<0.05) in SDPM, followed by Peruvian fishmeal, Southeast Asian fishmeal, poultry by-product meal, and chicken by-product meal in descending order.
Content of creatine, creatinine, and creatine phosphate in feedstuffs
Data on the content of creatine, creatinine, and creatine phosphate in feedstuffs are summarized in Table 8. For all animal-source feedstuffs tested, the content of creatine, creatinine, and creatine phosphate was the highest (P<0.05) in Peruvian fishmeal. The content of creatinine was the second-highest in Southeast Asian fishmeal, followed by Menhaden fishmeal, SDPM, poultry by-product meal, chicken by-product meal, and chicken visceral digest, and BSFM in descending order. The content of creatine phosphate was the second-highest in Southeast Asian fishmeal, followed by Menhaden fishmeal, SDPM, chicken visceral digest, BSFM, and poultry by-product meal (pet-food grade) in descending order. The content of all creatine moieties was the highest (P<0.05) in Peruvian fishmeal, followed by Menhaden fishmeal, Southeast Asian fishmeal, SDPM, and chicken visceral digest in descending order. Algae spirulina meal, soybean meal, and soy protein concentrate did not contain creatine, creatinine, or creatine phosphate.
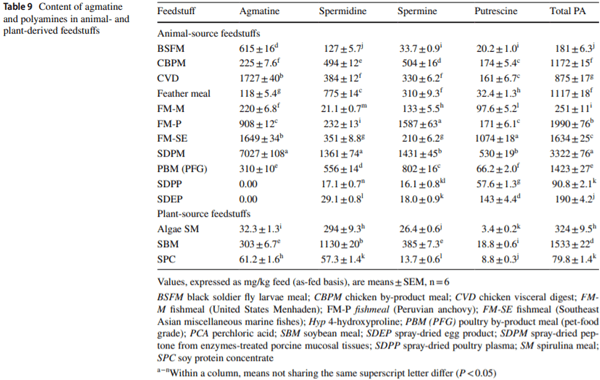
Content of total glutathione in feedstuffs
Data on the content of total glutathione in feedstuffs are summarized in Table 2. Among the analyzed animal-source feedstuffs, this tripeptide was most abundant in Southeast Asian fishmeal, followed by SDPM, BSFM, chicken by-product meal, poultry by-product meal (pet-food grade), spray-dried poultry plasma, and Menhaden fishmeal in descending order. The content of total glutathione in soybean meal and soy protein concentrate was similar to that in SDPM. Algae also contained a significant amount of glutathione.
Discussion
Adequate provision of AAs in diets is essential for the maximal growth and production performance as well as optimal health of all farm animals, including swine, poultry, ruminants, fish, and shrimp (Baker 2009; Li et al. 2007; Sulabo et al. 2013; Wu 2018; Yi et al. 2018; Zhang et al. 2015). Although AAs had long been classified as nutritionally essential or nonessential, the term “NEAA” has now been recognized as a misnomer in nutritional sciences (Hou and Wu 2017). Emerging findings have revealed the importance of NEAAs, such as glycine, proline, glutamate, glutamine and 4-hydroxyproline, in improving intestinal health and whole-body growth in animals (Fan et al. 2019; Le Floch et al. 2018; Li and Wu 2018; Wu et al. 2019). We have shown that glycine, proline and glutamate are the first, second, and third most abundant AAs in animal tissues, respectively (Wu 2013), supporting the notion that mammals, birds and other vertebrates have particularly high requirements for these nutrients (Hou and Wu 2018b; Liu et al. 2019; Meléndez-Hevia et al. 2009; Wu et al. 2014). Despite the immunological, nutritional and physiological importance of tryptophan, the content of this AA in feedstuffs was often not analyzed due to technical difficulties (e.g., Bryan 2018; Dozier et al. 2003; Jamdar and Harikumar 2008; Ravindran et al. 2005; Renna et al. 2017; Shumo et al. 2019). To our knowledge, this is the first report of all proteinogenic AAs, key nonproteinogenic AAs (taurine, β-alanine, ornithine, and citrulline), agmatine, polyamines, creatine, creatine phosphate, creatinine and glutathione in animal-source feedstuffs for guiding the formation of animal diets. Our findings are expected to shed light on the functionality of animal-derived feedstuffs in the production of farm animals, particularly carnivorous species.
