INTRODUCTION
Campylobacter spp. is well recognized as the leading cause of bacterial foodborne diarrheal disease and poses a serious economical and health risk globally (Skanseng et al., 2010; Silva et al., 2011; Meunier et al., 2015). The most common route of campylobacteriosis infection in humans is the handling or consumption of contaminated poultry meat (Lee and Newell, 2006). Campylobacter spp. is a commensal organism found in cattle, sheep, swine, wildlife, and domestic pets. However, it is generally accepted that chicks are a natural host for Campylobacter spp. and can carry a very high number of them in their gastrointestinal tract, probably due to their higher body temperature (Lee and Newell, 2006; Meunier et al., 2015; Saint-Cyr et al., 2016).
Campylobacter spp. is thought to produce no clinical signs in poultry, under natural conditions (Saint-Cyr et al., 2016). However, experimental challenge of young chickens with Campylobacter spp. can induce strong inflammatory response and clinical disease, including diarrhea, weight loss, or even mortality. In addition, Campylobacter spp. is positively associated with higher levels of footpad lesions and hock burns, as a result of poor gut health and subsequently wet litter condition (Humphrey et al., 2014), and thus compromise health and welfare status of broiler chicks.
The implementation of Campylobacter spp. control measures at the primary production level is vital in order to control the campylobacteriosis in humans (Meunier et al., 2015). According to Romero-Barrios et al. (2013), the reduction in caecal colonization of flocks by 2 or 3 log10 could reduce human campylobacteriosis cases, by at least 76% or 90%, respectively. Furthermore, the prevention of the disease in humans and the reduction of the pathogen reservoir in poultry, without the need for antibiotics, are ecological and profitable for the environment and industry, respectively (Saint-Cyr et al., 2016).
A plethora of different approaches have been considered and developed to reduce the incidence of Campylobacter spp. at the poultry industry level including: the use of feed additives to reduce and even eliminate Campylobacter spp. from colonized chicks, the application of strict biosecurity measures, logistic slaughter, improved processing hygiene, and alternative approaches such as phage therapy and vaccination (Meunier et al., 2015; Guyard-Nicodeme et al., 2016; SaintCyr et al., 2016). However, there is still no effective, reliable, and practical intervention measure available to prevent Campylobacter colonization in broiler chicks.
On the other hand, the use of natural feed additives in the poultry industry for pathogen control and growth promotion are gaining attention, due to the restriction of antibiotics as growth promoting agents. Prebiotics, probiotics, synbiotics, herbs, enzymes, and organic acids, which stimulate the immune system, affect the physico-chemical properties of intestinal digesta and enhance the intestinal microbiota have been efficiently used (Chaveerach et al., 2004; Van Deun et al., 2008; Solis de los Santos et al., 2009; Skanseng et al., 2010; Silva et al., 2011; Hermans et al., 2012; Meunier et al., 2015; Guyard-Nicod`eme et al., 2016; Saint-Cyr et al., 2016).
Whey is considered a promising natural feed additive, as it affects the physico-chemical properties of intestinal digesta and enhances the intestinal microbiota (Corrier et al., 1990; Shariatmadari and Forbes, 2005; Tsiouris, 2014). It has been successfully used to control intestinal pathogens, such as Salmonella spp. and Clostridium perfringens in broiler chicks (DeLoach et al., 1990; McReynolds et al., 2007). However, since chicks’ digestive system is not able to degrade lactose, increased quantities of whey are often correlated with poor gut health and wet litter condition (Shariatmadari and Forbes, 2005). To the best of our knowledge, there are no reports in the literature concerning an antiCampylobacter effect of whey. Therefore, the objective of the present study was to investigate the effect of the whey on broiler chicks’ caecal Campylobacter counts, performance, and welfare.
