1. Introduction
Maize is the most common energy source used in commercial monogastric diets, especially in the American, Southern Europe and most Asian countries where maize grain is the primary cereal for poultry feeds (Dei, 2017). However, the nutritional value of maize for livestock feeding can vary (Cowieson, 2005). Genotype, agronomic conditions and pre- and post-harvest processing can affect the chemical characteristics of cereal grains (Gehring et al., 2013). Indeed genetics has been demonstrated as an important source of biochemical and nutrient variability (Rodehutscord et al., 2016; Uribelarrea et al., 2004). The nutrient composition of maize can also be affected by the position of the grain on the cob and grain size (Nadeem et al., 2014). Consequently, the smaller, extreme apical grains can produce nutritional variability, which could affect the final composition of animal diets. Little is known about how grain position on the cob and their interaction with genetic factors contribute to variations in the chemical composition and physical characteristics of maize for poultry feeds. Characterization of the variation in nutritional value of maize grains that result from these factors may help define appropriate breeding objectives and improve the accurate use of additives that depend on nutrient composition, such as enzymes, in order to improve the feeding value of cereal grains for livestock nutrition. Xylanase is commonly added, particularly when viscous cereals such as wheat is used in poultry diets, in order to reduce intestinal viscosity of birds by degradation of soluble arabinoxylans (AX) (Choct et al., 2004). However, the benefits obtained by degradation of endosperm cell walls, which includes the release of encapsulated nutrients (“cage effect”) and/or through a prebiotic effect as a result of generation of short-chain oligosaccharides from polysaccharide AX (Bedford and Apajalahti, 2000; Khadem et al., 2016). However, these effects also contribute to the responses noted but these effects can be heterogeneous and result in variable animal responses, depending on the quantity and nature of the cereal fiber polysaccharides present.
It was hypothesized that maize genetic background and grain position on the cob might influence chemical composition, physical characteristics, and response to xylanase supplementation. The present study aimed to investigate the differences in nutrient composition, physical characteristics and short-chain xylo-oligosaccharide (XOS) content of basal or apical grains obtained from different maize hybrids harvested from the same field and under similar environmental growing and fertilizing conditions.
2. Materials and methods
2.1. Maize samples
Sixteen maize varieties were sown in the same field and environmental growing conditions (Gimenells, Catalunya, Spain), including fertilizer and harvesting. The rainfall of the area was 207 mm in the cultivation period and the average minimum/maximum temperatures varied between 15.2 and 29.3°C (AEMET, 2020). The planting density was 92,000 seeds/ha. Irrigation was with fixed cane sprinkling and, from sowing until an average plant height of 50 cm, at a rate of 1 h of watering per day, applied at night. From 50 cm until the plants had 12-14 leaves, they were watered according to the need for soil moisture, based on rainfall and visual assessment of the plants. From 12 to 14 leaves until the flowering spike is lost, water was applied for 30 min daily, while from flowering to harvest, plants were watered for 1 h daily at a rate of 50 min at night and 10 min at the time of maximum heat during the day. No pesticide was applied during cultivation, the fertilizer scheme was 170 kg of N/ha before sowing, 50 kg of liquid N/ha at 8 leaves, and 50 kg of liquid N/ha plus 5 L of organic fertilizer, through the irrigation system, at 12 leaves. Maize hybrids were sown in April 2018 and harvested in October 2018. The size of the land area and the harvest weights of each variety were used for the calculation of maize yield per hectare (Table 1). The plot for each variety consisted of eight rows with 17 cm of separation between plants within the row, and 70 cm of separation between rows of the same variety. The cobs used in the present study were collected at random from the experimental field; briefly, for each variety a total of 50 cobs were obtained from groups of five cobs in ten sampling points that followed a diagonal pattern along the four central rows from each variety plot. Ten cobs were then randomly selected from these 50 for each hybrid. For each cob, all grains were collected according to their position. Briefly, grain cob position was defined as apical or basal, with the line of differentiation between categories established subjectively considering the shape and size of the grains for each individual cob. The differences in the shape and size of the grains are shown in Fig. 1. Total grains from each position were oven dried at 60°C for 6 h, weighed (portion weight, PW), and stored in a cold room at 4°C. The proximate and physiochemical analyses were obtained for each hybrid and cob position using near infrared spectroscopy (NIRS), with results expressed on a fresh weight basis.
