1. Introduction
Salmonella enterica subsp. I serovar Enteritidis (SE) is the world’s leading cause of human salmonellosis (Braden, 2006; Schroeder et al., 2006). It is unique among over 2500 S. enterica (S.) serotypes, because it alone has the ability to efficiently contaminate the internal contents of eggs produced by otherwise healthy hens at a frequency associated with substantial food-borne disease (Gantois et al., 2009; Guard-Petter, 2001). The phenomenon of egg contamination is a pinnacle biological behavior impacting epidemiological prevalence, because S. Enteritidis has remained a prevalent and persistent problem within the food supply ever since its emergence (Gould et al., 2013). Some of the biology that differentiates SE from other pathogenic Salmonella serotypes associated with foods includes the ability to use the O-antigen as capsular material to aid survival in the internal contents of eggs, the ability to grow to high cell density, and the presence of a SEF14 fimbria (Guard-Petter, 1998; Morales, Guard, Sanchez-Ingunza, Shah, & Harrison, 2012; Parker, Liebana, Henzler, & Guard-Petter, 2001). Evolutionary adaptation of SE has been described as originating from recombination between strains centuries ago, and was then followed by continual generation of single nucleotide polymorphisms (SNPs) and niche adaptation to modern day environments associated with the egg-laying hen (Guard, Shah, Morales, & Call, 2011).
We suggest that progressive approaches are required to break through a plateau in reduction of SE and other Salmonella serotypes as food-borne pathogens (Fig. 1). We suggest that a lack of fundamental knowledge of the biology of SE in context of S. enterica in general impedes further reductions despite increased surveillance (Shuren, 2009). One factor known to have impeded research into the ecology of S. enterica is the relative lack of efficient methods to conduct serotyping, which is defined by epidemiologists as one of the most thorough methods of linking genotype to phenotype for pathogens known to exist. Technological advances have been made in the ability to serotype S. enterica subspecies I, and the cost and complexity of assigning serotype and conducting ecological surveys has been reduced (Guard et al., 2012; Pulido-Landinez, Sanchez-Ingunza, Guard, & do Nascimento, 2013). Therefore, we suggest that increasing knowledge of how serotypes interact in the hen to impact organ invasion is a relatively new area of research that can be explored to determine characteristics of healthy farms.
Earlier research had shown that SNP patterns from a reference strain of SE was at the opposite end of a scale that placed S. enterica serovar Kentucky (KY) closer to Escherichia coli in regards to gene content. We suggest that the juxtaposition of SE and KY SNP content warrants investigation into niche specialization for each serotype. KY is extremely prevalent in the poultry environment (Joerger, Sartori, & Kniel, 2009). However, it is infrequently observed as a laboratory confirmed serotype in people. For example, SE was the most frequently encountered laboratory confirmed serotype from people, and it was found in 15–20% of total laboratory samples collected between the years 2005 and 2011 (Fig. 1). During the same period, KY was confirmed in only 0.2–0.3% of human-derived samples and it was not in the top 40 serotypes encountered in people (Gould et al., 2013). KY was the most prevalent serotype recovered from non-clinical samples from chickens (17.3%) and the second most frequent isolate from clinical samples from chickens (18.5%) in 2011 FSIS, 1998 [calculations were made from serotyped isolates only]. In comparison, SE was the most prevalent serotype from clinical samples from chickens (55.6%) and the second most prevalent from non-clinical samples (14.2%). These data confirm that both SE and KY are prevalent within poultry and their environment, but only SE is a major threat to the safety of food. Despite an infrequent association with foodborne illness, KY can be a concern because of multiple antibiotic resistances (Le Hello et al., 2011).
