Mycoplasmas are bacteria characteristic for their lack of a cell wall, small size, and small genome (1). These pathogens are evolutionarily related to the low-%GC Gram-positive Clostridiales (2), and they are highly host specific and tend to inhabit mucosal surfaces within their host (1). Within the poultry industry, the ability of Mycoplasma gallisepticum to infect the respiratory and reproductive tracts of several avian species has made it a pathogen of great economic concern (3). In an effort to manage the disease, M. gallisepticum strain F (4, 5) ts-11 (6, 7), and 6/85 (8) live vaccines were developed. The F strain is a naturally attenuated field isolate that was first discovered in the 1950s, while ts-11 and 6/85 were commercially produced using serial passage or chemical mutagenesis (7). These vaccine strains exhibit various degrees of effectiveness and safety (9–11), and there is evidence that some of these M. gallisepticum vaccine strains can revert to virulence (9, 12, 13). Currently, the genetic basis behind the attenuation of the vaccine strains is not well understood. In addition, strain differentiation among vaccine strains and natural isolates has proven complex (14–17), making it difficult to determine if avian mycoplasmosis infection in vaccinated flocks is due to infection with an M. gallisepticum strain type similar to the vaccine (18), reversion of the vaccine strain (9), or mixed infection with the vaccine and a related strain type (19).
M. gallisepticum isolates vary widely in their relative degrees of pathogenicity in animal challenge experiments, depending on the route of infection and the number of in vitro passages (20–22). Serial passaging of this organism in vitro has been used to create attenuated strains for use as vaccines (7), but the likelihood of reversion to wild-type virulence is inherent to this attenuation method. It has also been difficult to differentiate vaccine M. gallisepticum strains from some field isolates (12, 13, 23). This is important in order to differentiate M. gallisepticum field infections from vaccine exposures in a timely manner and, also, in order to assess the reversion to virulence of vaccine strains.
The genome of Mycoplasma is relatively small compared to other bacterial genomes; the average size of Mycoplasma genome is 1.0 Mb (the range is from 580 kb to 1,380 kb) (24), one quarter of the average size of an Escherichia coli genome. Historically, Mycoplasma genitalium was the second complete bacterial genome ever published (25), and since then, over 50 Mycoplasma genomes, including pathogens of humans, animals, and plants, have been reported, including M. gallisepticum (26). Despite these facts, compared to other pathogens, few virulence-related genes have been identified in M. gallisepticum. GapA (a primary cytadhesin) and CrmA (an accessory cytadhesin) mediate the attachment of this pathogen to the respiratory epithelium of the host (27). VlhA is a surface lipoprotein that undergoes phase variation; changing the bacterial surface architecture and allowing the mycoplasmas to escape immune surveillance (28, 29). There are also several metabolic pathways important to M. gallisepticum virulence; M. gallisepticum has been shown to depend on the dihydrolipoamide dehydrogenase (Lpd), a component of the pyruvate dehydrogenase complex, for host colonization and pathogenesis (30). The expression of MalF, an ABC transporter, has also been shown to be essential for persistence (31).
In 2007, several broiler breeder flocks in northeastern Georgia were vaccinated with ts-11 vaccine to control an ongoing M. gallisepticum outbreak. Between 2008 and 2011, severe respiratory disease associated with M. gallisepticum infection was observed in the broiler progeny of several ts-11-vaccinated breeder flocks. M. gallisepticum isolates from the broilers and their parents were indistinguishable from the ts-11 vaccine strain by the genotyping methods used and were termed “ts-11-like” isolates (9). The epidemiology of the outbreaks, as well as genotyping and pathogenicity results, indicate that an increase in virulence and vertical transmission of ts-11 vaccine occurred and that the ts-11-like isolates were very likely revertants derived from ts-11 vaccine (9, 16, 32). In order to identify M. gallisepticum ts-11-specific marker alleles, whole-genome sequencing was used. M. gallisepticum DNA was sequenced using both Illumina and 454 sequencing methods and compared to the sequence of M. gallisepticum strain R (Rlow), a well-documented reference strain that is virulent in chickens (26, 29). In this study, the use of comparative genomics to identify strain-specific marker sequences that can distinguish between the M. gallisepticum ts-11 vaccine strain and natural field isolates is demonstrated.
