I. INTRODUCTION
Commercial laying hens have been successfully selected for increasing production cycle lengths. Rather than being depopulated at 60 to 70 weeks of age, or being moulted to allow for additional production cycles, the egg industry has moved towards cycle lengths of 80 weeks or even longer. Selection for increased persistency of production and livability, skeletal health, and a slow rate of increase in egg size past peak production has resulted in commercial operations being able to achieve 500 eggs per hen at 100 weeks of age. Because of the increased lifetime output of calcium (Ca) by the hen, this extended period of production requires excellent management, including feeding the bird to optimize skeletal Ca reserves at the start of lay, and to minimize the loss of bone mass over time. The factors that contribute to skeletal health will also contribute to shell quality throughout the production cycle.
II. BONE BIOLOGY OF EGG-TYPE BIRDS
The skeleton of the pullet is comprised of two distinct structural bone tissues: cortical and trabecular. The cortical bone is the outer shell of bone tissue, whereas the trabecular bone tissue is the struts of bone that provide additional support, while minimizing bone weight as an adaptation for flight in birds. Skeletal growth of layer pullets proceeds in a similar manner as in other types of poultry and mammals with bones growing in both length and diameter. Prior to the estrogen surge associated with the onset of sexual maturity, a normal pullet with have a thick cortical shell, and well-developed trabecular struts (Figure 1A). In sexually mature hens that are laying eggs, a third type of bone (medullary bone) is formed in response to increasing estrogen levels. Medullary bone provides an additional, labile source of Ca to support eggshell formation that can be mobilized and re-deposited on a daily basis as Ca supply and demand ebb and flow with the formation of each egg.
With the onset of egg production, formation of structural bone ceases, and only medullary bone will be formed (Fleming et al., 1998b). In the weeks prior to the first egg, the bones increase in diameter (Whitehead, 2004) by approximately 20% as a means of increasing the volume into which medullary bone can be deposited. The rapid expansion of bone diameter involves both deposition of dietary Ca and P, as well as a redistribution of existing bone Ca and P mobilised from the endosteal surface (Fleming et al., 1998b; Whitehead, 2004), leaving pores in the cortical shell. As circulating estrogen levels increase in advance of sexual maturity, medullary bone is deposited as small spicules (Wilson and Thorp, 1998) lining the surface of the structural bone and also within the pores of the cortical shell (Whitehead, 2004) (Figure 1B). The deposition of medullary bone begins approximately 14 days before the first egg is laid (Hurwitz, 1964).
Medullary, but also potentially structural bone tissue is mobilized to augment the Ca coming directly from the diet in support of eggshell formation, particularly at night, when the amount of Ca coming directly from the digestive tract is limited. When the hen is not actively forming an eggshell, and dietary Ca and P supply exceeds the immediate demand, medullary, but not structural bone is replaced. Over time, the continual mobilisation and deposition of medullary bone leads to a more diffuse arrangement of medullary bone spicules throughout the marrow cavity (Figure 1C). Eventually, medullary bone can even fill the entire cavity of pneumatised bones (Fleming et al., 1998a). The reduction in the close association of medullary bone with the structural bone surfaces exposes the structural tissues to the action of osteoclasts. Since structural bone is not replaced as long as the hen is in lay, the cumulative effect of this erosion is a substantially decreased in structural bone mass (Figure 1C), and a greatly increased susceptibility to bone breaks (Whitehead and Fleming, 2000).
Thus, the problem of osteoporosis/caged layer fatigue in laying hens is not due to a loss of medullary bone, which then requires the hen to mobilize structural bone to support eggshell formation. Rather, it is a gradual erosion of structural bone, in spite of the presence of a large amount of medullary bone.
Figure 1 - Cross- sections of tibiae from laying hens of various ages. CB = cortical bone; TB = trabecular bone; MB = medullary bone. A) Layer pullet (16 weeks of age) containing only the cortical shell and trabecular struts. Diffuse staining within the cortical shell is an artifact. Structural bone (CB + TB) tissue shows very little in the way of pore formation at this level of magnification. Sexual immaturity was confirmed at time of sampling by the absence of ovary development. B) Laying hen after the first egg was laid, showing the cortical shell, trabecular struts, and medullary bone. The medullary bone is present as small spicules of bone tissue, initially deposited on the surfaces of the structural bone tissues. Pores containing medullary bone within the cortical shell are clearly visible. C) End of lay (67-week old) hen showing depletion of cortical and trabecular bone tissues, and diffuse nature of medullary bone throughout the medullary cavity. MB arrows point to some larger spicules of medullary bone.