Given that two poultry-associated serotypes differ greatly in their impact on food-borne illness in people, we wanted to investigate if there was any evidence that prior exposure of mature hens to KY could impact the recovery of SE given to hens at high and low dosages. In this model, results from contact exposure are believed to more closely resemble field conditions, whereas higher systemic doses of SE allow further evaluation of organ invasion by SE in hens exposed to KY. Experiments described are initial forays into a complex topic, namely characterizing how serotype complexity of Salmonella on-farm might be used to help reduce food-borne illness in people. The main objectives were to evaluate impact of dose and route of exposure and best sampling times. While these are modest goals, details on how best to conduct such experiments require exploration before information can be applied to field situations.
2. Materials and methods
2.1. Bacterial strains and inoculum preparation
S. enterica subspecies I serovars Enteritidis (SE) and Kentucky (KY) with in-house accession numbers 22079 and 100304.7, respectively, were used throughout experimentation. The SE strain is a phage type 4 isolate obtained from an outbreak in California and subsequently tested for being virulent in egg laying hens (Kinde et al., 1996; Morales et al., 2012). The KY strain was isolated from a carcass rinse of a chicken (Guard et al., 2012). Both SE and KY strains were confirmed through DNA microarray hybridization and intergenic sequence ribotyping as strains likely to be encountered in the United States (Guard et al., 2012). Strains were reconstituted from culture frozen in brain heart infusion (BHI) (Acumedia, Lansing, MI, USA) broth supplemented with 20% glycerol and stored at 80 C. Frozen stocks were streaked onto brilliant green (BG) agar (Acumedia) for colony isolation. Plates were incubated for 16 h at 37 C. Single colonies of each strain were transferred to 10 ml brain heart infusion broth (BHI) and incubated for 24 h at 37 C. Cultures for intramuscular (IM) injection were centrifuged at 10,000g for 10 min, washed once in 1X PBS, and then suspended in 1X PBS to an optical density of 1.0 at 600 nm (AD600). Cultures of KY for per os (PO) exposure of hens were diluted to an optical density of 1.0 in BHI without centrifugation or washing of cells. To determine dose, an aliquot from each inoculum was serially diluted 5-fold for 5 dilutions. Then 20 ll per well was plated onto BG agar to determine plate counts and standard deviations. 2.
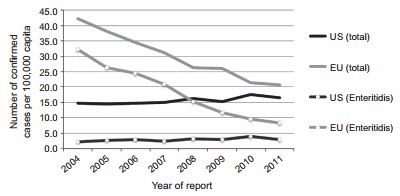
Fig. 1. Reported incidence per 100,000 capita of Salmonella enterica. Solid lines show total reported Salmonella enterica and the marked lines indicate reports for serovar Enteritidis (SE) only. For the US, data were obtained from the Centers for Disease Control FoodNet sites for the year indicated available at www.cdc.gov/FoodNet/reports.htm. For the EU, data were obtained from The European Food Safety Authority for the year indicated at www.efsa.europa.eu/en/efsajournal/doc/.
2.2. Experimental infection of laying hens
Animal experiments were reviewed and approved by the Southeast Poultry Research Laboratory Institutional Animal Care and Use Committee (IACUC). Leghorn hens, 27 weeks of age, were obtained from a specific-pathogen-free flock of single-comb white leghorn chickens (negative for antibodies to Salmonella in periodic routine monitoring) at the Southeast Poultry Research Laboratory in Athens, GA. Hens were distributed among four groups housed in a BS II disease-containment facility. The four treatment groups were:
(I) [SE-CN] – hens exposed by contact to SE only by housing underneath cages of hens injected intramuscularly (IM),
(II) [KY-CN:SE-CN] – hens exposed to both KY and SE by contact by housing underneath hens given KY by mouth (PO) and then SE IM 2 weeks later,
(III) [SE-IM] – hens injected with SE IM only,
(IV) [KY-PO:SE-IM] – hens given KY PO, and then injected 2 weeks later with SE IM
Birds were housed in individual laying cages and provided with water and pelleted feed ad libitum. Hens inoculated orally received 0.5 ml oral dose of the KY culture preparation. Intramuscular injections of SE were done in a 0.5 ml volume.