RESULTS
Of the 803 annotated M. gallisepticum ts-11 genes, 70 were identified as having homology with putative virulence genes, including those involved in adherence. There was significant genetic diversity in two adhesins, encoded by gapA (Fig. 1) and mcg2 (data not shown), among the analyzed M. gallisepticum genomes. No major genomic changes were identified among ts-11 avirulent and virulent isolates; there were numerous single-nucleotide polymorphisms (SNPs) that need to be further analyzed and confirmed. The gene sequences of gapA and mcg2 were conserved in M. gallisepticum ts-11 isolates, except for the presence of a 20-bp insertion in gapA of the vaccine strain ts-11. Forty of the virulence genes identified in the M. gallisepticum ts-11 genome were annotated as coding for the variable surface protein VlhA, and these vlhA genes mapped to one of 6 loci within the ts-11 genome. Two of these loci are depicted in Fig. 2, and vlhA locus 1, which contains strain-specific sequences, is highlighted. Comparison of the M. gallisepticum vlhA genes among M. gallisepticum strains identified several vlhA genes containing sequences that were unique to ts-11 strains (Fig. 3). The vlhA alleles containing sequences unique to the ts-11 isolates included vlhA3.04a, vlhA3.04b, vlhA3.04d, vlhA3.05c, vlhA3.08a, and vlhA5.03b. In addition to these vlhA alleles, several other genes were identified that were unique to the ts-11 isolates, contained strain-specific sequences, or were limited in their distribution among M. gallisepticum genomes. Of these genes and gene sequences, five likely candidates were selected for strain-specific-typing PCR tests: vlhA3.04a, vlhA3.04b, vlhA3.05, mg0359, and mg0377. The PCR protocols were optimized, and no evidence of nonspecific amplification or additional bands was identified when the gels were analyzed (Fig. 4). A summary of the PCR analysis of the M. gallisepticum isolates is presented in Table 1. All M. gallisepticum ts-11 isolates were positive for all five alleles tested by PCR (vlhA3.04a, vlhA3.04b, vlhA3.05, mg0359, and mg0377). There was a significant difference (P 0.005) in the distribution of these alleles in M. gallisepticum isolates (ts-11 versus field isolates). However, no single allele was unique to the ts-11 strain compared to the field isolates screened in this study. The distribution of the alleles in field isolates ranged between 5% and 36%. The vlhA3.05 PCR had the highest discriminatory power, followed by the mg0359 PCR (5% and 7% of non-ts-11 isolates were positive, respectively). A combination of primers (vlhA3.04a, vlhA3.05, and mg0359) was able to differentiate ts-11 isolates from M. gallisepticum field isolates (100% versus 0%, respectively).
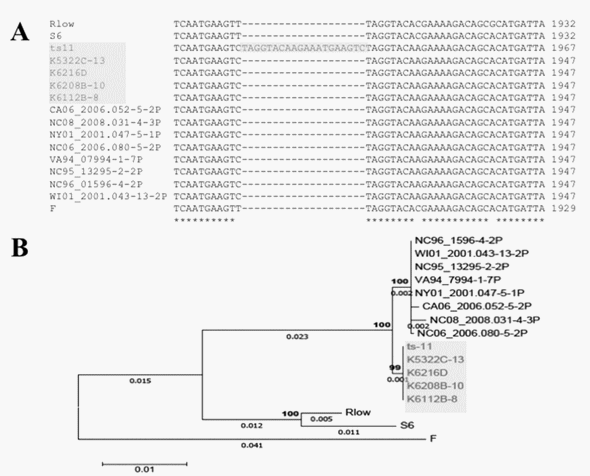
FIG 1 Sequence alignments and phylogenetic tree based on gapA adhesin. (A) A sequence alignment identified a 20-bp insertion (shaded in gray) in M. gallisepticum ts-11 within a conserved region of gapA. (B) Phylogenetic analysis confirmed that the gene sequences for gapA were identical in the M. gallisepticum ts-11 isolates (shaded in gray), except for this 20-bp insertion in the vaccine strain. The phylogenetic tree was constructed using the maximum-likelihood method based on the Tamura-Nei model. The tree is drawn to scale, with branch lengths measured in the number of substitutions per site (next to the branches). Bootstrap percentages after 1,000 replications and branch lengths of 0.001 are shown. The ts-11 isolates are shaded in gray.
