One of the great challenges of the next decade will be to provide sufficient high quality proteins for the rapidly growing aquaculture industry. A wide range of species to be farmed will necessitate sourcing feed proteins that are able to meet the specific requirements of the target species. Fish meals have traditionally provided a large proportion of the protein required by aquaculture species, and even if there is no immediate shortage of supply, there are requirements for alternatives for a variety of reasons. One of the key aspects is the high cost of fish meals, mainly caused by an imbalance of supply and demand.
Although this may vary from year to year because of supply variation, the trend toward higher prices has been steadily increasing because of growing demand. There are bountiful supplies of many protein sources that may, in principle, be suitable for inclusion in aquafeeds. However, just because an ingredient has a high crude protein level does not mean that it is a viable alternative, and a much greater depth of knowledge must be attained before a product can be accepted. Of course over the last decade, many researchers have been considering the need for fish meal alternatives, and how they can fit into cost effective and environmentally balanced feeds (Bureau and Viana, 2003).
This paper discusses a more fundamental stepwise approach to the problem of supplying alternative protein sources using, where appropriate, applied enzyme technology. This stepwise approach will be described, followed by a case study following the same principles, using enzyme processed feather protein for aquaculture feed as an example.
APPLICATION PRINCIPLES FOR UPGRADING BYPRODUCTS
WHAT ARE THE PROBLEMS WITH BYPRODUCTS?Poor nutritional value. Many potential sources of protein have a low nutritional value. This may be manifest as a poor or low digestibility of the protein or amino acids, or because the amino acid profile is not suitable for the target species.
Antinutritional factors. Certain (mainly vegetable) proteins contain anti-nutritional factors; the best known of which is probably trypsin inhibitor, a digestive enzyme inhibitor present in raw processed soya beans. Many antinutritional factors can be neutralised by a process step, and in the case of trypsin inhibitor, it is inactivated by a heat processing stage.
Stigma/prejudice. Some feed ingredients have a long history of having of little or no value as an animal feed ingredient. In this situation, additional promotional work will be required if efforts to increase value in terms of enhanced nutritional value have succeeded, but stigma still exists.
Environment. Some products have an adverse environmental impact if they cannot otherwise be used, either in an unmodified or added value state. This may manifest itself (in the worse case) as disposal in a landfill, which results in both a loss of nutrients and a negative environmental impact by leaching pollutants into groundwater or by increasing biogas production.
Legislation. In some cases legislation may ban the use of certain products for use in animal feeds. In Europe, a temporary ban on certain processed animal proteins (OJEC, 2000; 2000/766/ec), has resulted in a temporary loss of protein resource for animal feeds. It is hoped that the temporary ban will be lifted in stages during 2004/5, resulting in some key animal proteins being available for farmed animals such as aquaculture species.
Supply/demand imbalance. If the supply/demand considerations do not initiate interest in upgrading, then development of an upgrading strategy is more difficult. While the current situation of uncertain and expensive supply failing to meet growing demand is not critical, these circumstances must be addressed when developing an upgrading protocol.
No specific target species. If there are no specific target species for the by-product, then making an economic model for the upgrading process is particularly difficult. Identification of key species as the target market for the upgrading protocol can be crucial to the success of an upgrading project.
FOCUS ON TARGET SUBSTRATEProtein and nitrogen compounds. These are the key parameters. Much of the nutritional value analysis will depend on protein, particularly if protein is a major nutrient in the overall nutrient profile of the product. Major emphasis is now placed upon the in vivo digestible amino acid profile of ingredients, so this is a vital parameter to consider.
Complex carbohydrates. Although protein is the major focus for aquaculture feeds, the presence of complex carbohydrate may limit how much positive effect can be achieved by targeting protein alone. Therefore, if complex carbohydrates are present, an enzyme step to minimise any potentially negative actions on protein digestibility might be introduced.
Fats, oils and waxes. Fats and oils may inhibit enzyme activity in some circumstances. If the natural level of fat is high or there is major contamination, then steps may be taken to reduce the potentially negative effect that the fat may have on the proposed protein upgrading step.
Minerals. Minerals present in high quantities may affect any proposed protein upgrading step by interaction with the substrate or enzyme. Thus the effect of certain minerals on both of these parameters should be understood and compensated for if possible.
Potential interactions. It is critical to know in detail the nutrient profile of the target product. To be able to move the upgrading project forward, an understanding of the sample analysis and its provenance is essential. These details will be required for both targeting the parameters to be upgraded and for defining value to the target species.
THE KEY PROCESS PARAMETERSThe parameters discussed below are all factors that require a stepwise approach to the optimisation of the process. Here the benefit of using laboratory and pilot scale development equipment cannot be overestimated. The iterative approach required is possibly achieved most successfully in applied research establishments such as the Alltech Bioscience Centres.