2.3. Culture conditions for recovery of Salmonella
2.3.1. Fecal samples and paired cecal tonsils
At times before exposure to KY, before exposure to SE, and at 6, 13 and 20 days after exposure to SE approximately 1 g voided feces were collected at 6 locations under each tier of cages housing 20– 24 hens divided into upper and lower cages. Samples were transferred to 9 ml of tryptic soy broth (TSB) (Remel, Lenexa, KS, USA) and incubated for 48 h at 37 C. A 1 ml portion from each culture was transferred to Rappaport–Vassiliadis (RV) broth (Acumedia) for 24 h at 37 C, and then streaked onto brilliant green (BG) agar (Becton, Dickinson, and Co., Franklin Lakes, NJ, USA) and incubated for 24 h at 37 C. The serotype of colonies of Salmonella was confirmed by intergenic sequence ribotyping (ISR) as described. Paired cecal tonsils, spanning from the base of the cecal pouch to the ileocecal junction, was another complex flora sample that was cultured. Samples were put into tubes containing seven 3.2 mm sterile beads and 9 ml TSB, processed for 2 min at a setting of 8 in a DX-50 Bullet Blender (Next Advance, Averill Park, NY, USA), and then further processed as were fecal samples for serotype by ISR. 2
2.3.2. Internal organ samples
At 6, 13 and 20 days post-inoculation, approximately 1/3 of hens in each treatment group were humanely euthanized to allow removal of internal tissues for bacteriologic culture. Portions (approximately 5–10 g) of the liver, upper oviduct (centered on the infundibulum/magnum junction), middle, and lower oviduct (centered on the isthmus/uterus junction) from each hen were aseptically removed, transferred to tubes and processed by bead beating in 9 ml TSB for 2 min at a setting of 8. Spleens were placed in sterile whirlpak bags with broth, capsule was broken through the bag by pinching, and then they were stomached for 2 min. Each broth culture was incubated for 48 h at 37 C, and a loopful was then streaked onto BG agar. Typical Salmonella colonies were then serotyped by ISR. Recovery of SE from the oviduct can vary substantially, and thus 3 regions were sampled to improve recovery of SE (Guard, Gast, & Guraya, 2010).
2.4. Statistical analysis
Significant differences between treatment groups were defined using the Student’s T-Test as possibly significant if P value (P) was less than <0.05 but >0.01 between treatment groups, and significant if equal to or less than <0.01. Discussion of trends does not indicate an assessment of significance.
2.5. Determination of serotype by dkgB-linked intergenic sequence ribotyping (ISR)
Intergenic sequence ribotyping (ISR) analyzes SNPs present within a discrete region of the S. enterica genome that is located close to the gene dkgB and that encompasses a 5S ribosomal subunit and flanking regions. The dkgB-linked ISR method, including primers and steps in processing, has been described in detail (Guard, Morales, Fedorka-Cray, & Gast, 2011). Briefly, DNA was isolated from cultures suspected of being Salmonella on selective media described in Section 2.3. A first set of primers, one of which was specific to the dkgB gene, was used to produce an amplicon. A second set of sequencing primers were then used to produce sequence from the amplicon in both forward and reverse directions. DkgB-linked ISR sequences reliably distinguish SE from KY, and its application for otherwise serotyping mixtures of S. enterica subspecies I occurring on-farm has been described (Pulido-Landinez, Sanchez-Ingunza, Guard, & do Nascimento, 2014; Pulido-Landinez et al., 2013, 2014). In these experiments, it was also used to make sure that no other Salmonella serotypes than what was introduced into the hen house were recovered.