Because of limited knowledge about AA biochemistry and nutrition, both researchers and animal producers had paid little attention to the content of NEAAs (peptide-bound plus free AAs) in feedstuffs, including animal-source feedstuffs produced by the rendering industry (e.g., Cummins et al. 2017; Dust et al. 2005; Myers et al. 2014). In studies that reported the content of some NEAAs in feedstuffs, no data were provided for glutamate, glutamine, aspartate or asparagine because the acid hydrolysis of the feedstuffs converted glutamine into glutamate and asparagine into aspartate. A recent study even showed that glutamate and glutamine were absent from proteins in the meal prepared from black soldier flies reared on chicken manure (Shumo et al. 2019). To our knowledge, this is the first detailed report of the high abundance of glutamine, glutamate, asparagine and aspartate in rendered animal feedstuffs (Tables 3–7). Our current work also provided much-needed data on glycine, proline, 4-hydrocyproline, and taurine in animal-source feedstuffs, the AAs that had often been ignored in most studies (e.g., Cummins et al. 2017; Dust et al. 2005; Klemesrud et al. 1997; Myers et al. 2014; Shumo et al. 2019; Supplemental Tables 2-5).
Published data on the content of NEAAs (peptide-bound plus free AAs) in feedstuffs showed exceedingly large variations by up to three magnitudes despite their similar content of DM or protein. For example, spray-dried egg product was reported to contain either 1.91 g glycine and 1.90 g proline per kg DM (Donadelli et al. 2019) or 16.4 g glycine and 16.7 g proline per kg DM (Norberg et al. 2004). Surprisingly, spray-dried inedible whole egg was found to contain only 0.02 g glycine/kg DM (Donadelli et al. 2019). We determined that spray-dried egg product contained 25.8 g glycine and 35.6 g proline per kg DM (Table 3). In addition, chicken by-product meal was reported to contain 6.07 g glycine, 4.13 g proline and 1.82 g 4-hydroxyproline per kg DM (Donadelli et al. 2019), whereas poultry by-product meal was reported to contain 57 g glycine and 53 g proline per kg DM (Murray et al. 1997). We determined that chicken by-product meal contained 60.6 g glycine, 43.9 g proline and 18.9 g 4-hydroxyproline per kg DM and that poultry by-product meal contained 70.9 g glycine, 52.4 g proline and 23.2 g 4-hydroxyproline per kg DM (Table 3). Furthermore, BSFM was reported to contain 0.55 g cysteine and 23.2 g glycine per kg DM (Renna et al. 2017), 2.8 g cysteine and 19 g glycine per kg DM (Kawasaki et al. 2019), or 7.6 g cysteine and 29.2 g glycine per kg DM (Lei et al. 2019). In marked differences from porcine and poultry byproducts (Wu 2013), the content of 4-hydroxyproline in the meals from black soldier flies reared on chicken manure was reported to be 5.1 times that of proline (Shumo et al. 2019). We determined that BSFM contained 7.23 g cysteine, 30.5 g glycine, 30.6 g proline, and 13.4 g 4-hydroxyproline per kg DM (Table 3). A major difference in NEAAs between the animal- and plant-source feedstuffs analyzed in the present study is that the former contained a higher content of glycine and proline than the latter. These two NEAAs are nutritionally essential for the maximal growth and feed efficiency of poultry (Baker 2009), piglets (Wu et al. 2011), and fish (Li et al. 2009). Additionally, 4-hydroxyproline, along with glycine (Fan et al. 2019), protects the intestine from oxidative stress (Wu et al. 2019) and enhance the growth performance of animals, including fish (Aksnes et al. 2008; Liu et al. 2014). This may explain, in part, why gut damage often occurs in animals such as pigs (Wu et al. 2014) and fish (Daniel 2018) that fed diets containing plant ingredients as the sole source of protein. The complete information on the content of all proteinoenic AAs and key nonproteinoenic AAs in animal-derived feedstuffs from the present study is very useful to guide the formulation of diets for animals.