First the substrate must be defined. For example, the protein content and configuration of a raw material should be known before any attempt to upgrade is made. Any potential interaction with another nutrient such as complex carbohydrate that might affect protein or amino acid availability should also be considered.
In general, particle size should be as small as practical to ensure that the largest surface area possible is available for enzymic hydrolysis. If an additional pre-milling or size reduction step is required, then this requirement needs to be confirmed and included in the process development steps. As a rule, sufficient active water content is needed to ensure optimal hydrolysis. The optimum range may be narrow or wide, and this optimisation also requires previous knowledge or new development research, possibly using a pilot plant. When considering moisture, processes that operate for all or some of the time in a high moisture environment may have a significant advantage over those that are dehydrated before any enzymic action might be possible.
It is well known that most enzymes are somewhat sensitive to pH, and this range must be considered when matching the enzyme and substrate, particularly if any pH adjustment is likely to be required. As with pH, temperature and its control are critical. Too low a temperature may mean processing time is too long to be commercially viable. If temperature is too high, there are potential problems with enzyme inactivation when critical limits are reached. Of course many commercial enzymes have a broad range of temperature optima, so the challenge is to match the optimum enzyme temperature with the process temperature without having to make excessive alterations to the latter.
Mixing is a vital feature that may be overlooked, particularly when additional equipment is needed to facilitate optimal enzyme hydrolysis. If mixing is inadequate, then the desired improvements may not be realised, and the project may fail. Therefore the importance and scope of any mixing needs to be considered as early in the project as possible, as additional capital may be needed if a mixing stage is needed.
The enzyme of choice will be determined by the substrate, but many of the above mentioned factors may need to be taken into account before settling on one or more enzymes to be used. In some circumstances enzymes alone may not be completely effective and great benefit could be obtained by introducing a co-factor. For products with a complex chemical composition, the addition of a co-factor designed to achieve a specific purpose may allow the enzyme to be greatly more effective. Stabilization is critical. It is assumed that the materials of interest are of a high or unstable moisture content after the enzyme activity is complete. The choice of stabilization method depends on a number of factors, including the possible environmental challenges of one route vs another, the cost of equipment, operating (fuel and drying) cost and finished product transport cost.
The application of heat to dry the product will both inactivate any residual enzyme activity and reduce water activity to a level where the product is stable. Wet preservation is generally achieved by the addition of acid or alkali with the purpose of inactivating the enzyme and inhibiting any possible spoilage organisms. The moisture content is unchanged from the starting level, and therefore transport of finished product includes cost of transporting water as well as the product.
COMPARING CONVENTIONAL AND ENZYME PROCESSESIn the context of processes that are suited for the application of enzyme technology, there are existing processing conditions usually described as ‘conventional’. Here, benchmarks for a variety of process and product parameters are already known, and these can be used to evaluate the differences and improvements that enzyme technology can bring. In most cases a monetary value change can be ascribed to the differences seen, although this is not always possible with concepts such as product prejudice.
There are a number of key benchmarks that can be considered when comparing conventional and enzyme processing. Energy requirements are one such consideration. Many processes using enzyme technology will require less energy input in a variety of forms e.g. mechanical or electrical power, and steam generated to provide heat for processing and dehydration. Likewise, environmental impact of conventional and enzyme processes differs. Emissions to the environment in the form of solids, gases, or liquids must all be controlled and in the future minimised. The process and product that can deliver environmental benefits over a conventional method will be more valuable in the long term.
A product nutrient comparison of conventional and enzyme-processed products may not reveal major or dramatic differences using the proximate analysis. In reality, much of the value added by enzyme processing must be considered using other evaluation techniques such as in vivo response or animal performance. In vivo response is the penultimate evaluation method, but may involve both costly and time-consuming techniques. Notwithstanding these potential negatives, a number of in vivo tests are nearly always required as a prelude to a smaller number of animal performance trials. An in vivo evaluation may not however be possible in the prime target species, and a surrogate may be necessary as a first step, particularly if more than one target species has been identified. Palatability, mortality, feed conversion and growth must be evaluated. This type of evaluation will allow an economic value to be ascribed to the product in question, and therefore a cost benefit analysis of the upgrading technology step can be completed.
NEXT: FITTING INTO FEED FORMULASThe animal performance evaluation will yield data that will allow nutritionists and feed formulators to fully include the upgraded product into commercial formulas. The choice at that point is between an inclusion strategy that targets cost savings or one that focuses on performance enhancement, or a mixture of both. Many nutritionists will set up a formula to compare the conventional and enzyme products such that there will be a direct comparison. Alternatively, both products could be used as substitutes for a higher value ingredient, such as low temperature (LT) fish meal, and the costs of diets compared. This approach has great value if the formulator is able to make equally nutritious diets that give equal performance. Here the full economic value change can be determined under realistic commercial conditions.