Eight maize samples (variety 1, 2, 7, 11, 12, 13, 14 and 16) were selected, based mainly on starch, protein and non-starch polysaccharide (NSP) content and were subsequently analyzed for solubility of dry matter (solDM), water retention capacity (WRC) and xylooligosaccharides (XOS, including X2: xylobiose; X3: xylotriose and X4: xylotetraose) release when incubated with xylanase in vitro.
2.2. Maize proximate and physio-chemical analysis
The fresh weight proximate and physiochemical characteristics of maize samples (16 varieties & 2 grain positions) viz: apparent metabolizable energy (AME), crude protein, starch, crude fat, crude fiber, neutral detergent fiber (NDF), acid detergent fiber (ADF), protein solubility index (PSI), vitreousness, phytic acid phosphorus, total non-starch polysaccharides (NSP), total arabinoxylans (AX), soluble AX, total arabinose:xylose ratio (A:X) and soluble A:X were predicted by NIRS (Foss DS2500, Hilleroed, Denmark) using calibrations provided by the Feed Quality Service of AB Vista (Marlborough, England, United Kingdom).
2.3. Dry matter solubility and water retention capacity
Dry matter solubility and WRC (Anguita et al., 2006) for five cobs from the eight selected varieties (variety 1, 2, 7, 11, 12, 13, 14 and 16), with two portions (apical and basal) and two levels of xylanase inclusion (with and without) were determined following an in vitro procedure which simulates gastric pH (n = 160). In short, the samples were milled and 0.5 g of each sample was weighed into a 10 mL screw cap tube and incubated with 5 mL of 0.1 M sodium phosphate buffer and 2 mL of 0.2 M hydrochloric acid (pH = 2.5). Additionally, 0.5 mL of a solution of liquid xylanase (Econase XT, 16,000 BXU/mL; one BXU is defined as the amount of enzyme that produces one nmol reducing sugars from birchwood xylan in 1 min at 50°C and pH 5.3.) was added to half of the samples at a dose of 16 BXU/mL. Tubes were kept at 41°C for 2 h in a horizontal shaking water bath. The amount of sample submitted to analysis was recorded (W0) as well as the weight of the screw cap tube plus the sample (W1). After incubation, the tubes were centrifuged for 20 min at 2000×g. The supernatant was carefully removed and tubes were kept upside down for 10 min to ensure that the non-retained water
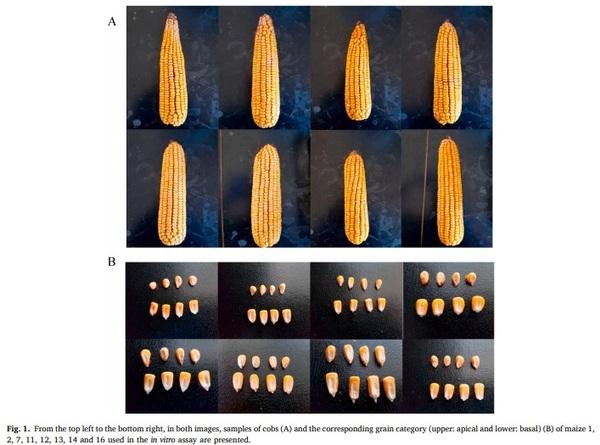
was drained. The supernatant was used for XOS determination. Tubes with sample were then weighed (W2), dried in the oven at 103°C for 16 h to ensure the complete drying of the insoluble residue, and then weighed again (W3). The solubility of the DM was calculated as follows:
SolDM = { W1 − W3 W0 } Water retention capacity determined after centrifugation is expressed as grams of water retained by the total amount of sample incubated: WRCDM = { W2 − W3 W0 }
2.4. Xylo-oligosaccharides determination