Like NEAAs, the content of EAAs in animal-source feedstuffs also varied greatly in the published studies by up to tenfold or greater despite their similar content of DM or protein. For example, spray-dried egg product was reported to contain either 4.67 g leucine and 4.14 g lysine per kg DM (Donadelli et al. 2019) or 44.1 g leucine and 35.8 g lysine per kg DM (Norberg et al. 2004). We determined that spray-dried egg product contained 48.5 g leucine and 43.0 g lysine per kg DM (Table 3). In addition, chicken by-product meal was reported to contain either 5.10 g leucine and 4.59 g lysine per kg DM (Donadelli et al. 2019) or 44.9 g leucine and 40.6 g lysine per kg DM (Dust et al. 2005), whereas poultry by-product meal was reported to contain either 46 g leucine and 38 g lysine per kg DM (Murray et al. 1997), or 31.3 g leucine and 30.4 g lysine per kg DM (Dozier et al. 2003). We determined that the chicken by-product meal contained 53.9 g leucine and 46.1 g lysine kg DM and that poultry by-product meal contained 44.7 g leucine and 36.6 g lysine per kg DM (Table 3). Furthermore, chicken visceral meal was reported to contain 12.5 g isoleucine and 15.0 g valine per kg DM (Djissou et al. 2018), 37 g isoleucine and 58 g valine per kg DM (Jamdar and Harikumar 2008), or 15 g isoleucine and 19 g valine per kg DM (Murray et al. 1997). It is unknown whether AA content in chicken visceral meal is affected by pre-mortem feeding. We determined that chicken viscera meal contained 41.2 g isoleucine and 59.7 g valine per kg DM (Table 3). Results of our work showed that leucine [an activator of the mechanistic target of rapamycin signaling to promote protein synthesis (Suryawan et al. 2020)] was the most abundant AA in all of the analyzed animal-source feedstuffs (Table 3), again underscoring their important roles in improving animal growth. Because the addition of animal-derived feedstuffs to a typical corn- and soybean meal-based diet improves the growth performance of the young pig (Hou et al. 2017; Wu 2018), their high content of leucine confers a nutritional benefit and does not result in an imbalance among dietary branched-chain AAs.
A novel observation from the present work is that tyrosine was the most abundant peptide-bound and free AA in BSFM (Tables 3 and 7). The content of tyrosine in BSFM was previously reported to be 23 g/kg DM (Kawasaki et al. 2019) or not reported at all (Dumas et al. 2018; Spranghers et al. 2017). Interestingly, tyrosine is actively synthesized from phenylalanine and accumulated in insects for cuticle hardening and for defense against pathogens (Vavricka et al. 2014). As the precursor of melanin that determines the pigment of skin, BSFM may be particularly useful to maintain desirable colors in animals (e.g., fish, dogs and cats).
Peptide nutrition has received much attention in recent years because small and large peptides appear to have a greater rate of digestion and absorption in animals than intact proteins (Hou et al. 2017). To our knowledge, this is the first study to determine the content of AAs in the oligopeptides and proteins of animal-source feedstuffs. Except for chicken visceral meal and SDPM, all other animal-source feedstuffs contained more proteins than oligopeptides. Most AAs were present as oligopeptides in chicken visceral meal, whereas AAs were equally distributed between oligopeptides and proteins in SDPM (Table 2). In addition, a significant proportion of AAs existed as oligopeptides in Southeast Asian fishmeal, likely because proteases were used to prepare this feedstuff. For feedstuffs with a high proportion of oligopeptides, it is possible that their inclusion rates in animal diets can be reduced, as compared with feedstuffs with a high proportion of intact proteins. This can economically spare the use of high-quality protein sources as feed additives. Of particular note, chicken by-product meal and poultry by-product meal (pet-food grade) contained large amounts of carnosine and anserine (Table 8). Appreciable amounts of carnosine and anserine were also present in spray-dried poultry plasma [likely released from red blood cells (Ng and Marshall 1976)] and SDPM. The different amounts of carnosine in Menhaden, Peruvian and Southeast Asian fishmeals reflect the different sources of fish used to manufacture the feedstuffs (FAO 2018). For example, the skeletal muscle of 50-g largemouth bass contained carnosine (43.6 ± 0.91 mg/kg of wet tissue; mean ± SEM, n = 6) but no detectable anserine (Wu G, unpublished data). Interestingly, all these three fishmeals did not contain a detectable amount of anserine, which is absent in humans and some animals including fishes (Boldyrev et al. 2013). Both carnosine and anserine are potent antioxidants (Boldyrev et al. 2013), their presence in the animal-source feedstuffs is expected to play an important role in protecting the small intestine from oxidative stress and inflammation, thereby improving intestinal health and function.