MATERIAL AND METHODS
Experimental Study
Husbandry, euthanasia methods, experimental procedures, and biosecurity precautions were conducted in accordance with Greek legislation governing experimental animals and were approved by the Ethical Committee of the Faculty of Veterinary Medicine, School of Health Sciences, Aristotle University of Thessaloniki.
Birds and Housing
One hundred and twenty-one-day-old Ross 308 broiler chicks were obtained from a local commercial hatchery and were randomly allocated to four treatment groups of six birds with five replicates. Birds in each group were placed in a pen with deep litter of wood shavings, which were previously sterilized in an autoclave at 121 °C for 20 min (Cyclomotic control, EA605A). Each group was kept in a specially designed experimental room (Unit of Avian Medicine, Faculty of Veterinary Medicine, School of Health Sciences, Aristotle University of Thessaloniki), where the temperature, the relative humidity, and the lighting program were controlled, following the recommendations of the breeding company (Aviagen). Temperature and humidity were monitored in each room at two locations at bird level using a temperature–humidity record system (HOBO UX100-003 Temperature/Relative Humidity data logger, Onset Computer Corporation, 470 MacArthur Blvd., Bourne, MA 02532). The standard stocking density for all groups was 15 birds per m2 or 33 Kg per m2.
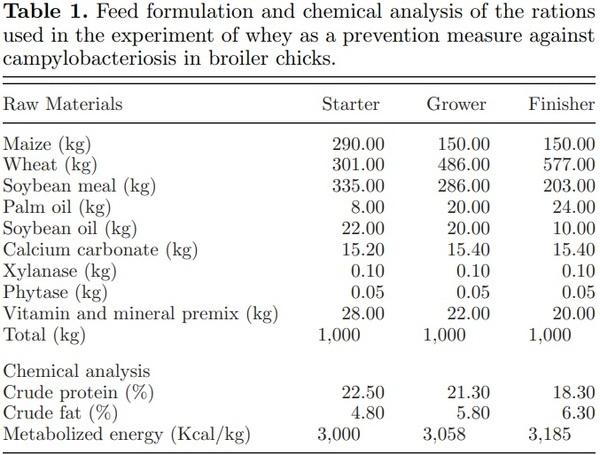
Experimental Diets
To meet the nutrient requirements of the broiler chicks during the experimental period, three complete basal diets were formulated each one for the starter (1 to 13 d), growing (13 to 23 d) and finishing period (23 to 37 d), respectively. The addition of whey powder was done on top in grower and finisher rations. Feed formulation and chemical analysis of rations is presented in Table 1, while chemical and microbiological analysis of commercial whey powder product is presented in Table 2. No antibiotic growth promoters, organic acids, and phytobiotics were used. Feed and drinking water were offered to all birds ad libitum throughout the experiment.
Campylobacter Strains and Inoculums Preparation
Campylobacter jejuni strain MB 4185 (KC 40) of poultry origin was kindly provided by Professor Frank Pasmans (University of Ghent, Belgium). Bacteria were cultured in Preston Campylobacter selective enrichment broth [Preston broth; Nutrient Broth No. 2 (CM00067, Oxoid) supplemented with sodium pyruvate (0.25 g/l), sodium metabisulphite (0.25 g/l), and ferrous sulphate (0.25 g/l) (Campylobacter growth supplement, SR0232, Oxoid)] at 41.5 °C for 24 h under microaerophilic conditions (5% O2, 10% CO2, 85% N2). Before inoculation the strain was cultured in Buffered Peptone Water (CM0509, Oxoid) at 41.5 °C and placed in a shaking incubator under microaerophilic conditions. An initial estimation of the Campylobacter count was performed by measuring optical density at 600 nm. In parallel, decimal dilutions in Maximum Recovery Diluent (CM0733, Oxoid) and surface platting in Columbia blood agar [Columbia blood agar base (CM0331, Oxoid) with 5% sheep blood (SR0051, Oxoid)] were performed. The initial inoculum was corrected to 107 colony-forming units (cfu)/ml by decimal dilutions in Buffered Peptone Water.