Determination of XOS was performed by high-performance liquid chromatography with an evaporative light scattering detector analysis (HPLC-ELSD), adapting the method described by Pu et al. (2017). Briefly, supernatant collected after centrifugation (20 min at 2000×g) in the SolDM and WRC analysis were used in these determinations. Xylose (X1; Merck Life Science S.L., Madrid, Spain), xylobiose (X2; Prod Code. O-XBI), xylotriose (X3; Prod Code. O-XTR) and xylotetraose (X4; Prod Code. O-XTE) were obtained from Megazyme (Wicklow, Ireland). Standards were used in a 250 ppm solution concentration with milli Q water to optimize the instrument parameters, and 0, 1, 2, 3, 4, and 10 mg/L solutions were prepared with milli Q water to obtain the calibration line. The samples and standards were filtered through 22 μm syringe filters. Analysis of the 162 standards and samples was carried out on an Agilent 1100 HPLC equipped with a DAD Agilent detector and an Agilent 1260 ELSD infinity detector. The HPLC column used was a Luna® 3u HILIC 200 A (150 × 2 mm), Phenomenex. The ELSD temperature was set at 80 C and air was used as nebulizer gas with a flow rate of 1 SLM. The injection volume was 1 μL. Column temperature was set at 35°C and flow rate was 1.0 mL/min. Despite high sensitivity, good precision, simple operation and rapid analysis by the HPLC-ELSD method, and being validated with purified samples of XOS in other studies (Li et al., 2016; Pu et al., 2017), the values obtained from this method are considered semi-quantitative, since the non-purified samples could contain compounds that could interfere with the results.
2.5. Statistical analysis
The cob was considered the experimental unit for all variables. The
physicochemical characteristics were analyzed by two-way ANOVA to identify genetic and grain cob position effects and the interaction between them. The solDM, WRC and XOS were analyzed by three-way ANOVA to identify genetic, grain cob position and xylanase effects and the interaction between them. The PROC GLM procedure was performed for both statistical analyses using SAS software (SAS, 2014). Significantly different means were separated using Tukey’s HSD test. Significance was declared at a probability P ≤ 0.05 and tendencies were considered when P-values were between >0.05 and < 0.10. Spearman’s non-parametric correlation analysis was used to explore the associations between NIRS nutrient predictions, solDM, WRC, and XOS semi-quantitative estimation, using corrplot package of R 3.6.1.
3. Results
3.1. Maize physicochemical analyses
The effects on physicochemical composition of the maize genotype and the grain cob position are showed in Figs. 2 and 3, as well as their interaction are shown in Tables 2 and 3. Predicted poultry AME, crude fat, crude fiber, NDF, ADF, total NSP, soluble NSP, total AX, soluble AX, phytic phosphorus, PSI, vitreousness, total A:X ratio, and portion weight were influenced by an interaction between genetics and grain cob position (P < 0.05). The poultry AME, phytic P, A:X ratio, PSI, and portion weight were higher in grains from the basal position compared to those from the apical position; however, these differences changed according to each variety. The contents of crude fiber, total NSP and AX were higher in the apical cob, while vitreousness and crude fat were higher in basal grains in most of the hybrids. In spite of the interaction, total NSP (range: 55.6–81.3 g/kg), soluble NSP (range: 1.0–8.5 g/kg), total AX (range: 38.8–50.0 g/kg), and soluble AX (range: 2.2–5.3 g/kg) showed the highest variability due to maize genotype.
