DESCRIPTION OF PROBLEM
A principal goal of nutritionists is to provide birds with a balanced diet that maximizes production at the least cost. Meat bird performance is maximized when feeding pelleted diets (Patton et al., 1937; Hussar and Robblee, 1962; Jensen et al., 1962). However, the nutritional value of feed presented to birds in feed pans throughout the house may not align with diet formulation due to mixer uniformity, feed line length, pellet degradation, and subsequent nutrient segregation. When considering the homogeneity of nutrients in animal feed, a great deal of emphasis is placed on the mixing process. However, post-mixing processes can also impact nutrient distribution, owing to the importance of understanding how nutrients segregate from the feed mill to the feed pan.
Pellet degradation can occur at the feed mill, during transportation, within feed bins, and throughout feed lines during feed augering (Mortiz, 2013). On-farm segregation of pellets and fines, as well as ingredients (i.e., phytase) and nutrients, have been previously documented (Scheideler, 1991; Moritz, 2013; Sellers et al., 2020; Poholsky et al., 2021). Uneven distribution of nutrients throughout the house may impact flock performance and uniformity. Sellers et al. (2020) fed diets collected from various locations of a commercial feed line to broilers from 28 to 56 d of age. The authors reported variations in phytase activity and percentage of pellets due to feed pan location and consequent effects on BW uniformity. Monitoring nutrient distribution throughout the house may be an important feed quality assurance control point to troubleshoot flock performance and uniformity concerns.
Recently, a series of field experiments were conducted by Penn State University to assess nutrient variability in commercial broiler houses by considering 4 scenarios: low pellet-to-fine ratio (P:F) feed (65:35) in both long (152 m) and short (76 m) feed lines, and high P:F feed (80:20) in the same long and short feed lines (Poholsky et al., 2021). Augering low P:F feed throughout 152 m feed lines resulted in the greatest degree of variability in amino acid concentrations and phytase activity throughout the house. On the other hand, there was no evidence of nutrient segregation after augering high P:F feed throughout 76 m feed lines. These results indicate that nutrient segregation is impacted by P:F and feed line length. However, these data only represent one snapshot in time. Monitoring feed flow and nutrient distribution over time is necessary to better understand nutrient segregation and its association with flock uniformity and production economics. Therefore, the current study was conducted to determine if on-farm nutrient segregation was apparent when high P:F feed was augered through short feed lines over 5 consecutive days. The research objectives were to monitor on-farm nutrient segregation across a 5-d period and to establish an appropriate on-farm feed sampling procedure by comparing 2 feed sample collection techniques.
MATERIALS AND METHODS
Overview of Field Research Conditions
These studies were conducted at a local commercial turkey farm in Pennsylvania. Hen turkeys were 12 wk of age and were provided a commercially formulated grower diet throughout the sample collection period, as part of a standard grow-out. Diet composition was proprietary, but it was formulated to meet or exceed Aviagen hen turkey nutrient recommendations. The diet was manufactured at a commercial feed mill where raw ingredients were mixed in a Hayes and Stolz twin shaft, single ribbon mixer with a 3.175-tonne capacity. Pellets were generated using a 200-HP Sprout 615 pellet mill equipped with a 4.37 £ 50.80 mm effective die. Importantly, phytase was added to the mixer before pelleting, whereas all soybean oil was added post-pelleting. The 91 m house was equipped with two 75 m feed lines. Herein, feed lines spanning 0 to 100 m in length are considered short and feed lines longer than 100 m are considered long. The feed lines were conceptually segmented into 5 feed pan locations (FPL) for sample collection, each location representing 15 m of the line.
Feed Sampling Procedure
The study consisted of 2 concurrent experiments that compared different on-farm feed sampling techniques. In experiment 1, feed particle and nutrient variability throughout the house were evaluated by collecting residual feed from feed pans in each FPL. A different feed sample collection technique was employed in experiment 2 where feed pans were emptied and filled with fresh feed from the lines before collection. Feed lines were raised prior to sample collection to restrict bird access to feed.