Both animals and plants contain agmatine, polyamines and glutathione (Baratella et al. 2018; Blachier et al. 2011; Lenis et al. 2018). There is little information about the content of these nitrogenous substances, creatine, creatine phosphate, creatinine, or glutathione in feedstuffs. Along with ornithine, agmatine is a precursor for the synthesis of polyamines in animal cells and plays a role in maintaining mitochondrial membrane permeability (Akasaka and Fujiwara 2019; Martinis et al. 2019). Bryan (2018) reported that the presence of significant amounts of agmatine (mg/kg DM): fishmeal, 240; feather meal, 0.68; and soybean meal, 17.1. The values of agmatine content in the fishmeal used by Bryan (2018) were similar to those we found for Menhaden fishmeal but the values in feather meal and soybean meal were substantially lower than those we found for feather meal and soybean meal, which were 125 and 338 mg/kg DM, respectively (Table 9). To our knowledge, this is the first report of a greater abundance of agmatine in chicken visceral meal and SDPM than in other animal-source feedstuffs. Polyamines are substances are essential to DNA and protein syntheses in all cell types (Provenzano et al. 2019) and to energy metabolism in excitable tissues (the brain and skeletal muscle; Brosnan and Brosnan 2007). Bryan (2018) reported that the content of putrescine in fishmeal, feather meal and soybean meal was much greater than that of spermidine or spermine, but this was not observed for all the feedstuffs used in the present study except for Southeast Asian fishmeal and spray-dried egg product (Table 9). Of note, the content of spermidine (14–137 mg/kg DM) and spermine (12–72 mg/kg DM) reported by Kerr et al. (2017) for chicken by-product meal, feather meal and poultry by-product meal was substantially lower than that we found for these types of feedstuffs (e.g., 500–804 mg spermidine/kg DM and 324–826 mg spermine/kg DM; Table 9). Among all of the analyzed feedstuffs, SDPM contained the highest level of polyamines (Table 9), and this may explain, in part, a beneficial role for this ingredient in promoting the rapid recovery of the small intestine from weaning-induced injury (Hou et al. 2017). Furthermore, the content of total polyamines was greater in animal- than algae spirulina meal and soy protein concentrate (Table 9).
Creatine may be a functional nutrient in diets for livestock, poultry and fish (Wallimann et al. 2011). In contrast to plant-source feedstuffs, creatine, creatinine, and creatine phosphate were present in significant amounts in animal source feedstuffs but were completely absent from all the plant-source feedstuffs (Table 8). These results indicate the unique importance of the former in improving the feed consumption, growth and health of animals (including companion animals). Furthermore, the simultaneous presence of a significant amount of glutathione [the major small molecular-weight antioxidant (Wu 2013)] with creatine and polyamines in animal-source feedstuffs protects the small intestine of animals from oxidative stress that often occurs under stressful conditions such as weaning, transportation, and heat stress (Wu 2018; Yang and Liao 2019). Finally, the inclusion of creatine in diets reduces the need for its endogenous synthesis of arginine, glycine and methionine due to a potent inhibition of renal arginine:glycine amidinotransferase expression (Brosnan and Brosnan 2007), thereby sparing these AAs for protein synthesis. In livestock, poultry and fish that are fed creatine-free diets, large amounts of arginine, glycine and methionine must be utilized for producing creatine, which is highly abundant in skeletal muscle. For example, in young (Wu et al. 2016b) and gestating (Wu et al. 2018) swine, creatine synthesis accounts for about 20% and 50%, respectively, of arginine catabolized in the body. There is also evidence that dietary supplementation with creatine to growing-finishing (Maddock et al. 2002; Young et al. 2007) and gestating (Vallet et al. 2013) swine improves their growth and reproductive performances. Collectively, these results indicate the unique importance of creatine in animal-source feedstuffs in improving the feed consumption, growth, health and reproduction of animals (including farm, companion and aquatic animals). Our findings will also guide the cost-effective formulation of new diets for livestock, poultry, fish and shrimp (e.g., reducing the use of fishmeal in aquafeeds) that is based on the nutrient composition and complementarity of feedstuffs, an important area of animal nutrition research (Le Floch et al. 2018; Turchini et al. 2019; Wu 2018).