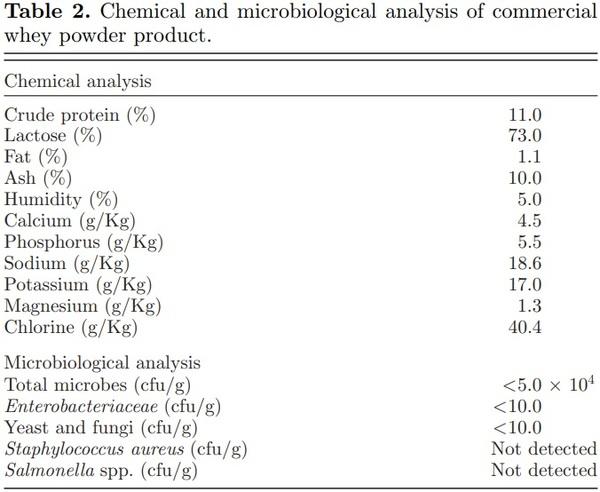
Experimental Design
The experimental treatments were the addition of 1% whey to broiler diets and the oral inoculation with 107 cfu C. jejuni at the 17th day of age. One hundred and twenty-day-old Ross 308 broiler chicks were randomly allocated to four treatment groups of six birds with five replicates, as described below: group A, which served as negative control; group B, supplemented with 1% of whey in the diets of birds; group C, challenged with C. jejuni; and group D, supplemented with whey and challenged, respectively. Three birds with five replicates per treatment group were sampled at 23rd and 37th day of age.
Performance
The body weight (BW) was measured on the 1st, 13th, 24th, and 37th day of age, while the feed conversion ratio (FCR), the average daily feed consumption (ADFC), and the average daily weight gain (ADWG) were calculated for the periods of the 1st to 13th day, 13th to 23rd day, 23rd to 37th day, and cumulatively. Mortality was recorded daily, while European Production Efficiency Factor (EPEF) was calculated at the end, taking into account the BW, the livability, the slaughter age, and the FCR. In order to assess the welfare status of broiler chicks, the performance indexes, the hock burn, and the foot pad dermatitis (FPD) lesions score were measured. In particular, all feet for each group were collected for macroscopic examination and scored in three classes of FPD, according to the classification of Ekstrand et al. (1997). In particular, score 0 for no lesions, score 1 for few and limited lesions, and score 2 for several or serious lesions. The group score was calculated from the formula below, leading to values between 0 and 60. The number of feet in class 0 did not contribute to the score. The number of feet in class 1 was multiplied by 0.5, the number in class 2 was multiplied by 2, and the scores were added. The same feet were evaluated for the incidence of hock burn on the basis of the absence (score 0) or presence (1) of the lesion.
Detection and Enumeration of Campylobacter in Caeca
The samples were examined for the presence and count of Campylobacter according to ISO 10272-1:2006 and ISO 10272-2:2006, respectively. Caeca were collected and homogenized in Preston broth and Maximum Recovery Diluent for the detection and enumeration, respectively. For detection purposes, the homogenates in Preston broth were incubated at 41.5 °C for 48 h. After incubation, 10 μl of the homogenate were surface-plated on modified charcoal cefoperazone deoxycholate agar [mCCDA; Campylobacter blood-free selective agar base (CM0739, Oxoid) supplemented with charcoal cefoperazone deoxycholate agar selective supplement (SR0155, Oxoid)] and incubated at 41.5 °C for 24 to 48 h under microaerophilic conditions. For enumeration, further dilutions were performed in Maximum Recovery Diluent, and 100 μl from each dilution was surface plated on mCCDA and incubated at 41.5 °C for 24 to 48 h under microaerophilic conditions. After incubation all characteristic colonies were counted. Up to five well-defined colonies were restreaked for purity on mCCDA and further typed by a multiplex polymerase chain reaction (PCR) method.