Experiment 1.Predetermined feed pans in each FPL along the feed lines were designated for the collection of residual feed (FPL: 7.5 −15, 22.5−30, 37.5−45, and 53.5−60 m). These samples represented unconsumed feed that was left in the feed pans (Figure 1A). On each day at 8:00 am, the feed lines were raised, and residual feed samples were collected from a single feed pan in each FPL. Residual feed samples were collected in approximately 45 min from new, unsampled feed pans equally spaced along the feed line each day (Figure 2).
Figure 1. (A) Photo of residual feed collected for experiment 1. This feed represents what is left over in the feed pans after the birds have consumed the pellets. (B) Photo of feed sampled in experiment 2. Designated feed pans were first emptied of residual feed and then filled with fresh feed dropping in from the feed lines before collection.
Figure 2. Diagram representing the feed pans designated for sample collection throughout the 5-d experimental period. In experiment 1, residual feed was collected from a single feed pan within each feed pan location (FPL) along the feed lines, resulting in 4 feed samples collected per feed line per day. On each day, a different feed pan was sampled for residual feed per FPL. For experiment 2, feed from the first 5 feed pans in each FPL was collected daily by emptying the feed pans and calling for feed.
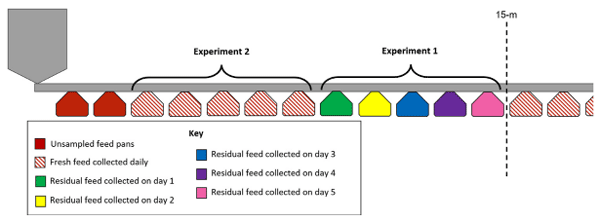
Experiment 2.Feed samples were collected from the first 5 feed pans within each 15 m portion of the feed lines each day (FPL: 0−7.5, 15−22.5, 30−37.5, 45−53.5, and 60−75 m; Figure 2). Samples were collected from one feed line at 9:30 am each day. The 1.5 h procedure for collecting feed was as follows: Feed lines were raised prior to sample collection to restrict bird access to feed. The designated feed pans were first emptied. Next, the feed line motor was turned on to fill the empty feed pans which were then collected for analysis (Figure 1B).
Feed Analysis
Experiment 1.All residual feed samples were passed through a modified particle size separator (Hofstetter and Boney, 2021) fitted with a No. 5 American Society for Testing and Materials screen (W.S. Tyler Industrial Group, Mentor, OH) at random to determine the P:F. Thereafter, the pellets and fines from each feed pan were combined and ground to an average particle size of 300mm using a Bunn Coffee Grinder (Bunn-O-Matic Co., Springfield, IL). Gross energy was analyzed using a Parr 6200 isoperibol bomb calorimeter (Parr Instrument Co., Moline, IL). Ground feed samples were then analyzed for predicted crude protein, crude fat, crude fiber, and ash using a handheld nearinfrared spectrophotometer (NIRS; AB Vista, Marlborough, Wiltshire, UK) with a calibration for turkey grower feed.
Experiment 2. Feed samples were analyzed for P:F, gross energy, and NIRS-predicted proximates using the same methods as described above. In addition, feed samples within each FPL were placed in a common tote. Composite feed samples were collected for analysis of phytase activity (AOAC, 2000), total amino acids (AOAC, 2005), and mineral (Cu, Mn, Zn) concentrations (AOAC, 1985) at commercial laboratories (Eurofins Scientific, Des Moines, IA, University of Missouri Agricultural Experiment Station Chemical Laboratory, and Central Analytical Laboratory, Fayetteville, AR, respectively).
Statistical Analysis
Data were analyzed by one-way repeated measures ANOVA using the MIXED procedure of SAS 9.4 statistical software (SAS Inst. Inc., Cary, NC). Feed pan location, Day of Collection (DoC), and FPL £ DoC were included in the model. Significant means were further separated using a Tukey’s adjustment when P ≤ 0.05.
