Introduction
Today, the broiler industry plays an important role in supplying the protein requirements of the people of the world. Shortening of broiler rearing cycle enhances the importance of growth during the first week of life (Nistan et al., 1991). Although genetic selection for growth has affected the digestive tract of poultry, the digestive processes are not fully developed on the day of hatch (Croom et al., 1999). Therefore, the insufficient nutrient digestion and absorption capacity of the intestinal tract of newly-hatched chicks may negatively affect their performance and survival (Croom et al., 1999). In addition, the final growth rate of broilers is directly proportional to the early growth rate of its digestive tract (Croom et al., 1999). Intestinal mucosal growth and production of digestive enzymes, such as alkaline phosphatase (ALP), γ- glutamyl transferase (GGT) and alanine aminopeptidase (AAP), are important for chick adaptation to the post-hatch period (Noy & Sklan, 1998). In the few days before and after hatch, intestinal weight increases more rapidly than body mass as a whole. The weight of the chicken intestine, relative to body weight, increases from approximately 1% on day 17 of embryonic age (Uni et al., 2003) to a maximum (10-20%) on days 4-8 post hatch (Noy & Sklan, 1998). Therefore, the first week after hatch may be the best period for studying the effects of exogenous factors on intestinal development in chickens.
Betaine is a methylated amino acid and plays a dual role as an osmolyte and as a methyl group donor in vertebrates. In the kidney, cells of the renal inner medulla accumulate organic osmolytes like betaine, sorbitol and inositol, to protect the cells against the osmotic load caused by urea and NaCl (Burg, 1995). Because the environment of the intestinal luminal content is hyperosmotic compared with the blood plasma, intestinal cells always need to cope with variable osmotic media (Morgin, 1976). Moreover, osmolytic protection mechanisms are necessary for nutrient digestion and absorption, since intestinal cells mediate the exchange of water and small solutes, such as ions, nutrients, and macromolecules between the blood plasma and the intestinal fluid. An in-vitro study with broiler chicks suggested that betaine acts as an intestinal osmolyte, at least in the duodenal epithelium, and affects the movement of water across the duodenal and ileal epithelium (Kettunen et al., 2001a). On the other hand, betaine supplementation in the diet of chickens suffering from coccidiosis significantly alleviated the harmful effects of the disease, as reported by Klasing et al. (2002), who showed that the reduction of villus height due to coccidia infection is recuperated after dietary betaine supplementation. According to Kettunen et al. (2001b), supplemental betaine reduces crypt/villus ratio in both coccidian-infected and healthy chicks, as well as lesion score in coccidian-infected chicks. In addition, betaine is involved in the protection of intestinal epithelium of broilers during periods of osmotic disturbance caused by water salinity stress, resulting in better growth rate and feed efficiency (Honarbakhsh et al., 2007).
It is well known that the microscopic structure of the small intestine mucosa and the activities of brush border enzymes are good indicators of the response of the intestinal tract to active substances present in feed and water (Viveros et al., 2011). Considering the effects of betaine on different aspects of intestinal development in one hand, and the importance of rapid intestinal growth during the first week post hatch in broiler chickens on the other hand, we decided to investigate the role of betaine on the intestinal development, including enzymatic indices and morphological changes, of broiler chickens during the first week of life.
Material and methods
All experimental procedures were approved and conducted by the recommendations of Animal Care Committee of Lorestan University (Khorramabad, Iran) with approval number LU.ECRA.2018.2.
Materials
Betaine (Betafin® 96%) was obtained from Biochem Company (Brinkstrasse 55, D-49393 Lohne, Germany). The ALP and GGT kits were supplied by Zeist Company, Tehran, Iran, and the alanine aminopeptidase (Chicken AAP) ELISA kit obtained from Elabscience Biotechnology co., Ltd, Wuhan, Hubei Province, P. R. China. All chemicals used were of analytical grade.
Birds, treatments, and management
A total of 50 one-day-old Ross 308 broiler chickens were purchased from Dorbar Poultry Company (Borujerd, Iran). The birds were individually weighed and randomly distributed into five treatment groups: control group (C, fed a basal diet formulated to meet the nutritional requirement of the birds according to the NRC (1994) recommendations; low-methionine group (LM, fed the basal diet which methionine content was reduced from 5.1 to 4.6 g/kg starter diet) (Sun et al., 2008); betaine 1 group (B1, control group diet + 500 mg betaine/L drinking water), betaine 2 group (B2, control group diet + 1000 mg betaine/L drinking water) (Attia et al., 2009); and low methionine plus betaine group (LM+B, low-methionine diet + 500 mg betaine/L drinking water). From 1 to 7 days of age, the birds of groups B1, B2, and LM+Breceived the respective betaine solutions by gavage once daily (Naidoo et al., 2008), at 2 mL on the first three days and 3mL on the next four days. In order to obtain the low methionine level for LM and LM+B groups, we decreased the amount of methionine premix in the diets manually.