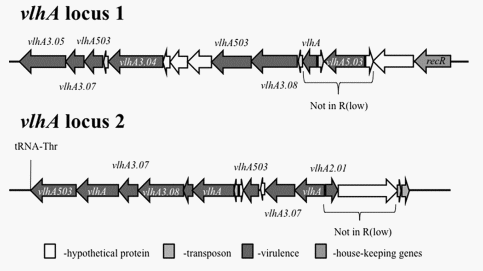
FIG 2 Identification of Mycoplasma gallisepticum ts-11 vlhA virulence loci. Forty of the virulence genes identified in M. gallisepticum were annotated as coding for the variable surface protein VlhA. These vlhA genes mapped to 6 loci within the ts-11 genome. Two of these loci are depicted, and locus 1 is of particular importance in that it houses the vlhA genes that were targeted for differentiating the ts-11 strain from natural field isolates.
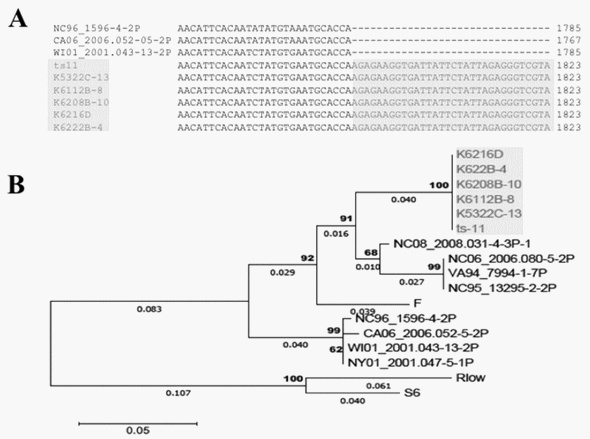
FIG 3 Identification of Mycoplasma gallisepticum ts-11-specific sequences in vlhA3.04b. (A) Sequences unique to the ts-11 isolates (shaded in gray) are contained in the 3= region of this vlhA3.04b gene allele. (B) Phylogenetic analysis confirmed that M. gallisepticum ts-11 isolates (shaded in gray) grouped together due to conserved sequences. The phylogenetic tree was constructed using the maximum-likelihood method based on the Tamura-Nei model. The tree is drawn to scale, with branch lengths measured in the number of substitutions per site (next to the branches). Bootstrap percentages after 1,000 replications and branch lengths of 0.001 are shown.
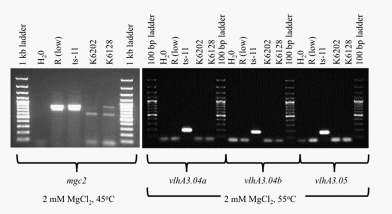
FIG 4 PCR to differentiate between ts-11 and field isolates. Five alleles were tested by PCR (vlhA3.04a, vlhA3.04b, vlhA3.05, mg0359, and mg0377). This gel depicts the results of three PCR tests (vlhA3.04a, vlhA3.04b, and vlhA3.05). K6202 and K6218 are field isolates (not ts-11 strain), and both were negative in the three PCR tests. All of the M. gallisepticum isolates were positive in the mgc2 PCR.
DISCUSSION
The inability to distinguish M. gallisepticum field isolates from vaccine strains has made it difficult to understand the epidemiology of avian mycoplasmosis, especially when there is an outbreak involving vaccinated flocks. Methods that employ DNA fingerprinting require the isolation of pure cultures (15) and can only differentiate M. gallisepticum ts-11 from a few other strains (14, 33). Other methods, such as genetargeted sequencing, require the use of PCR followed by sequencing and sequence alignments (23), a time- and resource-intensive process.
Although no major genomic differences that could differentiate ts-11 virulent from ts-11 avirulent isolates were identified, this study revealed that the M. gallisepticum ts-11 vaccine and ts-11 isolates, obtained from the field and from ts-11 animal passage experiments, exhibited genomic differences compared to the M. gallisepticum Rlow genome in the form of insertions/deletions of sequences. In some cases, entire gene islets (consisting of up to four genes) were seen in ts-11 isolates that are absent in Rlow. vlhA locus 1 contained a large number of vlhA genes that were shown to contain genotypic differences among the strains. This was essential in allowing for the identification of sequences that distinguish the M. gallisepticum ts-11 strain from field isolates. Phylogenetic analysis, carried out using each of these strain-typing candidate genes, showed that all the ts-11 isolates clustered, indicating there was sufficient sequence variability to formulate a discriminatory PCR-based test.