RESULTS AND DISCUSSION
Experiment 1
Factors FPL and DoC did not interact (P > 0.05). Feed pan location did not impact the percentage of pellets in the residual feed (P = 0.212; Table 1). Although the percentage of pellets varied by DoC (P = 0.043; Table 1), the average amount of pellets in the house throughout the experimental period was 27%. It was apparent that birds preferentially selected and consumed pellets, which is well supported in the literature (Schiffman, 1968; Portella et al., 1988).
Table 1. Effects of FPL1 and DoC2 on pellets3, fines3, NIRS-predicted nutrients, and gross energy of residual feed (experiment 1).
Variations in NIRS-predicted nutrients and gross energy across FPL were not observed (P > 0.05; Table 1). In addition, nutrient composition of the residual feed was the same on each collection day (P > 0.05; Table 1). These data demonstrate that birds leave feed fines behind and consume the feed dropping into feed pans each day. This should be considered when poor quality feed complaints are received. Allowing birds to clean up feed pans or emptying feed pans prior to calling for feed will allow for more accurate feed sample collection.
Experiment 2
Factors FPL and DoC did not interact (P > 0.05). The percentage of pellets in feed pans varied by 31-percentage pts across FPL (P < 0.001; Table 2), increasing as feed traveled from the hopper toward the feed line motor. Previous work has also demonstrated an increase in percent pellets as feed travels throughout the feed line (Scheideler, 1991; Moritz, 2013; Poholsky et al., 2021). In contrast, Sellers et al. (2020) reported that the amount of pellets present in the feed pans decreased as feed was augered from 0 to 55 m. This observation may be due in part to the fill level of the feed bin, the time at which samples were taken, and feed line length. It is also important to note that previous reports showed segregation of pellets throughout the house when using feed qualities ranging from 55 to 80% pellets (Sellers et al., 2020; Poholsky et al., 2021), indicating that some degree of pellet segregation will occur regardless of the starting pellet quality.
The percentage of pellets was affected by DoC, varying by 8-percentage pts when comparing d 1 and 2 (P < 0.001; Table 2). This may be explained by conditions inside the feed bin. Winowiski (2017) showed that the feed bin is a dynamic environment, leading to segregation of pellets and fines during discharge. In the current study, feed samples collected across each day represent feed discharged from different sections of the feed bin.
Predicted crude fat varied by 3.6% across FPL (P = 0.038; Table 2). Figure 3 shows that crude fat percentage was highest when the greatest amount of fines were present in the feed pan and lowest when the least amount of fines were present. Results may be influenced by the fat application technique utilized during feed manufacture. Post-pellet liquid ingredient application systems are used in the industry to maintain pellet quality by reducing or completely eliminating the amount of oil added to the mixer. However, this system may cause an uneven distribution of fat across pellets and fines. Pope et al. (2018) applied 3.5% liquid fat to diets post-pelleting and observed that fines absorbed more fat and exhibited greater gross energy compared to pellets. These authors theorized that liquid fat may preferentially coat fines as opposed to pellets since fines have a greater surface area. Although crude fat segregation was apparent in the current study, the difference did not contribute to variation in gross energy across FPL (P > 0.05; Table 2).
Table 2. Effects of FPL1 and DoC2 on pellets3, fines3, NIRS-predicted nutrients, gross energy, and phytase activity4 (experiment 2).
Feed pan location and DoC did not influence predicted crude protein, crude fiber, or ash concentrations (P > 0.05; Table 2). The authors expected to observe no evidence of nutrient segregation when augering high P:F feed in short feed lines based on previous findings (Poholsky et al., 2021).
In addition, phytase activity did not vary across FPL or DoC (P = 0.629; Table 2). A uniform distribution of phytase throughout the house may be related to mixer-added phytase application. Segregation of phytase in poultry houses when the enzyme was applied post-pelleting is well documented (Moritz, 2013; Wamsley, 2014; Poholsky et al., 2021). Sellers et al. (2020) reported a significant degree of variability in phytase activity (lowest: 1,603 FTU/kg; highest: 2,956 FTU/kg) throughout the feed line when phytase was added post-pelleting. In contrast, phytase activity was not different throughout the feed line when the enzyme was added at the mixer (Sellers et al., 2020). Results from the current study support uniform distribution of phytase activity and subsequent phosphorus release when a thermostable phytase is added at the mixer.