Multiplex PCR
DNA extraction was performed as described by Lazou et al. (2014). One loopful of cells was dispersed in 100 μl dispersal buffer (50 mM Tris-HCl, 50 mM ethylenediaminetetraacetate (EDTA), 1% v/v Triton X-100, pH 7.5), in which 100 μl of lysis buffer I (50 mM Tris-HCl, 50 mM EDTA, 4 M GuHCl, 10 mM CaCl2, 1% v/v Triton x-100, 2% N-Lauroyl-Sarcosine, pH 7.5), and 25 μl of proteinase K solution (22.4 mg/ml) were added, followed by incubation at 56 °C for 1 h. Consequently, 250 μl of lysis buffer II (50 mM TrisHCl, 25 mM EDTA, 8 M GuHCl, 3% v/v Triton x100, 3% N-Lauroyl-Sarcosine, pH 6.3) were added and incubated at 70 °C for 10 min. After incubation, 250 μl of absolute ethanol were added and the mixture was applied to silica columns (Qiagen). The column was then washed twice with wash I buffer (25 mM TrisHCl, 4 M GHCl, 50% ethanol, pH 6.6) and once with wash buffer II (10 mM Tris-HCl, 80% ethanol, 100 mM NaCl, pH 6.6). Finally, the DNA was eluted with ultrapure water and stored at –30 °C until examination. All reagents used were purchased from Sigma. The multiplex PCR method described by Wang et al. (2002) was followed with a modification so as to detect C. jejuni. Two primer sets were used (Table 3) for the detection of hipO and 23S rRNA genes of C. jejuni. PCR was performed at 25 μl volume containing dNTPs (200 μM), MgCl2 (2 mM), the primers CJF and CJR (1 μM), the primers 23SF and 23SR (0.2 μM), and Taq polymerase (1.25 U). All PCR reagents were purchased from New England Biolabs. The initial denaturation (6 min at 95 °C) was followed by 30 cycles of amplification (denaturation at 95 °C for 30 s, annealing at 59 °C for 30 s, and extension at 72 °C for 30 s), ending with a final extension at 72 °C for 7 min. The PCR products were visualized in 1.5% agarose gels stained with ethidium bromide.
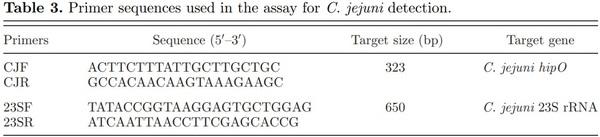
Field Study
The use of whey under field conditions was evaluated in a broiler farm with two houses (A and B), where the rations, the drinking, the feeding, the ventilation, and the heating systems were identical. The chicks were obtained from the same parent flock, hatched at the same hatchery, and transferred to the farm simultaneously. Seventeen thousands chicks were allocated to house A, which served as negative control and nineteen thousands chicks were allocated to house B, which fed 1% whey on top of the rations from 13th day of age until slaughter age.
Performance
The BW of 50 birds per house was measured on the 1st, 13th, 28th, 38th, and 43rd day of age, while the FCR and the ADFC were calculated on a pen basis for the periods of the 1st to 13th day, 13th to 23rd day, 23rd to 38th day and cumulatively. The mortality rate and EPEF was calculated at the end.
Statistical Analysis
Both parametric and nonparametric statistical methods were applied for the statistical evaluation of the experimental results. For accessing the assumptions of normality and stability of variances, data were transformed to log10 or sqrt. In the case of normality and variance’s homogeneity, a one-way analysis of variance was performed, to evaluate possible significant effects of treatment on the performance and the caecal C. jejuni counts. Differences between mean values of specific treatments were evaluated using Duncan’s new multiple range test. Where assumptions about either variability or the form of the populations distribution were seriously violated, with or without transformed data, the Kruskal–Wallis nonparametric test was applied to evaluate treatment dependent differences, while differences between mean values of specific treatments were evaluated using the nonparametric Wilcoxon rank sum test (Mann–Whitney U-test). All analyses were conducted using the statistical software program SPSS for Windows (v. 15.0). Significance was declared at P ≤ 0.05. Back-transformed mean values are reported in the results.