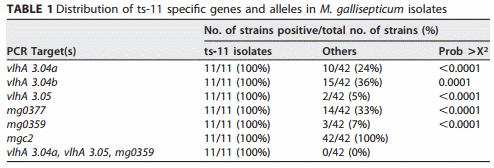
Multiple sequence alignments allowed for the rapid identification of genomic differences between the M. gallisepticum ts-11 isolates and the virulent Rlow strain. One such difference was the identification of a 20-bp insertion in gapA of the vaccine strain ts-11. This insertion has been predicted to disrupt the protein and affect the adherence capability of M. gallisepticum, and consequently, this insertion in GapA affects the colonization of chickens by the vaccine strain (34). The insertional mutation was lost in the M. gallisepticum ts-11 field isolates, suggesting that the vaccine can revert to wild type. Studies have reported reversion of GapA- to GapA+ when ts-11 is passaged in chickens, resulting in an increased ability of the bacteria to colonize. However, GapA+ ts-11 isolates are not pathogenic (18, 34), indicating that additional changes are necessary for full reversion to the virulent phenotype.
Most of the other genes identified as candidates for ts-11-specific detection were vlhA genes. These encode variable surface lipoproteins and contain significant interstrain sequence variation (16). This genetic diversity is not surprising, as mycoplasmas have been known to have high mutation rates (24). This variability is introduced by various mechanisms, such as insertions, deletions, or rearrangements. vlhA locus 1 is the main locus in which many vlhA genes exhibited significant sequence diversity. The vlhA3.04a, -3.04b, and -3.05 genes from this locus were chosen because they contained sequences that were unique to the M. gallisepticum ts-11 isolates and highly conserved within this group. The vlhA3.05 gene possessed significant differentiating power, identifying all ts-11 isolates (all of these were positive), while only 5% of the field isolates tested positive. To achieve optimal discriminatory power, a combination of vlhA3.05, mg0359, and vlhA3.04a PCR tests was effective in differentiating M. gallisepticum ts-11 isolates from natural field isolates. This study describes a useful molecular assay that can differentiate at a high level between the M. gallisepticum ts-11 strain and field isolates. This will supplement current tools available for diagnostics and is necessary for determining strain interactions, evaluating vaccination programs, and controlling the spread of M. gallisepticum within poultry complexes. This work will allow differentiation between an attenuated vaccine strain and field strains, thereby providing the tools necessary to rapidly recognize field challenges.
MATERIALS AND METHODS
M. gallisepticum isolates. The ts-11 vaccine and ts-11 reisolates used in this study are listed in Table 2. The vaccine was obtained from the manufacturer (Mycoplasma gallisepticum vaccine, serial no. MA649; Merial Select, Gainesville, GA, USA). The field isolates were recovered from clinical submissions to the Poultry Diagnostic and Research Center (PDRC), University of Georgia, Athens, GA USA, during a period from October 2007 to February 2010. The isolates were genotyped as ts-11 strains based on multiple genetic analyses, including targeted sequencing and random amplified polymorphic DNA (RAPD) analysis (9). The clinical picture was obtained from history at submission and/or interviews with field veterinarians. Bird trials confirmed the pathogenicity of two of the isolates (K6212D and K6222B) (9, 32). One reisolate (K5322C-13) was obtained from specific-pathogen-free layer-type chickens at 2 weeks postvaccination with ts-11. Three additional M. gallisepticum ts-11 isolates from the field were tested by the PCR protocols developed, as well as 42 additional strains, including 6 reference (PG-31, Rlow, A5969, and S-6) and vaccine (F and 6/85) strains and 15 field isolates (1998 to 2011) from the United States. International isolates from Jordan (2 isolates), India (2 isolates), Israel (2 isolates), Slovenia (6 isolates), Brazil (1 isolates), Germany (3 isolates), Mexico (1 isolates), and the Netherlands (4 isolates) were also tested. These M. gallisepticum isolates were obtained from the PDRC repository
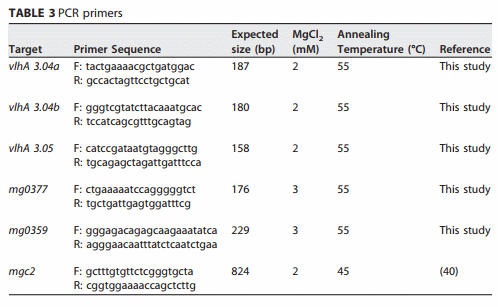
DNA extraction. M. gallisepticum isolates were grown as previously described (9). DNA was extracted from M. gallisepticum isolates as follows: after growth in Frey’s modified broth, cells were centrifuged at 13,000 x g for 3 min, the supernatant discarded, and the cell pellets reconstituted in 200 l of phosphate-buffered saline (pH 7). Genomic DNA was extracted using the Qiagen DNeasy blood and tissue kit (Qiagen, Valencia, CA) following the manufacturer’s recommendations.