Proline was the only amino acid concentration that varied by FPL (P = 0.050; Table 3). Amino acids did not vary by DoC (P > 0.05; Table 3). These results align with previous on-farm nutrient segregation findings where the same amino acids were analyzed and segregation was not apparent after augering high P:F feed throughout short feed lines (Poholsky et al., 2021).
Figure 3. Variation in pellet-to-fine ratio and predicted crude fat concentration throughout a commercial poultry house (experiment 2). Feed lines in the house were divided into 5 feed pan collection locations (FPL), each representing 15 linear meter portions of the feed line. Pellet-to-fine ratios were determined by sifting feed using a modified particle size separator (Modified Penn State Particle Separator, Agricultural and Biological Engineering Department, University Park, PA) fitted with a No. 5 American Society for Testing and Materials screen. Crude fat concentration of feed was predicted using a handheld NIRS with a calibration for turkey grower diet.
Table 3. Effects of FPL1 and DoC2 on total amino acid concentrations3 (experiment 2).
Feed quality control can be enhanced at the feed mill. Adequate mixing is essential for mash feed homogeneity prior to pelleting. A variety of mixer profile analyses have been assessed and are commonly used (Clark et al., 2007; Rocha et al., 2015). A common analysis considers high density minerals that are provided in low concentrations in the diet where chemical analysis of 10 samples obtained from a single batch of feed is used to evaluate mixer uniformity (ASAE, 1990). For these reasons, copper (Cu), manganese (Mn), and zinc (Zn) were selected for analysis in the current study as possible markers for on-farm nutrient segregation.
Mineral concentrations (Cu, Mn, and Zn) were not influenced by FPL (P > 0.05; Table 4), which aligned with the lack of amino acid segregation when high P:F feed was augered through short feed lines. Copper concentration was influenced by DoC (P = 0.009; Table 4), likely associated with feed bin dynamics. However, the interferences associated with inductively coupled plasma-optical emission spectroscopy (ICP-OES) should be considered when interpreting these data. Spectral, physical, and chemical interferences are possible during ICP-OES analysis and can diminish analytical precision and accuracy, particularly when determining trace element concentrations (U.S. EPA, 2018).
Table 4. Effects of FPL1 and DoC2 on mineral concentrations3 (experiment 2).
CONCLUSIONS AND APPLICATIONS
1. Preferential selection of pellets influences the perception of on-farm feed quality. Residual feed collected from feed pans for 5 consecutive days consisted largely of fines (73%) but nutrients did not vary across feed pan locations.
2. An appropriate on-farm feed sample collection procedure should be utilized to better understand the feed provided to birds in various locations in the house. We recommend emptying 5 select feed pans of residual feed along the feed line, calling for feed to fill empty pans and collecting fresh feed from these 5 selected feed pans for analysis.
3. When our recommended collection procedure was utilized, on-farm nutrient segregation was not apparent when augering high P: F feed through short feed lines. When this feed was augered short distances, nutrient homogeneity in feed pans was achieved.
4. Results from the current study build upon previous field research from our lab and validate that augering high-quality pelleted feed throughout short feed lines evenly distributes nutrients throughout the house. Although pellets and fines in the feed pan varied across feed pan location, the measured nutrient segregation markers were similar across feed pan location. These data support feed manufacturing techniques that improve pellet quality and showcase the importance of proper on-farm feed sampling methods.
DISCLOSURES
The authors declare no conflict of interest.
This article was originally published in 2024 Journal of Applied Poultry. Research. 33:100398 https://doi.org/10.1016/j.japr.2023.100398. This is an Open Access article under the CC BY-NC-ND license (http://creativecommons.org/licenses/by-nc-nd/4.0/).