3. Results
3.1. Impact of mixed infection on egg production
Hens that were exposed only to SE by contact infection (SE-CN) had the highest level of production (Fig. 2), and this was an expected outcome because SE is known to stimulate egg production at low dosages. In contrast, hens from group SE-IM had the lowest egg production (Fig. 2), which again was an expected outcome because systemic challenge with SE suppresses egg production. Prior exposure of hens by mouth to KY mitigated the extreme suppression in egg production seen with hens infected IM with SE only (KY-PO:SE-IM vs SE-IM, P < 0.05) (Fig. 2). Hens also had significantly lower (P < 0.01) egg production when contact exposed (CN) to KY prior to contact exposure to SE (KY-CN:SE-CN versus SE-CN) (Fig. 2). Egg production is thus perhaps a sensitive barometer for assessing interactions between Salmonella serotypes, but further research is needed to explore parameters associated with changing combinations of KY and SE. To summarize, prior exposure of hens to KY by mouth mitigated extremes in egg production seen when hens were exposed to SE alone by contact or systemic infection (see Fig. 3).
3.2. Recovery of Salmonella from internal organs
Table 1 shows results from culturing organs on three different days post-infection and Table 2 shows results for total number of samples collected per organ type. Only SE was recovered from normally sterile organs, which is an expected result because the ability of Salmonella to invade internal tissues of the hen is a parameter of pathobiology that indicates risk of serotypes for causing foodborne illness.
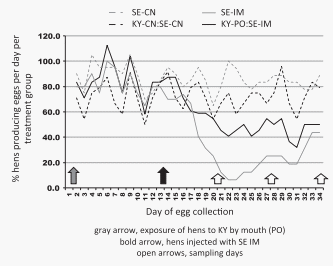
Fig. 2. Percent daily egg production of hens in treatment groups that vary in exposure to KY and SE. Treatment group key: SE-IM, solid gray; KY-PO:SE-IM, solid black; KY-CN:SE-CN, hatched black; SE-CN, hatched gray. Data begin the day after hens were exposed to KY by mouth (PO). Contact infected (CN) hens were located in cages below PO infected hens. The solid gray arrow indicates the day hens were exposed to KY PO, and the solid black arrow on the X-axis indicates the day that SE was injected intramuscularly (IM). Open arrows on the X-axis indicate sampling dates at 6, 13 and 20 days post-infection of hens with SE. Graph lines show a 2-day moving average, because time of collection of eggs varied somewhat during the day. Percent of hens producing eggs can exceed 100% due to lay of more than one egg per day.
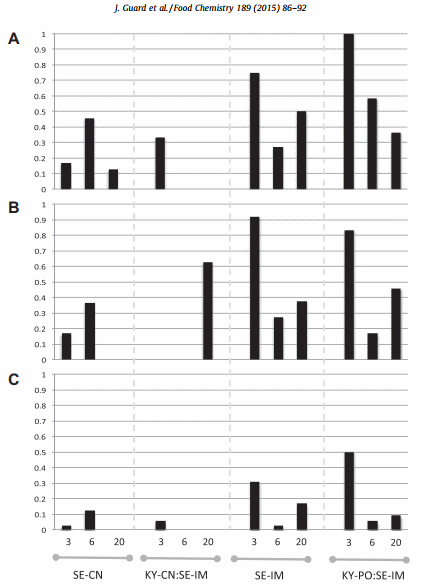
Fig. 3. Recovery of SE at days 6, 13 and 20 post-infection from hens that vary in exposure to KY and SE. Percent of samples (100) that were positive for SE per sampling day post-infection is indicated by bars and raw data are shown in Table 1. (A) Spleens; (B) livers; (C) compilation of all oviduct samples (upper, middle, lower). Treatment groups listed on the X-axis are described in text.