Whole-genome sequencing, alignment, and annotation. The sequenced isolates are listed in Table 2. Whole-genome libraries were produced using Illumina, following the manufacturer’s protocols to produce paired-end 151-bp reads. Raw sequence data were screened for adapter contamination and quality using FastQC (http://www.bioinformatics.babraham.ac.uk/projects/fastqc/). The sequence libraries were quality trimmed to remove low-quality bases using FastaQ/A Trimmer in the FASTX-Toolkit (http://hannonlab.cshl.edu/fastx_toolkit/index.html). The best k-mer length for de novo assembly was determined using KmerGenie (35). De novo assembly of paired-end Illumina reads was done using Velvet (36). Reads obtained by the Roche 454 sequencing protocol were assembled using GS De Novo Assembler (http://www.454.com/products/analysis-software/). Contigs from each isolate library were ordered against the complete genome of M. gallisepticum Rlow (GenBank accession number NC_004829.2), which served as the reference genome for this study (26), using the Mauve Genome Alignment Tool (37). Contigs were renamed using Perl scripting to reflect the order derived from the reference genome. Each library was then annotated using the RAST annotation server.
Bioinformatic analysis. Sequence-based analysis of the annotated genomes was accomplished using RAST Seed Viewer (http://theseed.org). Each isolate’s genome was compared to the M. gallisepticum Rlow reference genome in order to identify genes that were absent in Rlow, present in M. gallisepticum ts-11 vaccine and ts-11 reisolate genomes, or had 80% similarity to the corresponding gene in the reference genome. Jalview (38) was used to create multiple sequence alignments of each candidate ts-11-specific gene, comparing the genomes of M. gallisepticum ts-11 vaccine strain and ts-11 isolates to published M. gallisepticum genomes (strain S6, GenBank accession number NC_023030.1; strain F, GenBank accession number NC_017503.1; and house finch isolates, GenBank accession numbers NC_018412.1, NC_018409.1, NC_018406.1, NC_018407.1, NC_018408.1, NC_018410.1, NC_018411.1, and NC_018413.1). Nucleotide sequences found to be conserved in the ts-11 vaccine strain and ts-11 reisolates were then searched against the NCBI database using BLAST to test their specificity in silico. Phylogenetic trees were constructed using the neighbor-joining method (Jalview) and the maximum likelihood method based on the Tamura-Nei model in MEGA6 (39) in order to assess the evolutionary similarities among the M. gallisepticum ts-11 vaccine strain, the ts-11 reisolates, and other M. gallisepticum isolates. Nucleotide and amino acid sequences for M. gallisepticum strain-specific genes/alleles, as well as gapA and mgc2, were submitted to National Center for Biotechnology Information (NCBI) GenBank (http://www.ncbi.nlm.nih.gov/GenBank/) under GenBank accession numbers KU577580 to KU577613. In addition, the M. gallisepticum whole-genome sequences were deposited with NCBI GenBank (Bioproject accession number PRJNA325637).