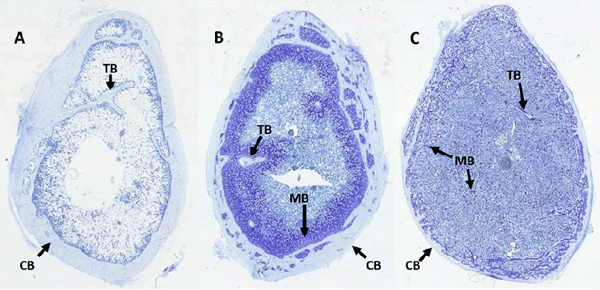
If the hen goes through a moult, oestrogen levels decrease (Braw-Tal et al., 2004), the medullary bone is resorbed, and structural bone reserves can be replenished (Whitehead and Fleming, 2000). The gradual loss of structural bone can increase the risk of osteoporosis, and the gradual increase in egg size (Zhang et al., 2019) and the ability to deposit only a constant amount of eggshell material (Roland Sr et al., 1975) after peak production can lead to decreasing shell thickness. These two factors represent important limitations to extended egg cycles, since production rates can remain high even at older ages (Bello and Korver, 2019).
Although genetic selection for skeletal health has allowed for greatly increased productivity, as well as increased skeletal health, the high demand for Ca to support eggshell formation means that Ca nutrition and skeletal health management are critical factors from the start of the pullet phase to the end of the production cycle.
III. PULLET MANAGEMENT FOR LONG-TERM BONE HEALTH
Because structural bone deposition ceases when the pullet approaches sexual maturity (Fleming et al., 1998b), great care and attention must be paid to pullet skeletal development. Once the hen commences to lay eggs, structural bone can only be depleted as long as she remains in production. Although medullary bone can be deposited and mobilized on a daily basis, and can even accumulate over the laying cycle, it makes only a small contribution to the strength of the skeleton (Fleming et al., 1998a).
The fundamentals of proper pullet management in general are consistent with the specific requirements for optimising skeletal development at the onset of lay. Maintaining appropriate body weight and condition for age, maintaining high flock uniformity, photostimulation at an appropriate physiological state (rather than a specific age) will not only support long-term hen productivity, but also skeletal quality. The importance of excellent pullet management becomes even greater when the long-term demands placed on the bird with longer laying cycles are considered. To support the longer laying cycles currently in use in the egg industry, the pullet skeleton should be managed such that the amount of structural bone is optimized before the point at which structural bone deposition ceases, and medullary bone formation begins. At that point, the objective is to maximize the amount of medullary bone within the skeletal system.
Birds that enter production at too light a body weight are not only susceptible to a post-peak dip in egg production because of limited energy reserves; such birds are also likely to have a small skeleton and limited medullary bone reserves. Frequent weighing of birds can ensure that reductions in growth rate relative to targets are identified and rectified quickly. More frequent feeding, use of higher nutrient density diets, or midnight feeding can be used to increase nutrient intake and therefore body weight gain. Midnight feeding of pullets close to the onset of lay may interfere with the regulation of sexual maturity, and induce pullets to come into production too soon (Leeson et al., 2003). This underscores the importance of maintaining an appropriate growth rate of pullets from the beginning, rather than responding with drastic measures to a large problem. To ensure the long-term health of the birds, it is important to delay photostimulation of an under-weight flock until an appropriate body size has been reached. Pre-lay diets can be an effective means of preparing the skeletal system, but should be used with caution. Since medullary bone is not deposited until 10 to 14 days before the first egg is laid, the higher Ca level of a pre-lay diet is of no advantage to the bird before this point. Although early work with layer pullets suggested that increasing dietary Ca level well before the 10 to 14 day period in advance of the first egg had no detrimental effect on the productivity and skeletal health of laying hens (Keshavarz, 1987), such work has not been repeated with modern genetics intended for very long production cycles. Maintaining a high degree of flock uniformity is important, particularly around the onset of lay. The increase in Ca demand to support egg production is the greatest of any nutrient as the bird progresses from immature to mature. This change happens suddenly. With a very uniform flock and appropriate timing of the change from a pullet developer or pre-lay diet to the start lay diet, a high proportion of the flock will have their needs for dietary Ca met. With a non-uniform flock, the first birds that enter lay may not be receiving sufficient Ca, whereas those entering later may be receiving too much Ca, which may alter Ca metabolism (Guo et al., 2008) and impair the ability to maintain shell quality and a healthy skeleton.