Results of this study may have important implications for human nutrition. Soybean proteins have been consumed by humans for centuries in a variety of forms, including infant formulas, fours, protein isolates and concentrates, and textured components (Friedman and Brandon 2001; Singh et al. 2008). This food is popular for infants, children and adults who are allergic to cow’s milk protein. The content of most AAs was similar between SPC and egg (Table 3). Notably, the content of methionine in SPC (g/kg food) was relatively low (Gorissen et al. 2018) and was only 58% of that in eggs (Table 3). Complementary intake of animal-source protein [e.g., meat (Wu et al. 2016a) and egg] can prevent a deficiency of methionine in soy product-based diets. Likewise, algae has been part of human diets for thousands of years in many regions of the world, including Asia, Europe, South America, and North America (Wells et al. 2017). Global demands for algae as a functional food are growing due to its health benefits (Koyande et al. 2019). However, algae contained 36% less cysteine than eggs (Table 3), and this should be brought into the attention of nutritionists. Finally, insects (including black soldier fy larvae) have been used as human foods in more than 110 countries all over the world (MacEvilly 2000) and at least 2,000 insect species are considered to be edible in human diets (Jongema 2012). Edible insects provide high-quality animal protein, with their nutritional quality likely depending on their species and developmental stage (egg, larvae, pupae and adult; Roos 2018; Rumpold and Schlüter 2013). Interestingly, BSFM contained 48% more tyrosine than eggs (Table 3). Thus, under various environmental and psychological