PCR. PCR primers were designed with the web-based software Primer3Plus (http://www.bioinformatics. nl/cgi-bin/primer3plus/primer3plus.cgi), using strain-specific sequences identified in vlhA3.04a, vlhA3.04b, vlhA3.05, mg0359, and mg0377. PCR was performed using a RapidCycler thermocycler (Idaho Technologies; Idaho Falls, ID) (40) in 10-l-capacity capillary tubes; the primers are listed in Table 3. The PCR mixture consisted of 0.2 mM deoxynucleoside triphosphates (dNTPs), 2.0 mM MgCl2 (with vlhA3.04a, vlhA3.04b, vlhA3.05, and mgc2 primers) or 3.0 mM MgCl2 (with mg0359 and mg0377 primers), 0.5 M each primer, 0.5 unit of Taq DNA polymerase (Invitrogen, Waltham, MA), and 1 l DNA template in a 10-l volume. The program parameters for the thermocycler were 94°C for 0 s, 55°C for 0 s, and 72°C for 15 s with a slope of 2.0 for 30 cycles. For mgc2, the program parameters were 94°C for 1 min, then 94°C for 10 s, 45°C for 10 s, and 72°C for extension for 35 s with a slope of 2.0 for 30 cycles. TA
Amplicons were separated by gel electrophoresis in a 1.5% agarose gel (15 by 10 cm) with 0.5 g ethidium bromide/ml and 1x TAE (40 mM Tris, 20 mM acetate, 1 mM EDTA, pH 8) buffer at a constant voltage of 80 V for 60 min. A 100-bp ladder (DNA molecular weight marker; Roche, Indianapolis, IN) was used as the molecular weight (MW) standard for determining the MW of the PCR amplicons. The agarose gels were exposed to ultraviolet light and photographed using a digital photo documentation system (Molecular Imager Gel Doc XR system; Bio-Rad Laboratories, San Diego, CA). The discriminatory power of each PCR to distinguish the vaccine strain (ts-11) from field isolates of M. gallisepticum was determined by Pearson’s chi-square test in JMP Statistics Made Visual (SAS Institute, Inc., Cary, NC) (P 0.005).
ACKNOWLEDGMENTS
This research received no specific grant from any funding agency in the public, commercial, or not-for-profit sectors.
REFERENCES
1. Ferguson-Noel N. 2013. Mycoplasmosis. Introduction, p 875– 876. In Swayne DE, Glisson JR, McDougald LR, Nolan LK, Suarez DL, Nair V (ed), Diseases of poultry. Wiley-Blackwell, Ames, IA.
2. Weisburg WG, Tully JG, Rose DL, Petzel JP, Oyaizu H, Yang D, Mandelco L, Sechrest J, Lawrence TG, Van Etten J. 1989. A phylogenetic analysis of the mycoplasmas: basis for their classification. J Bacteriol 171: 6455– 6467.
3. Raviv Z, Ley DH. 2013. Mycoplasma gallisepticum infection, p 877– 893. In Swayne DE, Glisson JR, McDougald LR, Nolan LK, Suarez DL, Nair V (ed), Diseases of poultry. Wiley-Blackwell, Ames, IA.
4. Luginbuhl RE, Tourtellotte ME, Frazier MN. 1967. Mycoplasma gallisepticum— control by immunization. Ann N Y Acad Sci 143:234 –238. https:// doi.org/10.1111/j.1749-6632.1967.tb27662.x.
5. Adler HE, McMartin D, Shifrine M. 1960. Immunization against Mycoplasma infections of poultry. Am J Vet Res 21:482– 485.
6. Whithear KG, Soeripto, Harrigan KE, Ghiocas E. 1990. Immunogenicity of a temperature sensitive mutant Mycoplasma gallisepticum vaccine. Aust Vet J 67:168 –174. https://doi.org/10.1111/j.1751-0813.1990.tb07748.x.
7. Whithear KG, Soeripto, Harringan KE, Ghiocas E. 1990. Safety of temperature sensitive mutant Mycoplasma gallisepticum vaccine. Aust Vet J 67:159 –165. https://doi.org/10.1111/j.1751-0813.1990.tb07745.x.
8. Evans RD, Hafez YS. 1992. Evaluation of a Mycoplasma gallisepticum strain exhibiting reduced virulence for prevention and control of poultry mycoplasmosis. Avian Dis 36:197–201. https://doi.org/10.2307/1591490.
9. El Gazzar M, Laibinis VA, Ferguson-Noel N. 2011. Characterization of a ts-11-like Mycoplasma gallisepticum isolate from commercial broiler chickens. Avian Dis 55:569 –574. https://doi.org/10.1637/9689-021711 -Reg.1.
10. Abd-el-Motelib TY, Kleven SH. 1993. A comparative study of Mycoplasma gallisepticum vaccines in young chickens. Avian Dis 37:981–987. https:// doi.org/10.2307/1591903.
11. Kleven SH, Fan HH, Turner KS. 1998. Pen trial studies on the use of live vaccines to displace virulent Mycoplasma gallisepticum in chickens. Avian Dis 42:300 –306. https://doi.org/10.2307/1592480.
12. Throne Steinlage SJ, Ferguson N, Sander JE, Garcia M, Subramanian S, Leiting VA, Kleven SH. 2003. Isolation and characterization of a 6/85-like Mycoplasma gallisepticum from commercial laying hens. Avian Dis 47:499 –505. https://doi.org/10.1637/0005 -2086(2003)047[0499:IACOAL]2.0.CO;2.