IV. LAYER MANAGEMENT FOR LONG-TERM BONE HEALTH AND EGGSHELL QUALITY
At the start of the egg production cycle, the hen has the maximum amount of structural bone that she will have, until she goes through a molt. In high-producing hens, the loss of structural bone over time is inevitable, but the key is to minimize the rate at which structural bone is lost. This involves maximizing the extent to which the medullary bone acts as a protective layer on structural bone surfaces, and reducing the need to mobilize bone Ca to support eggshell formation. Therefore, optimising the supply of dietary Ca is essential for long-term skeletal health and eggshell quality. With moderate reductions in dietary Ca from breeder-recommended levels, modern laying hens can maintain high levels of egg production and shell quality, with only minimal effects on skeletal Ca reserves. This indicates both a resistance to slight reductions in Ca and available P supply and an ability to utilize bone mineral reserves to support eggshell formation (Bello and Korver, 2019). Although modern laying hens are more resistant to caged layer fatigue than in the past, their high level of production over long laying cycles can predispose them to problems with skeletal and shell quality in the long term.
An egg contains approximately 10% eggshell by weight (Pelicia et al., 2009; Bello and Korver, 2019), meaning a 60 gram egg contains approximately 6 g of shell, comprised of approximately 95% CaCO3 (Nys et al., 2004), for a total of 2.3 g of Ca. This amount of Ca is obtained, in variable proportions, directly from the diet (absorbed from the gut and transported via the blood to the shell gland), or from the bone (bone mineral, both Ca and P, is resorbed by osteoclasts and the Ca transported to the blood to the shell gland. Approximately 60 to 75% of shell Ca is derived directly from the diet on shell-forming days (Driggers and Comar, 1949), and the greater the proportion coming directly from the diet, the greater the eggshell quality tends to be. Therefore, factors that increase shell quality will also reduce the need to mobilize bone Ca, and therefore will also tend to maintain skeletal health. On a daily basis, eggshell quality is negatively related to bone strength (Orban and Roland Sr, 1990). This relationship likely also impacts the long-term health of the skeleton, particularly since modern laying hens can maintain shell quality at the expense of bone mineralization (Bello and Korver, 2019). After peak production, the ability to deposit Ca onto the shell remains relatively constant (Roland Sr et al., 1975), meaning that increases in egg size after peak production will tend to result in reduced shell quality.
Dietary requirements for Ca tend to increase, and for phosphorus (P) tend to decrease as hens age. As hens age, the efficiency of Ca metabolism decreases (Wistedt et al., 2019), and increases in dietary Ca, and a widening of the Ca:available P ratio are intended to counteract this. Care must be taken not to over-feed Ca, which can reduce bone and eggshell quality (Akbari Moghaddam Kakhki et al., 2019), and interfere with exogenous phytase activity (Bello and Korver, 2019). Excess dietary P can also reduce shell quality (Miles et al., 1983). Because of its importance in Ca and P absorption from the gut, adequate dietary vitamin D activity must also be provided (Wen et al., 2019). Feeding of the vitamin D metabolite 25-OH vitamin D3 can help to maintain skeletal and shell quality in high-producing laying hens (Silva, 2017; Akbari Moghaddam Kakhki et al., 2019).
A hen will ovulate approximately 15 to 75 minutes following oviposition (Beuving and Vonder, 1981), and the ovum will take approximately 4.25 hours to reach the shell gland (Roberts, 2004), at which point calcification takes approximately 17 hours (Hincke et al., 2012). Since hens tend to lay eggs in the morning and the early part of the afternoon (Samiullah et al., 2016; Hunniford et al., 2017), the hen can use dietary Ca and P to replenish medullary bone stores for the first 5 hours after oviposition. When the ovum reaches the shell gland, Ca demand increases dramatically to support eggshell formation. The greatest rate of eggshell mineral accretion occurs from 5 to 15 hours after the egg enters the shell gland (Hincke et al., 2012), corresponding to the late afternoon and through the night before an egg is laid. If a hen is fed a diet containing only a small-particle Ca source such as finely ground limestone, the intestine will become devoid of a source of Ca during the night, when demand for Ca is highest. At that point, the hen will be entirely reliant on bone Ca to support eggshell formation. However, a large particle (2 to 4 mm), slowly dissolved source of dietary Ca will be retained in the gizzard for a long period of time, and Ca will be gradually released for absorption by the digestive tract, thus providing a direct dietary source of Ca throughout the night, and reducing the need for mobilization of bone Ca. A combination of 1/3 small particle, and 2/3 large particle Ca sources will provide readily available Ca to the hen when feeding begins at the start of the daytime (small particle), as well as a slowly-released source to support eggshell formation during the night (large particle).