Moisture, protein, starch, ash, and soluble A:X ratio differed (P < 0.005) by genetic and grain cob position as individual factors. Except for ash, these factors were higher in basal grains than in those from the apical portion (moisture: 104 vs 108 g/kg; crude protein: 78 vs 81 g/kg; starch: 672 vs 676 g/kg; ash: 10.2 vs 9.7, g/kg and soluble A:X: 0.86 vs 0.88 g/g). The average and standard deviation of these components considering the genetics were; moisture: 106 ± 11.6 g/kg; crude protein: 80 ± 5.6 g/kg; starch: 674 ± 13.3 g/kg; ash: 10 ± 1.0 g/kg and soluble A: X 0.87 ± 0.05.
3.2. In vitro analyses
Results from the SolDM, WRC and semi-quantitative determination of XOS in maize samples after the in vitro incubation with xylanase are shown in Table 4. An interaction between variety and grain cob position was observed for solDM, WRC, xylobiose, xylotriose and xylotetraose. The solDM and WRC were highest in the apical grains in six and five of the eight varieties, respectively. The contents of xylobiose and xylotetraose were highest in the apical grains in four and five of the eight varieties, respectively, while xylotriose content was higher in apical
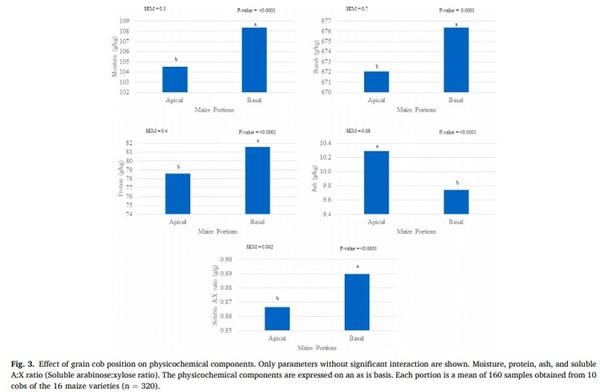
grains in seven of the eight hybrids (Fig. 4). Furthermore, two interactions were detected for xylotriose, the first between the genetic variety and xylanase, and the second between the grain portion and xylanase. The xylotriose content was increased in all hybrids when xylanase was added (Figs. 5 and 6). Similarly, higher xylotriose content was observed when xylanase was added in both grain cob positions; however, the response was greater in the grains of the apical portion compared those from the basal portion.
3.3. Correlations
Fig. 7 shows the correlation matrix and significance for the relationships between all parameters. Significant correlations (P < 0.05) were observed among physicochemical maize values. For example, predicted poultry AME was positively correlated with PSI (r = 0.83), fat (r = 0.71), soluble A:X ratio (r = 0.68), and negative correlated with crude fiber (r = − 0.50), total NSP (r = − 0.51), soluble NSP (r = − 0.70), and soluble AX (r = − 0.67). Starch was negatively correlated with crude fiber (r = − 0.72), total NSP (r = − 0.48), soluble NSP (r = − 0.47), total AX (r = − 0.40), and ash (r = − 0.63). Crude protein was positively correlated with crude fiber (r = 0.47), total A:X ratio (r = 0.49), phytic P (r = 0.51), and vitreousness (r = 0.82), and negatively correlated with starch (r = − 0.52). Soluble AX was negative correlated with PSI (r = − 0.74), crude fat (r = − 0.76), and AME (r = − 0.60). Vitreousness was positive correlated with soluble A:X ratio (r = 0.61) and PSI (r = 0.62). Maize yield was positive correlated with crude protein (r = 0.33), soluble A:X ratio (r = 0.37), PSI (r = 0.32), and vitreousness (r = 0.49). Additionally, significant correlations (P < 0.05) between physicochemical maize values and in vitro analysis were observed. The solDM in samples was negative correlated with phytic P (r = − 0.60). Xylobiose was negatively correlated with PSI and crude fat (r = − 0.42 & r = − 0.60, respectively). Xylotriose was positively correlated with WRC (r = 0.60). The xylotetraose was positively correlated with soluble NSP and soluble AX (r = 0.54 & 0.63, respectively), and negatively correlated with poultry AME (r = − 0.61), crude fat (r = − 0.56), soluble A:X ratio (r = − 0.40), and PSI (r = − 0.54).