13. Gharaibeh S, Laibinis V, Wooten R, Stabler L, Ferguson-Noel N. 2011. Molecular characterization of Mycoplasma gallisepticum isolates from Jordan. Avian Dis 55:212–216. https://doi.org/10.1637/9526-091510 -Reg.1.
14. Raviv Z, Callison SA, Ferguson-Noel N, Kleven SH. 2008. Strain differentiating real-time PCR for Mycoplasma gallisepticum live vaccine evaluation studies. Vet Microbiol 129:179 –187. https://doi.org/10.1016/ j.vetmic.2007.11.017.
15. Hong Y, Garcia M, Levisohn S, Savelkoul P, Leiting V, Lysnyansky I, Ley DH, Kleven SH. 2005. Differentiation of Mycoplasma gallisepticum strains using amplified fragment length polymorphism and other DNA-based typing methods. Avian Dis 49:43– 49. https://doi.org/10.1637/7254 -080504R.
16. Ghorashi SA, Noormohammadi AH, Markham PF. 2010. Differentiation of Mycoplasma gallisepticum strains using PCR and high-resolution melting curve analysis. Microbiology 156:1019 –1029. https://doi.org/10.1099/ mic.0.031351-0.
17. Ghorashi SA, Bradbury JM, Ferguson-Noel NM, Noormohammadi AH. 2013. Comparison of multiple genes and 16S-23S rRNA intergenic space region for their capacity in high resolution melt curve analysis to differentiate Mycoplasma gallisepticum vaccine strain ts-11 from field strains. Vet Microbiol 167:440 – 447. https://doi.org/10.1016/ j.vetmic.2013.09.032.
18. Shil PK, Kanci A, Browning GF, Markham PF. 2011. Development and immunogenicity of recombinant GapA() Mycoplasma gallisepticum vaccine strain ts-11 expressing infectious bronchitis virus-S1 glycoprotein and chicken interleukin-6. Vaccine 29:3197–3205. https://doi.org/ 10.1016/j.vaccine.2011.02.035.
19. Kleven SH, Khan MI, Yamamoto R. 1990. Fingerprinting of Mycoplasma gallisepticum strains isolated from multiple-age layers vaccinated with live F strain. Avian Dis 34:984 –990. https://doi.org/10.2307/1591393. 20. Rodriguez R, Kleven SH. 1980. Pathogenicity of two strains of Mycoplasma gallisepticum in broilers. Avian Dis 24:800 – 807. https://doi.org/ 10.2307/1589957.
21. Lin MY, Kleven SH. 1982. Pathogenicity of two strains of Mycoplasma gallisepticum in turkeys. Avian Dis 26:360 –364. https://doi.org/10.2307/ 1590106.
22. Lin MY, Kleven SH. 1984. Evaluation of attenuated strains of Mycoplasma gallisepticum as vaccines in young chickens. Avian Dis 28:88 –99. https:// doi.org/10.2307/1590131.
23. Ferguson NM, Hepp D, Sun S, Ikuta N, Levisohn S, Kleven SH, García M. 2005. Use of molecular diversity of Mycoplasma gallisepticum by genetargeted sequencing (GTS) and random amplified polymorphic DNA (RAPD) analysis for epidemiological studies. Microbiology 151: 1883–1893. https://doi.org/10.1099/mic.0.27642-0.
24. Razin S, Yogev D, Naot Y. 1998. Molecular biology and pathogenicity of mycoplasmas. Microbiol Mol Biol Rev 62:1094 –1156.
25. Fraser CM, Gocayne JD, White O, Adams MD, Clayton RA, Fleischmann RD, Bult CJ, Kerlavage AR, Sutton G, Kelley JM, Fritchman RD, Weidman JF, Small KV, Sandusky M, Fuhrmann J, Nguyen D, Utterback TR, Saudek DM, Phillips CA, Merrick JM, Tomb JF, Dougherty BA, Bott KF, Hu PC, Lucier TS, Peterson SN, Smith HO, Hutchison CA, III, Venter JC. 1995. The minimal gene complement of Mycoplasma genitalium. Science 270: 397– 403. https://doi.org/10.1126/science.270.5235.397.