The diets were based on corn-soybean meal (Table 1) and supplemented with a commercial vitamin and mineral premix and 5000 mg/kg choline chloride at 50%. The feeds contained no coccidiostats, antibiotics, or enzymes. Feed and water were provided ad libitum during the experimental period. The birds were subjected to a continuous lighting program of 23 L:1D, and the environmental temperature was maintained at 32°C.
The birds were housed in five separate floor pens on wood-shavings litter. Pens were located in the environmentally-controlled shed of the experimental farm of the Faculty of Agriculture, Lorestan University.
Each pen was equipped with a bell drinker and a pan feeder.
No signs of diseases or mortality were detected during the 7-d period. On day 7, birds were fasted for 3 h and then sacrificed by neck dislocation (Marchini et al., 2011). The small intestine was dissected, and the intestinal content was carefully flushed with saline solution. Duodenum, jejunum and ileum segments were collected for both biochemical and histological examinations. Tissues were fixed in 10% formalin buffer solution and submitted to the pathology lab of the Faculty of Veterinary Medicine, Lorestan University.
Sample preparation for Intestinal enzyme activity measurement
Sections of (2cm) the aforementioned intestinal segments were collected for the enzyme activity assays and stored at -70°C until analyses (within 2 months).
Samples were thawed and manually homogenized using liquid nitrogen in cold phosphate buffer saline (pH= 7, 0.1 M) containing 5mM EDTA, and the debris were removed by centrifugation at 5000 g for 10 min (Alirezaei et al., 2012). Supernatants were recovered and used for protein measurement and for the determination of alkaline phosphatase (ALP), γ-glutamyltransferase (GGT) and alanine aminopeptidase (AAP) activities.
Measurement of ALP and GGT Activities
Enzyme activities were assayed in homogenized tissues using commercial kits, according to manufacturer’s instruction. The activity of ALP was determined by spectrophotometrically measuring of the hydrolysis ofp-nitrophenol, as previously described (Palo et al., 1995). A unit of ALP activity is expressed as the amount of enzyme activity releasing 1 µmol of p-nitrophenol/hour. The activity of γ-glutamyltransferase (GGT) was also determined spectrophotometrically, as described by Rosalki et al. (1970), in micromoles of 5-amino-2-nitrobenzoate released per hour. Enzyme activities were expressed asmU/mg of tissue homogenates’ protein.
Measurement of AAP activity
The AAP activity was assayed by a sandwich-ELISA kit (Elabscience Biotechnology, China). This ELISA kit is used for in-vitro quantitative determination of chicken AAP concentrations in the serum, plasma and other biological fluids. Detection range was 0.31-20 ng/ mL and coefficient of variation was < 10%. Enzyme activity was calculated according to the manufacturer’s instructions and expressed ins ng/mg protein of tissue homogenate (ng/mg protein).
Small Intestine Morphometry
Segments of approximately 5 cm of the duodenum, jejunum, and ileum were cut and fixed in 10% buffered formalin solution, routinely processed for histology, and stained with hematoxylin and eosin (H & E) for morphometric analyses. Villus height (tip of villus to base of villus), crypt depth (base of villus to muscularis mucosa), and muscularis mucosa thickness were measured. Three slides of the duodenum, jejunum and ileum were prepared per bird. Villus height, crypt depth and muscularis mucosa thickness were measured in three points in each slide, totaling 21 points (Morovvati et al., 2017).
Statistical Analysis
All results are presented as mean± standard error of mean. Data were compared by one-way analysis of variance (ANOVA) with Tukey’s post-hoc analysis. A calculated P value of less than 0.05 was considered as statistically significant. Statistical analysis was performed using the IBM SPSS statistics version 22 (IBMcorp., USA). All variables were previously tested for normal distribution and homogeneous variances by Leven’s statistic test.
Results
Enzyme activity
The mean values (±SEM) of γ-glutamyltransferase (GGT), alkaline phosphatase (ALP) and alanine aminopeptidase (AAP) activities of the three segments of small intestine are presented in Figures 1 to 3, respectively. Duodenal and jejunal GGT activity was significantly higher in group B2 than in group LM (p< 0.05) (Figures 1a and 1b). Ileal GGT activity of group LM was statistically similar to that determined in the Betaine groups (Figure 1c). The activity of ALP in all three segments of the small intestine was significantly higher in the LMgroup compared with the other groups (p< 0.01) (Figure 2). Duodenal and ileal AAP activity was not statistically influenced by the treatments, but in the jejunum, AAP activity was significantly higher in the LM group compared with groups B1 and B2 (p< 0.001) (Figure 3). In addition, AAP activity in jejunum of the betaine groups was lower than in the C group (p< 0.001) (Figure 3b).
Small Intestine Morphometry
The administration or not betaine on day 7 posthatch had significant effects on gut morphometric parameters, as summarized in Tables 2-4.
The histological assessment of the duodenal sections showed significantly lower crypt depth (p< 0.05) after betaine administration in group B1 compared with the control group (Table 2). However, duodenal crypts were deeper (p< 0.05) in group C than in group LM (Table 2). No significant crypt depth differences were observed among groups B1, B2, LM+B and LM. Higher duodenal villi height/crypt depth ratio was determined in group B1 compared with group C. Duodenal muscularis mucosa was significantly thicker (p< 0.05) in group B2 compared to the other groups (Table 2), but not significantly different between groups LM and LM+B.