4. Discussion
In the current study, the proximate analysis by NIRS of 16 maize varieties showed an effect of genotype on all parameters; however, most of these parameters also show an interaction with the grain position on the cob. The physicochemical characteristics which were not subject to a genotype:position interaction were moisture, crude protein, starch, ash, and soluble A:X ratio, but, as described, these were influenced by genotype. Rodehutscord et al. (2016) showed that genotype is an important factor influencing nutrient composition in several cereals used for animal feeding. The results obtained for moisture (97 ± 5.9 g/kg), crude protein (93.5 ± 9.18 g/kg DM), starch (740 ± 28.2 g/kg DM) and ash (13.3 ± 1.51 g/kg DM) of the 27 maize genotypes analyzed by Rodehutscord et al. (2016) are similar to those reported in the present study. Similarly, physicochemical composition mean values were also in
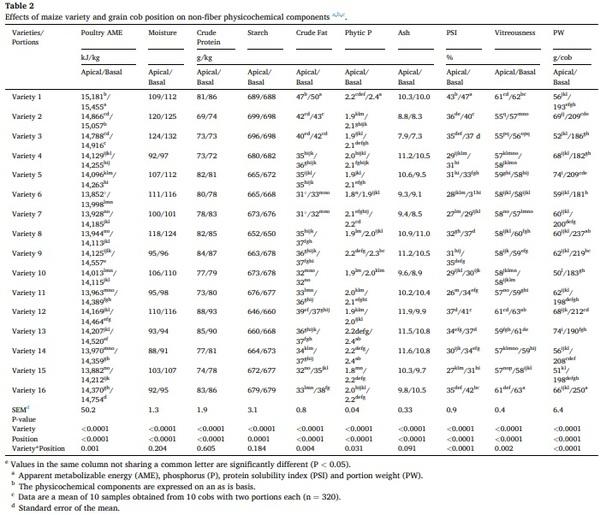
agreement with FEDNA (2019) values reported for Spanish maize; however, the standard deviation for many, including crude protein and starch (5 and 13 g/kg, respectively), could result in large differences between random samples and thus would affect the final nutritional value of diets based on such grains and consequently animal performance. The NIRS technology has become an important analytical tool in the field of animal nutrition, due to its practicality, ease of use, low cost, and speed in obtaining results. However, the accuracy is reliant on a detailed database of samples and the calibrations developed from this database.
Several studies have shown that the variability contributed by genotype for wheat, triticale, maize, and rye for nutrient digestibility was low with respect to ruminants (Krieg et al., 2017) but higher in poultry (Zuber and Rodehutscord, 2017). The lesser effect in ruminants could be explained by the fact that their digestive process is based largely on bacterial fermentation.
Vitreousness, the ratio of hard to soft endosperm, has been showed to be an indicator of nutrient digestibility in maize used in animal diets. In this regard, a negative effect on digestion of starch and NDF has been associated with high vitreous maize endosperm in dairy cows (Lopes et al., 2009). Similarly, in broiler chickens fed maize-based diets, the presence of a significant content of hard (high vitreousness) endosperm reduced the nutritional value (Kaczmarek et al., 2013). The present study identified variation in vitreousness with genotype and grain cob position, which suggests the proportion of hard endosperm varies with these factors.
The WRC was also influenced by genetic background, which is likely linked to differences in fiber components between varieties (Robertson and Eastwood, 1981). The high variation contributed by genotype for total and soluble NSP, and AX observed in the current study suggests that it could be important to consider these components in plant breeding and subsequently to take these into account in feed formulation. The antinutritive effect of soluble NSP is linked with increased digesta viscosity, with negative changes in digesta transit time and modification of intestinal mucosa and microbiota of the gut. Although the quantity of soluble AX is lower in maize compare than other cereals,
such as wheat, barley, rye, and triticale, the present study showed that genetics can play an important role in soluble AX variability in maize.