26. Papazisi L, Gorton TS, Kutish G, Markham PF, Browning GF, Nguyen DK, Swartzell S, Madan A, Mahairas G, Geary SJ. 2003. The complete genome sequence of the avian pathogen Mycoplasma gallisepticum strain R(low). Microbiology 149:2307–2316. https://doi.org/10.1099/mic.0.26427-0.
27. Indikova I, Much P, Stipkovits L, Siebert-Gulle K, Szostak MP, Rosengarten R, Citti C. 2013. Role of the GapA and CrmA cytadhesins of Mycoplasma gallisepticum in promoting virulence and host colonization. Infect Immun 81:1618 –1624. https://doi.org/10.1128/IAI.00112-13.
28. Tulman ER, Liao X, Szczepanek SM, Ley DH, Kutish GF, Geary SJ. 2012. Extensive variation in surface lipoprotein gene content and genomic changes associated with virulence during evolution of a novel North American house finch epizootic strain of Mycoplasma gallisepticum. Microbiology 158:2073–2088. https://doi.org/10.1099/mic.0.058560-0.
29. Szczepanek SM, Tulman ER, Gorton TS, Liao X, Lu Z, Zinski J, Aziz F, Frasca S, Jr, Kutish GF, Geary SJ. 2010. Comparative genomic analyses of attenuated strains of Mycoplasma gallisepticum. Infect Immun 78: 1760 –1771. https://doi.org/10.1128/IAI.01172-09.
30. Hudson P, Gorton TS, Papazisi L, Cecchini K, Frasca S, Jr, Geary SJ. 2006. Identification of a virulence-associated determinant, dihydrolipoamide dehydrogenase (lpd), in Mycoplasma gallisepticum through in vivo screening of transposon mutants. Infect Immun 74:931–939. https:// doi.org/10.1128/IAI.74.2.931-939.2006.
31. Tseng CW, Kanci A, Citti C, Rosengarten R, Chiu CJ, Chen ZH, Geary SJ, Browning GF, Markham PF. 2013. MalF is essential for persistence of Mycoplasma gallisepticum in vivo. Microbiology 159:1459 –1470. https:// doi.org/10.1099/mic.0.067553-0.
32. Armour NK, Ferguson-Noel N. 2015. Evaluation of the egg transmission and pathogenicity of Mycoplasma gallisepticum isolates genotyped as ts-11. Avian Pathol 44:296 –304. https://doi.org/10.1080/ 03079457.2015.1044890.
33. Charlton BR, Bickford AA, Walker RL, Yamamoto R. 1999. Complementary randomly amplified polymorphic DNA (RAPD) analysis patterns and primer sets to differentiate Mycoplasma gallisepticum strains. J Vet Diagn Invest 11:158 –161. https://doi.org/10.1177/104063879901100209.
34. Shil PK, Kanci A, Browning GF, Marenda MS, Noormohammadi AH, Markham PF. 2011. GapA Mycoplasma gallisepticum ts-11 has improved vaccine characteristics. Microbiology 157:1740 –1749. https:// doi.org/10.1099/mic.0.046789-0.
35. Chikhi R, Medvedev P. 2014. Informed and automated k-mer size selection for genome assembly. Bioinformatics 30:31–37. https://doi.org/ 10.1093/bioinformatics/btt310.
36. Zerbino DR, Birney E. 2008. Velvet: algorithms for de novo short read assembly using de Bruijn graphs. Genome Res 18:821– 829. https:// doi.org/10.1101/gr.074492.107.
37. Darling AC, Mau B, Blattner FR, Perna NT. 2004. Mauve: multiple alignment of conserved genomic sequence with rearrangements. Genome Res 14:1394 –1403. https://doi.org/10.1101/gr.2289704.
38. Waterhouse AM, Procter JB, Martin DM, Clamp M, Barton GJ. 2009. Jalview version 2—a multiple sequence alignment editor and analysis workbench. Bioinformatics 25:1189 –1191. https://doi.org/10.1093/ bioinformatics/btp033.
39. Tamura K, Stecher G, Peterson D, Filipski A, Kumar S. 2013. MEGA6: Molecular Evolutionary Genetics Analysis version 6.0. Mol Biol Evol 30:2725–2729. https://doi.org/10.1093/molbev/mst197.
40. Wittwer CT, Fillmore GC, Hillyard DR. 1989. Automated polymerase chain reaction in capillary tubes with hot air. Nucleic Acids Res 17:4353– 4357. https://doi.org/10.1093/nar/17.11.4353.