SE was recovered from liver, spleen and at least one oviduct sample at days 6, 13 and 20 for all hens injected IM with SE (group SE-IM) (Table 1). Only the upper oviduct of contact infected hens had no recoverable SE on any of the sampling days (Table 1). Treatment groups varied in which day yielded the highest percentage of positive samples, with days 6 and 13 yielding the most useful comparisons. For example, group SE-CN had 23.6% positive organ samples on day 13 (13/55), whereas days 6 and 20 had respectively 8.3% and 2.5% positives. Group KY-CN:SE-CN had 12.5% and 10% positive internal organs on days 20 and 6, respectively, but no positives were recovered on day 13. Hens in group SE-IM had 51.7%, 12.7% and 27.5% internal organs positive on days 6, 13 and 20, respectively, which appeared similar to hens in group KY-PO:SEIM with 66.7%, 18.3% and 21.7% positives on the same days. Further experimentation is needed to discern if there is a decrease in positive samples from internal organs on the 13th day post-infection as suggested by results from 3 of the 4 sampling groups. Only SE-CN hens had a relative peak in incidence of positive organs on day 13.
Recovery of SE from oviduct varied per sampling day and samples from days 13 and 20 post-infection for group KY-PO:SE-CN were negative for SE. Overall, group KY-CN:SE-CN had the fewest days where SE was recovered for any organ type (Table 2). An unexpected finding is that group KY-PO:SE-IM had the highest frequency of recovery of SE from samples, and upper oviduct was nearly 8-fold more likely to be positive for this group than for hens infected IM with SE alone, with respective values of 22.9% and 3.2% (Table 2). Group KY-PO:SE-IM also had the highest percentage of positive spleens (65.7%), but it is not known how this may have impacted the oviduct or if group SE-IM had significantly fewer positive spleens (51.6%).
Systemic infection of hens with SE (group SE-IM) resulted in 54.8% and 51.6% positive livers and spleens, respectively (Table 2). Prior exposure of hens to KY before systemic infection with SE (group KY-PO:SE-IM) appeared to produce similar results, with 48.6% and 65.7% of livers and spleens positive. Hens exposed to SE by contact (SE-CN) had 19.4% and 25.8% positive livers and spleens, indicating that contact infection resulted in organ invasion frequently and at low environmental dosages. Hens that were contact infected with both serotypes (KY-CN:SE-CN) had the lowest incidence of reticuloendothelial system organ invasion, with 15.6% and 12.5% of livers and spleens positive. The total percentages of SE samples were substantially lower for reproductive tract organs as compared to liver and spleen (Table 2). For example, only 3.2% of upper oviduct samples were positive in the SE-IM group and none of the regions of the oviduct on a given day for contact infected hens surpassed 10% positive for SE. There appeared to be some uniformity of results for most of the oviduct samples when SE was given IM, which ranged from 20% to 25.8% positive for SE, with the exception for upper oviduct in the SE-IM group as already noted (Table 2).
3.3. Recovery of Salmonella from fecal samples and cecal tonsils
Two types of samples were processed that had complex microbial floras and these were cecal tonsils and floor droppings. Table 1 includes columns showing results from cecal tonsils. No Salmonella was recovered from cecal tonsils collected from the SE-CN group. Recovery of SE from the SE-IM group only occurred on day 6 post-infection. Hens that had been exposed to KY frequently had positive cecal tonsils. Of 32 total cecal tonsils collected from group KY-CN:SE-CN, 5 (15.6%) were positive and all were KY. For the 35 cecal tonsils collected from group KY-PO:SE-IM, 6 (17.1%) were positive for Salmonella, and 4 of these were KY. Of 120 samples of floor droppings collected over the course of the experiment, 7 (5.8%) were positive for Salmonella and all isolates were KY. These results suggest that KY has an advantage for emerging as a dominant serovar in the cecal tonsils of chickens and in the poultry environment in comparison to SE.