Sustainability is particularly important if the conventionally-manufactured product is prohibited (in extreme cases ) or restricted to a lower level that does not allow use of all of the product. Notwithstanding economics, if enzyme upgrading allows full utilisation of a product, this can be seen to improve sustainability of the whole livestock sector. The proof of any economic advantage can only come after performance trials have been completed and the improvements indicated by previous evaluations have been proven. Under these circumstances, the enzyme technology can be endorsed as providing the expected benefits. In the case of advantages both at the process and the inclusion (in feed) level, then the economic advantage may be shared, according to the local circumstances.
Case study: Enzyme hydrolysed feather protein for aquaculture feeds
PROBLEM TO BE SOLVEDHydrolysed feather protein has in the past been poorly regarded by nutritionists around the world. Its status as a variable product with low digestibility and an absence of essential amino acids has probably justified this opinion in the past. However on the positive side, the high level of crude protein, and high levels of some key amino acids might make it a valuable ingredient for certain target species. Feather protein is comprised of keratin, a complex polymer that is difficult to solubulise. As a result, typical processing using steam to hydrolyse the feather often results in an overprocessing which destroys part of the chemical constituents.
In consideration of the nature of feather protein it was decided to offer maximum upgrading potential. A stepwise approach was adopted, commencing with laboratory studies and continuing through pilot plant tests and into full-scale production. The trial goal of targeting aquaculture feeds as the most appropriate use of the upgraded protein was kept in mind from the early stages of the project.
TARGET SUBSTRATEProtein is the major component of feathers comprising ~85% of the dry matter, but oil/waxes are also naturally present at ~5%. These fatty components may interfere with protein hydrolysis, so their presence must be dealt with by use of appropriate enzymes or surfactants. In the case of keratin protein, there are numerous sulphur bridges. A reducing compound may be effective in assisting the enzyme to break down these structural bonds.
PROCESS PARAMETERSIn the case of feather proteins there are a range of conventional or steam hydrolysis systems in use around the world. In most cases the most effective way forward has been to adapt conventional systems by addition of an extra step or by simply altering the processing profile.
LABORATORY AND PILOT SCALE PRELIMINARIESDuring the development of the enzyme system key process parameters must be controlled. The following parameters were evaluated in laboratories such as the Alltech Bioscience Centers followed by pilot plant work, initially at PDM Group, Ltd. in the UK. Feathers are naturally present in a wide variety of shapes and sizes, ranging from small down-type feathers from broilers to large primary turkey wing feathers up to 18 inches long. The development work has concluded that no pre-size reduction is necessary, as long as all other parameters are met satisfactorily. Process temperature and time are vital parameters. Temperature must be controlled below a maximum of 55ºC. A target of 50ºC is recommended, for a time of 30 minutes. If a temperature of 50ºC is not possible, then 40ºC for 60 minutes or 30ºC for 120 minutes are viable alternatives.
A dose of 0.5 kg of Allzyme
™ FD (Alltech Inc.) plus 2.0 kg of Allzyme
™ CoPack per tonne of raw feather is recommended. This addition rate was established following a series of dose response experiments.
The method of mixing should be able to distribute the enzyme and Allzyme
™ CoPack throughout the feathers. A ribbon blender type design is particularly suitable, either as integral to the batch processor (as used in this study) or as a separate unit in a continuous system.
An adequate level of moisture is required to provide the appropriate water activity. In the case of feathers the natural moisture content is about 70% after removal from the bird and transporting around the slaughterhouse using a water fluming system. Some operators press excess water out of the feathers, resulting in a moisture level of ~50%. However this is still an adequate level of moisture for enzyme action to take place.
Sterilization is important for hygiene standards to be attained, and a pressure / temperature combination of 2 bar/122°C for 20 minutes is sufficient to kill pathogenic microorganisms, such as Salmonella spp., and also vegetative states of bacteria such as Clostridia spp.
The moisture level following sterilisation is similar to the starting level, so dehydration is required to stabilise the product to ~5% moisture. There are several choices, including indirect steam drying or direct air drying. There is anecdotal evidence that the latter is preferable in terms of enhanced nutritional value, but the former may have advantages in terms of lower energy demands and the fact that environmental controls of lower volumes of air are somewhat easier.
For the enzyme process to be introduced into a full scale batch production, only changes to the processing profile will, in all probability, be necessary. As a result, no additional investment in plant and machinery is required. For continuous systems an additional enzyme process step is required which takes the form of an enzyme pre-hydrolyser unit. Both systems are outlined in Figure 1.
EVALUATING RESPONSESIn the case of enzyme processing of feathers there are two main desired effects. Firstly there are process and environment benefits, and secondly there are nutritional improvements.