RESULTS
Experimental Study
The effect of whey in poultry diets on the performance of broiler chicks is presented in Tables 4 and 5. The BW was significantly higher in group C and significantly lower in groups D compared to group A and B in 13th day of age. Later on, the BW was not significantly different among experimental groups (P ≤ 0.05). The ADWG was significantly different in group D (P ≤ 0.05) in the starter period and significantly higher in group B in the finisher period compared to other groups. The FCR was significantly lower in group C (P ≤ 0.05) and significantly higher in group D (P ≤ 0.05) compared to other groups in the starter period, while in the finisher period as well as in totally, the FCR was significantly higher in group B (P ≤ 0.05) compared to other groups. The ADFC was significantly higher in group B (P ≤ 0.05) compared to other groups in the finisher period. The effect of whey in poultry diets on the EPEF is only indicative, since it was calculated on a pen basis, and had not been statistically analyzed and evaluated. The EPEF was higher in group C, while the lowest was in group B. As it is shown in Table 6, all unchallenged birds were remained C. jejuni negative throughout experimental procedure, whereas all challenged birds were C. jejuni positive at 24th and 37th day of age. In addition, C. jejuni counts were significantly higher in challenged groups compared to unchallenged groups (P ≤ 0.05) at 24th and 37th day of age. Between challenged groups there was no significant difference (P ≤ 0.05). There were neither footpad dermatitis lesions nor hock burn lesions in any of the experimental groups, as the hock burn and the FPD lesions score were 0 in all experimental groups.
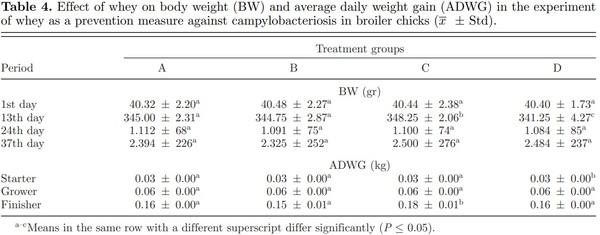
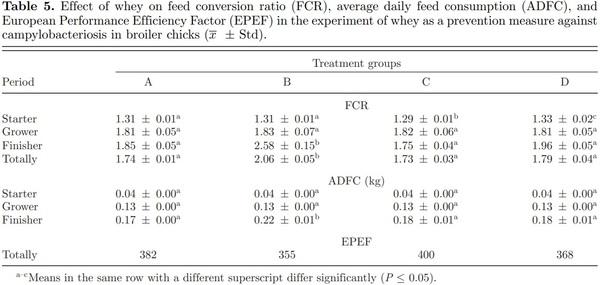
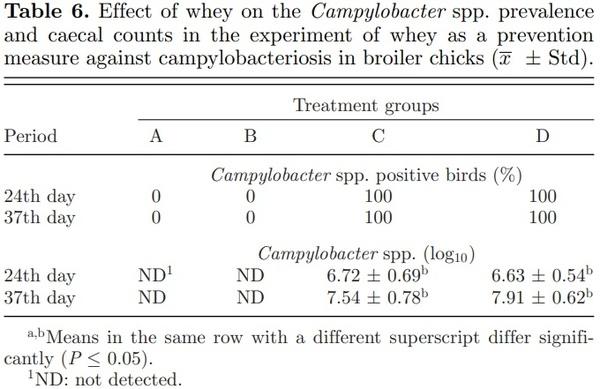
Field Study
In the field study, the FCR, ADFC, EPEF, BW, and mortality were presented in Tables 7 and 8. The data were calculated on a pen basis, with the exception of BW, and therefore they had not been statistically analyzed and evaluated. However, since the number of birds in each house was really high, we can draw some conclusions. More specifically, the use of whey had no effect on the FCR, ADFC, EPEF, and mortality, since they were almost equal between houses. The BW was significantly higher in group B compared to group A (P ≤ 0.05) at the 13th day of age, prior the use of whey in the poultry diets. The BW was relatively higher in group B compared to group A at the 43rd day of age. This difference was only 50 g per bird and it was not significant (P ≤ 0.05); however, if we consider it in a farm level or even in an integrated operation level, it could add significant profit to the farmer or to the operation.