stresses, ingestion of insect proteins may be a lost-cost source of tyrosine for the syntheses of dopamine and norepinephrine in the brain to improve the adaptation, cognitive performance, and neurological function of humans (Lieherman 1994; Wurtman et al. 1981). This may also be applied to farm animals when they are faced with a variety of stress factors, such as weaning, lactation, pregnancy, and exposure to low temperatures.
In conclusion, we analyzed all peptide-bound and free AAs, as well as agmatine, polyamines, creatine, carnosine, anserine, and glutathione, in animal- and plant-source feedstuffs. Compared with plant-source feedstuffs, most of the animal-source feedstuffs contained much larger amounts of glycine, proline and 4-hydroxyproline. All feedstuffs contained significant amounts of agmatine, polyamines, and glutathione. Chicken by-product meal, poultry by-product meal, and spray-dried poultry plasma contained large amounts of carnosine and anserine. In contrast, taurine, creatine, creatinine, and creatine phosphate were present in significant amounts in all animal-derived feedstuffs but were absent from plant-derived feedstuffs. These results indicate the unique importance of animal-source feedstuffs in improving the feed efficiency, growth and health of animals (including companion and aquatic animals). Because soy protein concentrate is consumed by infants, children and adults, as are BSFM and algae for children and adults, our findings also have important implications for human nutrition.
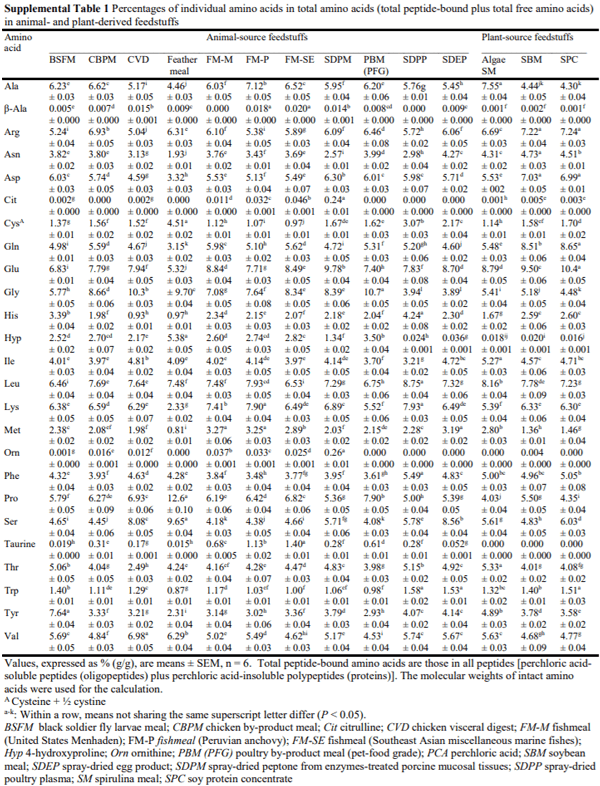
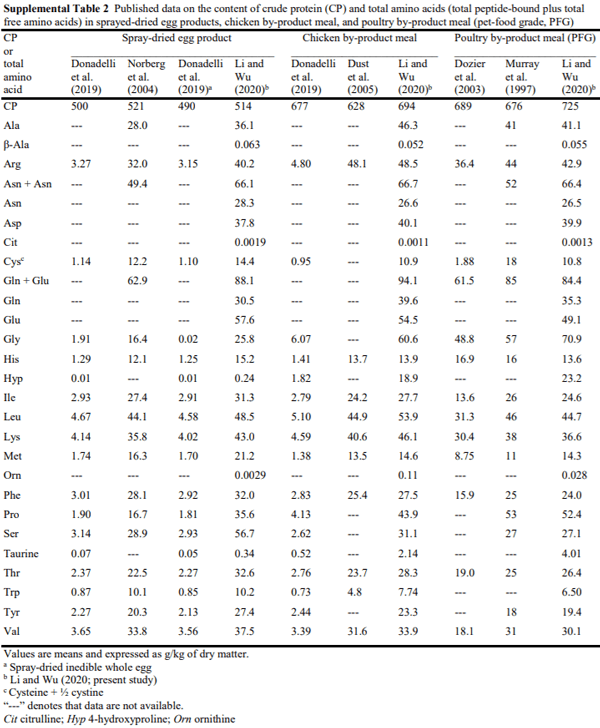
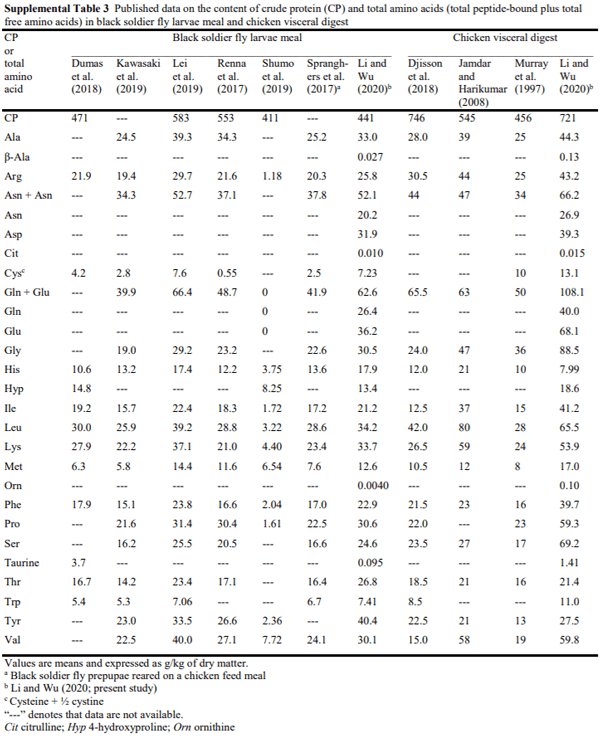
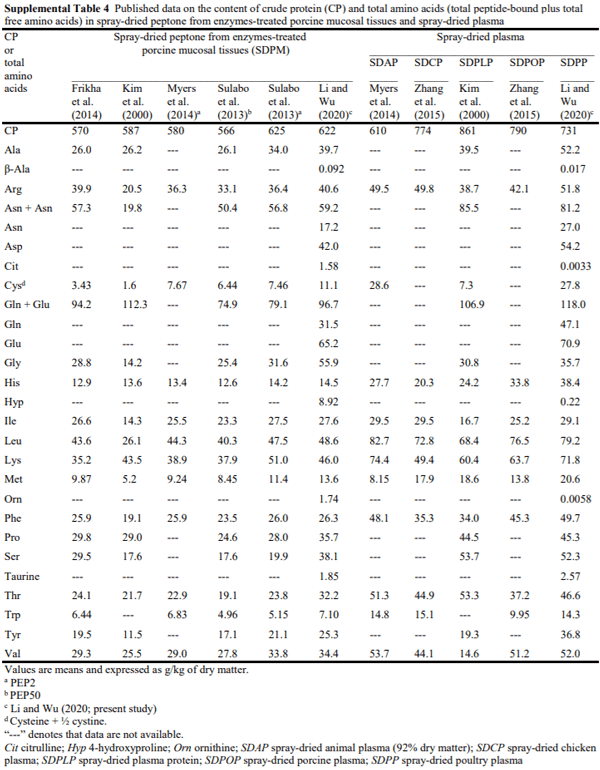
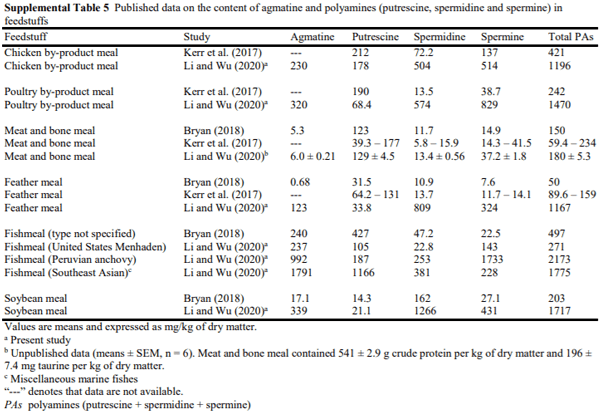