Process and environmental responses include significantly lower energy inputs required, as can be seen by the contrast in time and temperature profiles in Figure 2. The lower input of energy in the enzyme process also results in lower environmental pollution because there is less breakdown of the nitrogen and sulphur-containing protein. As a consequence, lower levels of polluting chemicals such as ammonia and hydrogen sulphide need treating by the environmental control equipment, resulting in lower environmental control costs.
Nutritional evaluation requires assumptions to be made which may or may not always be valid. A major issue arises for feather protein when it is assumed that in vitro and in vivo measurements of nutritional value are positively related. This is not the case for feather protein digestibility and therefore only in vivo measurements are valid (Woodgate, 2004a). In addition, much preliminary work was necessary in a species that was not expected ultimately to be the major target species. For example, in the case of feather proteins, much in vivo evaluation was completed in chickens, although the substrate is not expected to feature in poultry diets in the future. The typical analytical profiles of hydrolysed feather proteins compared to fish meal, including in vivo data, are shown in Table 1.
In the case of hydrolysed feather protein, legislative limitations must be considered. In particular, the European Union (EU) regulation on the use of animal by-products (OJEC, 2002) is a limitation to utilization both in Europe and in countries that export to Europe. Woodgate (2004b) describes the practical implications of this regulation.
Figure 1. Flow diagram showing production of enzymehydrolysed feather protein.
Figure 2. Comparison of standard and enzyme-hydrolysed feather protein processes.Table 1. Typical analysis of enzyme- and steam-hydrolysed feather proteins and fish meal1.
1In vivo determination in poultry of feather protein sources (not fish).
2Allzyme™ FD.
*Derived from in vivo data.Response to feather protein meal in aquaculture Hydrolysed feather protein (HFP) in aquaculture feed meets both the requirements of the EU regulation and potentially offers a supply of highly digestible amino acids to a farmed species which has a particularly high demand for them. Preliminary digestibility studies were completed in rainbow trout (Serwata et al., 2004) and a comparison of enzymehydrolysed HFP with steam-hydrolysed HFP, together with a reference protein (low temperature fish meal, LTFM). Table 2 indicates the in vivo digestibility of protein and Table 3 shows the in vivo digestibility values for essential amino acids for all three protein sources.
Table 2. Digestibility data for enzyme- and steam-hydrolysed feather protein (HFP) sources compared to low temperature fish meal in rainbow trout.
Table 3. Amino acid digestibility (%) for enzyme- and steamhydrolysed feather protein (HFP) sources compared to low temperature fish meal in rainbow trout.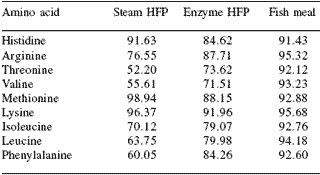
The digestibility data obtained in this trial were then used to formulate a series of diets for rainbow trout that were isonutritive in terms of crude protein, digestible amino acids and energy. The growth trial was completed over 10 weeks, with the increase in live weight over the period shown in Figure 3. There were no significant differences in growth between the treatments, thus confirming the in vivo nutritional value determinations, and most importantly indicating that successful formulation based upon digestible amino acids is both practical and feasible. This allows more precision in formulation and lowers the waste protein in the diet that could be a potential source of pollution. Table 4 shows a comparison of the supply of indigestible protein (from digestibility data) which, when supplying equal amounts of digestible protein, reveals a significant difference between the steamhydrolysed and enzyme-hydrolysed feather protein meal. These differences are important, as minimising the potential for nitrogen pollution is now critical for the aquaculture industry.
Figure 3. Effect of feather meal processing method on liveweight gain of rainbow trout.Table 4. Feed trial formulations including substitution of fish meal protein with feather protein and the indigestible protein content of the diet (kg/mT).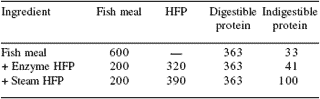
Feeding trials on two tilapia species have been completed at the University of Plymouth in the UK (Davies and Fasakin, 2004), and preliminary data are shown in Tables 5 and 6. In both of these species, a positive response to the inclusion of enzymehydrolysed feather protein was observed when compared with the conventional steam-hydrolysed feather protein. Increased weight gain and improved FCR was seen with the diet including the enzymeprepared product; and this improvement is currently being converted into economic value.
Table 5. Summary of feeding Trial 1: Tilapia (O. niloticus).
Table 6. Summary of feeding Trial 2: Red tilapia (O. niloticus x O. mossambicus).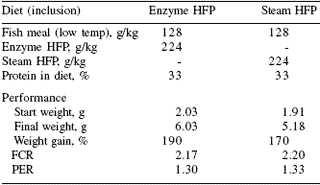
Further research is ongoing in a range of other freshwater and marine species, including sea bass, sea bream and turbot. Plans are to extend studies to shrimp and eel in the future.
Conclusions