DISCUSSION
Control measures at farm level are of vital importance in every Campylobacter control program. Measures taken to reduce Campylobacter spp. colonization in the intestinal tract of poultry during primary production have potential for reducing contamination of poultry products and thus the incidence of human campylobacteriosis (Meunier et al., 2015). In the present study, the supplement of 1% whey on the poultry diets had no effect on the performance, the welfare, and the caecal Campylobacter counts after experimental challenge. However, the addition of 1% whey on the poultry diets improved numerically the BW of broiler chicks under field conditions.
Whey is a by-product of the production of cheese, curd cheese, or casein from milk. It has been successfully used in the feed for young animals as milk replacer and fattening purposes of swine, cows, and calves (Shariatmadari and Forbes, 2005). The use of whey in the poultry diets may be compromised by its high water, lactose, and sodium contents. Chicken do not produce lactase as the mammals have evolved to do. This enzyme is responsible for the hydrolysis of the milk sugar lactose into glucose and galactose. (Mozdziak et al., 2003). In cases of large concentrations of whey in the diets, lactose remains unabsorbed and has been reported to cause diarrhea, with negative effects on broiler performance and problems with wet litter (Shariatmadari and Forbes, 2005; McReynolds et al., 2007). However, the results of the present study do not agree with the above, since the addition of whey had no negative effect on the performance under experimental conditions, whereas a positive effect has been observed under field conditions. This phenomenon could be attributed to the fact that in the present study a small dose of whey was used. Perhaps, whey has acted as a natural growth promoter, since it contains high nutritional value proteins, minerals, and vitamins (G¨ul¸sen et al., 2002). Moreover, lactose could have been used as a fermentation substrate by intestinal lactic acid bacteria and thus influenced the pH of the intestinal digesta, as observed also by Corrier et al. (1990).
Footpad dermatitis and hock burn are important problems of the modern poultry industry, and deteriorate bird’s welfare and health status, walking and feeding activity, performance indices, carcass quality, and economic profit of meat production. (Shepherd and Fairchild, 2010; Swiatkiewicz et al., 2017). Both conditions are contact dermatitis, which start as a hyperkeratosis, erosions and discoloration of the skin followed necrosis of the epidermis. In more severe cases, the changes lead to ulcerations and inflammation of the subcutaneous tissue. The lesions may serve as an entry point for pathogenic bacteria and complicated, leading to arthritis and systemic infection (Greene et al., 1985; Ekstrand et al., 1997; Shepherd and Fairchild, 2010).
Contact dermatitis lesions are caused by a combination of litter moisture and the chemical burning effect of ammonia from urea in the litter, when the litter contains high moisture with sticky fecal droppings (Martins et al., 2013). Both conditions are likely to cause pain, as a result of tissue trauma, the degree of which will vary with lesion severity. For this reason, the incidence and severity of contact dermatitis may reflect many aspects of bird welfare, as well as being a direct source of pain, and so may indeed be a valid assessment measure for broiler welfare (Haslam et al., 2007).