4. Conclusion
It is common to encounter multiple Salmonella serotypes on farm and in the environment (Basler et al., 2014; Oscar, Rutto, Ludwig, & Parveen, 2010; Pulido-Landinez et al., 2013; Thomas, Slawson, & Taylor, 2013). However regulatory agencies often focus on identification of single serotypes, and subtypes within serotype, to establish causation of anyone food-borne outbreak (Mody et al., 2011). While there are reasons to identify the most dominant serotype and its prevalence within outbreaks, research described here is focused on building an understanding of why the United States has not been able to reduce its baseline incidence of SE over the past 5 years (Fig. 1). We suggest that a more encompassing view of S. enterica on-farm and in the food supply is needed, and it should in part consider how multiple serotypes on-farm might either facilitate or impede outbreaks (Gicquelais et al., 2014).
Two serotypes that vary in pathogenicity and ecology on-farm were initial subjects for exploring this issue, namely SE and KY. Many potential pairings are of interest, but combining KY and SE explored aspects of mixing the confirmed pathogen SE with the environmental colonizer KY. Examples of other pairings of interest would include mixtures of S. Gallinarum, S. Enteritidis and S. Isangi that were associated with exceptional morbidity and mortality in flocks (Pulido-Landinez et al., 2014). Recovery of this group of serotypes from the affected farms raises the question of the role of S. Isangi in moderating or worsening dynamics of infection on-farm, as well as questions about interactions between broad-spectrum and host-restricted pathogens such as SE and S. Gallinarum, respectively. Other mixtures of serotypes of interest include S. Heidelberg, S. Newport and S. Infantis. While the combinatorial complexity of Salmonella serotypes is challenging, concentrating on more commonly encountered co-habitants with distinguishable epidemiological trends will facilitate building a framework for understanding if a flora of multiple serotypes impacts outbreaks of food-borne disease.

In order to investigate if the presence of multiple Salmonella serotypes on-farm alter overall epidemiological potential of any Table 1 Results on different days for organs cultured from hens that varied in exposure to one serotype, we thus explored an experimental design that included low dose exposure somewhat analogous to what might happen in the field. Results here suggest that high dose infections may not allow observation of nuanced outcomes associated with mixtures of serotypes, because SE was recovered from organs collected from both SE-IM and KY-PO:SE-IM groups at similarly high levels. However, mixing high-dose exposed hens (SE-IM and KYPO:SE-IM) in a 50:50 ratio with hens left to be contact infected (SE-CN and KY-CN:SE-CN) facilitated an incidence of infection in low dose groups that could be monitored within a small sampling group. It is desirable to eventually reduce the number of deliberately infected hens used to initiate successful contact infection. However, results support that the number of hens in this investigation was an appropriate starting point for the experimental objective of studying impact of mixtures of Salmonella serotypes on the avian host.
Egg production may be especially sensitive to monitoring the impact of multiple serotypes. Exposure of the mature hen to SE can either increase or decrease egg production (Guard-Bouldin & Buhr, 2006). Suppression is most frequently observed and it is a typical outcome in experimental situations following systemic infection with dosages above 106 CFU per hen. However, contact infection and even some lower dose systemic challenges can increase egg production. Part of the reason SE may alter egg production is that it has a profound impact on serum calcium levels, which invokes a parathyroid hormonal axis and thus physiological outcome can vary according to details of exposure (Morales et al., 2012).
Liver and spleen are non-reproductive tract organs associated with the reticuloendothelial system that generate, harbor and eventually clear phagocytic cells. As such, they are part of the innate defense of the host that helps to remove pathogens from the blood supply. Pathogenic S. enterica serotypes can survive phagocytosis, which makes liver and spleen especially important for monitoring the outcome of infection.
The experimental design successfully mirrored some real-world epidemiology of both KY and SE. Specifically, (i) KY was more easily recovered from cecal tonsils than SE, (ii) only SE invaded organs, and (iii) KY was easily recovered from droppings. An unexpected result that requires further consideration is that prior exposure of hens by mouth to high dosage of KY followed by systemic infection with SE (group KY-PO:SE-IM) gave the highest percentage of positive spleens and upper oviducts. One explanation is that artifi- cially high infectious dosages do not represent what happens in field situations. For example, low dose contact exposure in group KY-CN:SE-CN had the least positive internal organs. Another explanation is that the host immune system was compromised by experimental design exposing the hens to two serotypes in the manner described. We suggest that this latter explanation again suggests high dosages given in group KY-PO:SE-IM had unexpected consequences for the host. In summary, we suggest we have developed useful guidelines and a feasible experimental design for investigating if mixtures of serotypes have potential to impact ecology of S. enterica on-farm and possibly food-borne outbreaks. Future experiments may be improved by reducing dosage to avoid compromise of the host’s ability to respond to infection in a manner that best reflects challenges experienced during production.