Under conditions of heat stress, increased respiration rate can cause an increase in CO2 loss from the bird (Franco-Jimenez et al., 2007). The reduces the pool of bicarbonate ions, and causes respiratory alkalosis, an increase in blood pH (Franco-Jimenez et al., 2007). A reduction in bicarbonate ions in the shell gland reduces the formation of CaCO3, and decreases shell quality (Balnave et al., 1989).
Heat stress can also reduce feed intake, thereby reducing Ca intake and shell quality as a consequence. Midnight feeding is the addition of one to two hours of light in the middle of the dark period to all the birds to consume feed (van Staaveren et al., 2018). Birds will tend to decrease their feed intake during the day to reduce diet-induced thermogenesis, and will consume a proportion of their daily feed during the night, when temperatures are typically cooler. Thus, overall daily feed (and Ca) intake is increased. Midnight feeding can also have the benefit of providing a dietary source of Ca to support eggshell formation during the night, and reduce reliance on bone reserves (Harms et al., 1996). If the supplemental light is provided with a sufficient number of hours of dark before and after, the birds do not perceive the light in the middle of the night as the start of a new day, and regulation of egg production is not impaired.
Because the hen’s Ca and available P requirements, as well as the efficiency of absorption of these nutrients fluctuate throughout the day, some researchers have begun to investigate the concept of split feeding, in which the composition of diets offered to the hen is altered to reflect the changing nutrient requirements over the course of a day (Molnar et al., 2018). More work is needed to determine whether such an approach can be successful.
In acute situations of reduced shell quality because of feed mixing errors (deficiency in Ca), reduced feed intake due to high environmental temperatures, or other factors, it may be possible to provide additional Ca in the water, or by top-dressing feed with large particle Ca. Water supplementation of Ca can be effective (Damron and Flunker, 1995), but caution should be taken, as extended use can lead to lime deposits in water lines and valves, and hens may not be able to regulate their Ca intake. Top-dressing a large-particle Ca source is labour-intensive, but allows the hens ready access to Ca. As hens have an appetite for Ca, and can regulate intake based on need (Classen and Scott, 1982), it is unlikely that hens provided top-dressed feed with will over-consume Ca.
A number of other approaches have been used to manage skeletal and eggshell quality in laying hens. Copper is a component of lysyl oxidase (Chowdhury, 1990), manganese is part of glycosyl transferases ((Leach and Gross, 1983)), and zinc is found in carbonic anhydrase (Zhang et al., 2017). These trace minerals are involved in the calcification of the collagen matrix of the shell membrane, as well as the formation of the organic matrix of bone. Organic trace minerals can have greater bioavailability than inorganic sources, and increase shell quality (Stefanello et al., 2014). Dietary fat quality can also influence shell quality. Saturated fats in the diet can form insoluble soaps that are not well digested by poultry (Atteh and Leeson, 1983), although the effect may be greater in broilers than in layers (Atteh and Leeson, 1985).
High levels of salt intake reduce eggshell formation (Balnave et al., 1989). If the water provided to hens has a high level of sodium, the addition of salt to the diet should be reduced accordingly. There is evidence that at the same level of excess, sodium from water has a greater negative impact on shell quality compared to dietary sodium.
In addition to the move towards longer egg production cycles, the global move away from cage housing to more extensive housing systems (Leenstra et al., 2016) may also present additional challenges. Although the opportunity for increased exercise may increase bone strength overall (Silversides et al., 2012; Rodriguez-Navarro et al., 2018), the greater freedom of movement may increase the incidence of keel fractures due to collisions with structures such as perches (Wilkins et al., 2011). As well, the greater exposure of the hens to environmental dust and bacteria can increase systemic inflammation (Riddell et al., 1998; Nimmermark et al., 2009; Le Bouquin et al., 2013; Roque et al., 2015), which in turn could reduce bone (Tarlton et al., 2013) and eggshell quality (Nie et al., 2018).
Because structural bone formation does not take place after the onset of lay unless the bird goes through a moult, it is imperative that skeletal quality be maintained at as high a level as possible throughout the laying cycle. Conditions which result in excessive mobilisation of bone mineral to support eggshell formation can result in increased risk of broken bones over time. This not only results in lost productivity and reduced bird welfare, but also in irreparable damage to the skeleton, as long as the hen is in lay. The negative impacts can be managed, but cannot be reversed while the hens are producing eggs. Proper pullet development, the timing of photostimulation to ensure the birds have sufficient skeletal development, and using nutritional and management factors to reduce the use of bone Ca to support eggshell formation are key aspects of maintaining skeletal health and shell quality throughout long laying cycles.
Abstract presented at the 30th Annual Australian Poultry Science Symposium 2020. For information on the next edition, click here.