The increment of soluble dry matter content when xylanase was included in the incubation of maize samples in the in vitro simulation suggests a release of nutrients and/or soluble NSP and oligosaccharide production. Xylanase supplementation clearly can result in significant release of oligosaccharides when used with different cereals but maize seems to be much more intransigent to such effects compared with wheat of other small grain cereals (Dale, 2020; Morgan et al., 2017). However, there is no information concerning the variability in XOS production from maize samples when treated with xylanase; indeed Dale (2020) reported no production of XOS from maize samples when incubated with and without xylanase. However, the results of the present study have shown that XOS contents increased, albeit marginally, with xylanase addition in all maize samples, with different effects on xylobiose, xylotriose and xylotetraose release depending upon the maize sample itself. The current results show a marginal increment in xylotriose and/or reduction in xylotetraose contents with xylanase supplementation. This study demonstrates that there are clear differences in XOS contents between maize samples whether they are treated or not with xylanase. The amount of XOS likely is dependent upon the simulation conditions used, as it has been shown that xylanase production of XOS can be greater under more acidic conditions (Morgan et al., 2017). However, despite the benefits described about the HPLC-ELSD method, and the fact that the ELSD is not limited by the optical properties or functional groups of the analytes and has been widely used in the detection of chromophore-free compounds, such as carbohydrates (Ma et al., 2014), the results from this study should be confirmed by other methods, since maize extracts are complex and could contain compounds that could interfere with the analysis.
The grain cob position influenced moisture, crude protein, starch, ash and soluble A:X ratio. The apical and basal portions in this study represent 23 ± 2% and 77 ± 2% of the total grain cob weight, respectively. Apical grains contained less moisture, crude protein, starch and soluble A:X ratio, and higher total NSP, soluble NSP and other fiber compounds, suggesting the differences in nutrient availability of a maize sample can be due to grain position. In this regard, our results also showed a negative correlation between crude fiber, total NSP and total AX with AME, SolDM and PSI, suggesting that these fiber compounds influence nutrient availability. Previous studies showed that the size of the cereal grain could affect the physicochemical characteristics (Nadeem et al., 2014), possibly related to the amount of NSP, which has
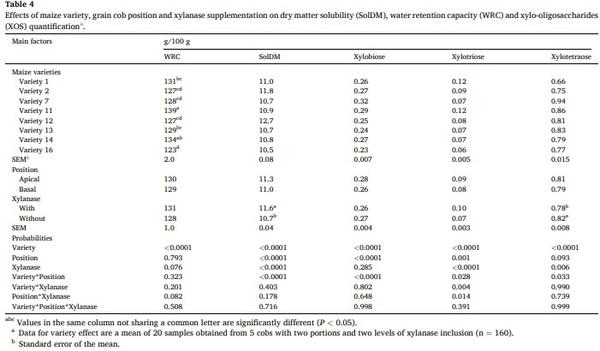
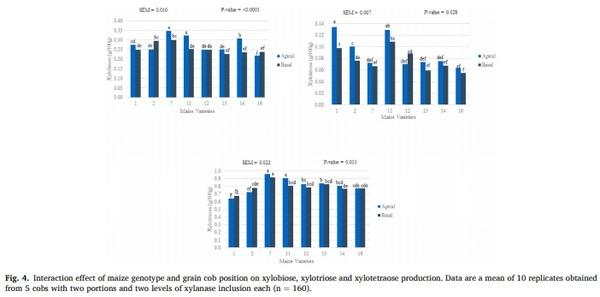
been shown to affect the nutritional value of the maize (Kaczmarek et al., 2013). Indeed, one of the strategies to minimize such variation in nutrient availability is the use of xylanase to reduce variability in animal performance resulting from dietary AX (Gonzalez-Ortiz et al., 2016). In the current work, xylanase application interacted with grain position, showing a greater production of xylotriose from the apical grains compared to those from the basal, which is likely related to the higher NSP values in the apical grains and/or lower soluble A:X ratio.