Increased quantities of whey can cause osmotic diarrhea and influence the water intake, the excreta moisture, and the litter quality and in this way affecting the occurrence and severity of footpad lesions and hock burn (McReynolds et al., 2007). In addition, C. jejuni-infected chicks showed signs of pododermatitis (Humphrey et al., 2014). However, in the present study footpad lesions and hock burn were show neither to Campylobacter-infected chicks nor to whey consumed birds. Plausible explanations, for the contradictory effect of Campylobacter challenge, could be the strain pathogenicity that used for the challenge of birds in the experiments (Saint-Cyr et al., 2016), as well as the breed sensitivity of the chicks, which used as experimental animals (Humphrey et al., 2014).
The effect of the whey on litter quality and subsequently to the footpad lesions and hock burn depends on whey concentration. High amount of the whey on the broiler diets causes osmotic diarrhea, increased liter humidity, and predispose to foot pad lesions (Shariatmadari and Forbes, 2005; McReynolds et al., 2007). On the contrary, low amount of whey, as it was to the present study, although it is not absorbed from the digestive system of the broiler, it is used as a substrate from the lactic acid bacteria of broiler microbiota, promoting gut health and performance (G¨ul¸sen et al., 2002; Shariatmadari and Forbes, 2005; Neal-McKinney et al., 2012).
The addition of whey in poultry diets has been used for the control of intestinal pathogens, such as Salmonella spp. and Clostridium perfringens in poultry (Corrier et al., 1990; DeLoach et al., 1990; McReynolds et al., 2007). In particular, the use of whey in poultry diets decreased the number of Salmonella spp. positive birds by 90%, and the intestinal carriage of Salmonella typhimurium by more than 2 log10 in chicks fed with whey compared to the controls (DeLoach et al., 1990). The proposed mechanism is that lactose acts as a growth substrate for beneficial bacteria of intestinal microbiota, such as Lactobacillus spp. and Bifidobacterium spp., which ferment it to lactic acid and volatile fatty acids. Possible mechanisms for the effect of lactic acid bacteria on intestinal pathogens include the production of bacteriocins, the stimulation of adaptive immunity, the alteration of the caecal microbiome, and the antimicrobial activity of the produced lactic acid (G¨ul¸sen et al., 2002; Neal-McKinney et al., 2012).
The ability of lactic acid bacteria, possibly favored by the addition of whey to chicken diet, has been extensively documented (Santini et al., 2010; Campana et al., 2012; Campana et al., 2017). Campana et al. (2017) report that lactic acid bacteria culture supernatant, adjusted to pH 6.5, showed the greatest zone of growth inhibition against C. jejuni ATCC 33,291, with invasion ability on Caco-2 cells variably inhibited. Moreover, Dolan et al. (1989) have reported that human whey inhibits among others the growth of C. jejuni. However, in our study, the use of whey in poultry diets did not affect the percentage of Campylobacter-positive birds neither the caecal Campylobacter counts. The results of our study are in accordance with the results of other in vivo studies, which also tried to investigate the effect of feed additives, such as organic acids, probiotics, and prebiotics on the C. jejuni counts (Hermans et al. 2012; Gracia et al., 2016; Guyard-Nicod`eme et al., 2016; Saint-Cyr et al., 2016). This could be attributed to the increased number of C. jejuni population and subsequently to the stability of this population in the caeca. A possible explanation is that the intestinal and caecal environment protects C. jejuni from the antibacterial activity of various substances (Gracia et al., 2016).
The use of whey did not suppress the growth and welfare in broiler chicks. Moreover, under filed conditions the use of whey increased numerically the BW of chicks. The anti-Campylobacter effect of whey was inexistent, since it had no effect on Campylobacter counts. Further research is required to find an optimum concentration of whey as well as a combination with different types of products, such as probiotics and organic acids, acting synergistically to reduce or eliminate the caecal colonization of Campylobacter spp. in broilers. In addition, further research on identifying the changes in physicochemical conditions and microbiota within the intestine in response to addition of whey in poultry diets would be worthwhile.
This article was originally published in 2019 Poultry Science 98:236–243. http://dx.doi.org/10.3382/ps/pey388. This is an Open Access article under a Creative Commons Attribution License.