Acknowledgements
This research was funded by the U.S. Department of Agriculture, Agricultural Research Service as part of CRIS#6612-32000-007-00, which addresses USDA-REE Goal 5: Food Safety and USDA-REE Goal 1: Local and Global Food Supply and Security (Subgoal 1D: Consumer and Industry Outreach, Policy, Markets, and Trade).
References
Basler, C., Forshey, T. M., Machesky, K., Erdman, M. C., Gomez, T. M., Nguyen, T. A., et al. (2014). Multistate outbreak of human Salmonella infections linked to live poultry from a mail-order hatchery in Ohio – March–September 2013. MMWR. Morbidity and Mortality Weekly Report, 63, 222.
Braden, C. R. (2006). Salmonella enterica serotype Enteritidis and eggs: A national epidemic in the United States. Clinical Infectious Diseases: An official publication of the Infectious Diseases Society of America, 43, 512–517.
FSIS. Serotypes Profile of Salmonella Isolates from Meat and Poultry Products: January 1998 through December 2011. In. U.D.o.A. Food Safety and Inspection Service, ed. USDA, http://www.fsis.usda.gov. p 121. 2011.
Gantois, I., Ducatelle, R., Pasmans, F., Haesebrouck, F., Gast, R., Humphrey, T. J., et al. (2009). Mechanisms of egg contamination by Salmonella Enteritidis. FEMS Microbiology Reviews, 33, 718–738.
Gicquelais, R. E., Morris, J. F., Matthews, S., Gladden, L., Safi, H., Grayson, C., et al. (2014). Multiple-serotype Salmonella outbreaks in two state prisons–Arkansas, August 2012. MMWR. Morbidity and Mortality Weekly Report, 63, 169–173.
Gould, L. H., Walsh, K. A., Vieira, A. R., Herman, K., Williams, I. T., Hall, A. J., et al. (2013). Surveillance for Foodborne Disease Outbreaks – United States, 1998– 2008. Morbidity and Mortality Weekly Report (MMWR), 62, 1–34.
Guard, J., Gast, R. K., & Guraya, R. (2010). Colonization of avian reproductive-tract tissues by variant subpopulations of Salmonella enteritidis. Avian Diseases, 54, 857–861.
Guard, J., Morales, C. A., Fedorka-Cray, P., & Gast, R. K. (2011). Single nucleotide polymorphisms that differentiate two subpopulations of Salmonella enteritidis within phage type. BMC Research Notes, 4, 369.
Guard, J., Sanchez-Ingunza, R., Morales, C., Stewart, T., Liljebjelke, K., Van Kessel, J., et al. (2012). Comparison of dkgB-linked intergenic sequence ribotyping to DNA microarray hybridization for assigning serotype to Salmonella enterica. FEMS Microbiology Letters, 337, 61–72.
Guard, J., Shah, D. H., Morales, C. A., & Call, D. R. (2011). Evolutionary trends associated with niche specialization as modeled by whole genome analysis of egg-contaminating Salmonella enterica serovar Enteritidis. Salmonella: From Genome to Function (pp. 91–106). Norfolk, UK: Caister Academic Press.
Guard-Bouldin, J., & Buhr, R. J. (2006). Evaluation of eggshell quality of hens infected with Salmonella enteritidis by application of compression. Poultry Science, 85, 129–135.