The interaction between genotype and grain position showed differences for many of the physicochemical characteristics of maize, including predicted poultry AME, crude fat, crude fiber, NDF, ADF, NSP, soluble NSP, AX, soluble AX, total A:X ratio, phytic acid phosphorus, PSI, vitreousness, and PW. As suggested previously, the interaction between AX and other major nutrients could have a negative effect on nutrient availability. Arabinoxylan physico-chemical characteristics, such as gelling capability, depends on many characteristics, including molecular weight, side-chain ferulic acid concentration and A:X ratio (Izydorczyk and Biliaderis, 1995), and AX-protein associations can also play an
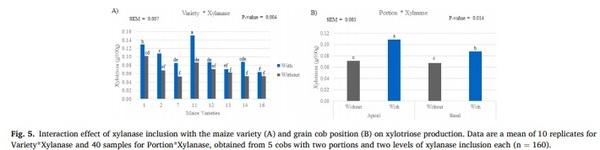
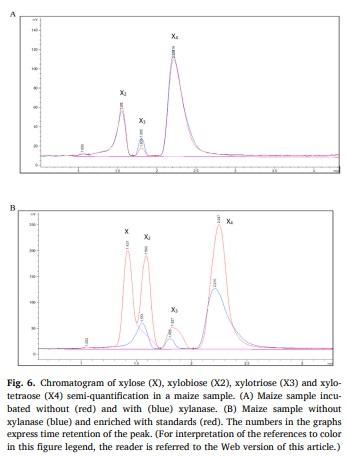
important role, for example on the digestibility of the protein fraction (M´endez-Encinas et al., 2019). These factors add to the problem of the deficient levels of lysine and tryptophan in maize (Larkins et al., 2017), so variance in their digestibility could exacerbate this and influence animal performance significantly. The amount and packing of protein within the grain is partly reflected in the vitreousness (Paulsen et al., 2003), and a potential reduction of access to digestive enzymes, with consequent lower digestibility. Non-starch polysaccharide anti-nutritional effects are considered minimal in maize, because it is believed to have a relatively low soluble AX content with a minor impact on animal digesta viscosity. However, in this study it was demonstrated that soluble AX ranged from 2.2 to 5.3 g/kg, depending on genetics. These values highlight that some maize could behave similar to wheats in promoting viscosity issues. An improvement has been observed in poultry performance and nutrient digestibility when carbohydrases are included in maize-basal diets, suggesting that the availability of the nutrients is compromised by these fiber compounds (Cordero et al., 2019). However, modulation of the microbiota by the supply of XOS produced from AX breakdown by xylanase represents an alternative method to increase the relative abundance of bacteria producing butyrate in the animal gut, as has been demonstrated by direct feeding of XOS (Cordero et al., 2019; Onrust et al., 2015). In this context, xylanase supplementation of maize-based diets could represent a valuable strategy to control the negative effects of AX. The improvement shown in the SolDM fractions when xylanase was added may indicate an increased nutrient release and/or oligosaccharide production.
The present results suggest that genetic background and the grain position on the cob, to a greater and lesser extent, contributed to differences in nutrient composition, while the xylanase supplementation could be used to improve the nutritional value of maize through the increment of soluble compounds (nutrient releasing) and microbiota modulation by XOS production. The eight maize samples selected for detailed analysis in the present study were assessed in a broiler in vivo trial that showed differences on performance and nutrient digestibility associated to the nutritional variability produced by the maize genotype, showing the impact of this variability under practical conditions.
5. Conclusion
In conclusion, maize genotype and grain position on the cob have an important influence on the physicochemical composition and oligosaccharide contents and may contribute to nutrient variability. The NSP compounds were close related maize predicted AME for poultry. Apical grains have lower nutritional value and higher fiber compounds, which could compromise the final composition of animal diets. Xylanase supplementation increased dry matter solubility and xylotriose content in vitro, suggesting an improvement in the nutrient availability if oligosaccharide production also occurred within the gastrointestinal tract of broilers.