Guard-Petter, J. (1998). Variants of smooth Salmonella enterica serovar Enteritidis that grow to higher cell density than the wild type are more virulent. Applied and Environmental Microbiology, 64, 2166–2172.
Guard-Petter, J. (2001). The chicken, the egg and Salmonella enteritidis. Environmental Microbiology, 3, 421–430.
Joerger, R. D., Sartori, C. A., & Kniel, K. E. (2009). Comparison of genetic and physiological properties of Salmonella enterica isolates from chickens reveals one major difference between serovar Kentucky and other serovars: Response to acid. Foodborne Pathogens and Disease, 6, 503–512.
Kinde, H., Read, D. H., Chin, R. P., Bickford, A. A., Walker, R. L., Ardans, A., et al. (1996). Salmonella enteritidis, phase type 4 infection in a commercial layer flock in southern California: Bacteriologic and epidemiologic findings. Avian Diseases, 40, 665–671.
Le Hello, S., Hendriksen, R. S., Doublet, B., Fisher, I., Nielsen, E. M., Whichard, J. M., et al. (2011). International spread of an epidemic population of Salmonella enterica serotype Kentucky ST198 resistant to ciprofloxacin. The Journal of Infectious Diseases, 204, 675–684.
Mody, R. K., Greene, S. A., Gaul, L., Sever, A., Pichette, S., Zambrana, I., et al. (2011). National outbreak of Salmonella serotype saintpaul infections: Importance of Texas restaurant investigations in implicating jalapeno peppers. PloS One, 6, e16579.
Morales, C. A., Guard, J., Sanchez-Ingunza, R., Shah, D. H., & Harrison, M. (2012). Virulence and metabolic characteristics of Salmonella enterica serovar enteritidis strains with different sefD variants in hens. Applied and Environmental Microbiology, 78, 6405–6412.
Oscar, T. P., Rutto, G. K., Ludwig, J. B., & Parveen, S. (2010). Qualitative map of Salmonella contamination on young chicken carcasses. Journal of Food Protection, 73, 1596–1603. Parker, C. T., Liebana, E., Henzler, D. J., & Guard-Petter, J. (2001). Lipopolysaccharide O-chain microheterogeneity of Salmonella serotypes Enteritidis and Typhimurium. Environmental Microbiology, 3, 332–342.
Pulido-Landinez, M., Sanchez-Ingunza, R., Guard, J., & do Nascimento, V. P. (2013). Assignment of serotype to Salmonella enterica isolates obtained from poultry and their environment in southern Brazil. Letters in Applied Microbiology, 57, 288–294.
Pulido-Landinez, M., Sanchez-Ingunza, R., Guard, J., & do Nascimento, V. P. (2014). Presence of Salmonella enteritidis and Salmonella gallinarum in commercial laying hens diagnosed with fowl typhoid disease in Colombia. Avian Diseases, 58, 165–170.
Pulido-Landinez, M., Washington, P., Thornton, J. K., Zhang, Y., Sanchez-Ingunza, R., Banda, A., et al. (2014). Serotype and antimicrobial resistance patterns of Salmonella isolates from commercial birds and poultry environment in Mississippi. Avian Diseases, 58, 64–70.
Schroeder, C. M., Latimer, H. K., Schlosser, W. D., Golden, N. J., Marks, H. M., Coleman, M. E., et al. (2006). Overview and summary of the Food Safety and Inspection Service risk assessment for Salmonella enteritidis in shell eggs, October 2005. Foodborne Pathogens and Disease, 3, 403–412.
Shuren, J. (2009). Prevention of Salmonella Enteritidis in shell eggs during production, storage and transport 21 CFR Parts 16 and 118. H.. Food and Drug Administration (pp. 33101). USA: Federal Register. Thomas, J. L.,
Slawson, R. M., & Taylor, W. D. (2013). Salmonella serotype diversity and seasonality in urban and rural streams. Journal of Applied Microbiology, 114, 907–922.