Jejunal villi were significantly higher in group B2 compared with group C (p< 0.05), whereas higher jejunal crypt depth values were determined in groups B2 and LM compared with groups LM+B and C (Table 3). Betaine administration to the LM group significantly increased (p< 0.05) jejunal villi height/crypt depth ratio (Table 3). It is noteworthy that groups B1, B2, and LM presented significantly thinner (p< 0.05) muscularis mucosa in the jejunum compared to groups C and LM+B (Table 3). Ileal villi height and crypt depth values were significantly higher (p< 0.05) in groups B2 and LM+B compared with groups C and LM (Table 4). Ileal crypts of B1 group were significantly shallower (p< 0.05) compared with group LM+B (Table 4). Significantly lower ileal villi height/crypt depth ratio (p< 0.05) was determined in group LM+B relative to group C (Table 4). The groups receiving betaine had significantly thicker (p< 0.05) ileal muscularis mucosa layer than groups C and LM, which presented the lower muscularis mucosa layer value (Table 4).
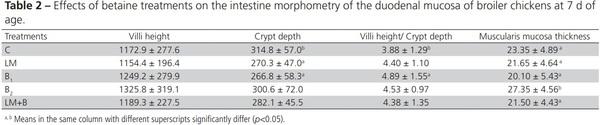


Discussion
Timely morphological adaptations of the enteric mucosa are required to accommodate functional brush border enzymes that mediate the digestion of carbohydrate- and protein-rich diets after hatch (Bohórquez et al., 2011). Noy & Sklan (1995) observed that, the secretions of pancreatic enzymes and bile per gram of feed intake were constant after 7 days posthatch, and that feed digestibility was uniform and high after this period. Therefore, brush border enzymes are critical during the first week of the chick’s life and factors having beneficial effects on the activity of those enzymes should be considered. To the best of our knowledge, the development of mucosal enzymatic activities has not been extensively examined in broiler chicks. During the migration of enterocytes from the crypt toward the villus tip, they acquire differentiated functions for digestion, including expression of enzymes such as disaccharidase and alkaline phosphatase (ALP) (Uni et al., 1998). The amount of enzymatic activity in the brush border of newly-hatched chicks is correlated with feed digestion (Noy & Sklan, 1998).
In the present study, γ-glutamyl transferase (GGT) activity was higher in duodenum and jejunum of B2 group compared to the LM group on day 7,and in the jejunum of LM+B group compared to the LM group (Figures 1a and 1b). This enzyme is involved in amino acid transport in the intestine (Uni et al., 1999). Temporal changes in mucosal enzyme activities are similar in chicks and turkey poults. In turkey poults, jejunal GGT activity increased from low levels at hatch, peaked at day 5, and reached a nadir at day 7, and tended to increase again by day 12. Other regions of the intestine show a constant increase in GGT activity (Uni et al., 1999).
The ALP activity was significantly higher in all three segments of LM group on day 7 (Figure2). A previous study (Uni et al., 1998) indicated that the ALP activity of chickens is higher in the duodenum and jejunum than the ileum on days 1 to 4 posthatch, and on day 7, it is relatively similar in all three segments of small intestine. Therefore, any influence of exogenous factors, e.g. methionine deficiency, is exerted along the entire small intestine. ALP is expressed in active and mature mucosal enterocytes and has been suggested as an enterocyte maturation marker (Traber et al., 1991). The proliferation rate of enterocytes rapidly changes, with a decrease in the proportion of proliferating villus cells to less than 10% in all intestinal segments within 48 h post hatch, indicating a rapid rate of maturation of enterocytes in chickens; however, in mammals, villus enterocytes are proliferating at birth, but enterocyte proliferation becomes restricted to the crypt after the third postnatal week (Hermos et al., 1971). Our results indicated that dietary methionine deficiency in broilers may cause enterocytes in all three segments of small intestine to be more mature than in broilers fed a conventional diet. Actually, methionine, as it is an essential amino acid, is required for enterocyte mitosis and intestinal development. Methionine deficiency would decrease crypt proliferation and may then limit the number of enterocytes available for villus growth, forming premature enterocytes, as shown by lower crypt depth in duodenum and ileum of low methionine group birds (Table 2).
Alanine amino peptidase (AAP) activity was higher in the jejunum, but not in the duodenum and the ileum of the LM group than in the groups receiving betaine (Figure 3). This is in agreement with the small intestine morphometry results, in which jejunal crypt depth in the LM group was higher than the LM+B and control groups. AAP is an intestinal brush border enzyme and plays a role in the final digestion of peptides generated from the hydrolysis of proteins by gastric and pancreatic proteases. According to the results of Uni et al. (1998), chicken’s brush border disaccharidase activities were significantly lower in the duodenum than in the jejunum. In line with the above-mentioned study, our results indicated a significant difference in the AAP activity in jejunum, but not duodenum. Cheled-Shoval et al. (2011) showed that MOS administration to chicken embryos (3 days before hatch) led to the early maturation of several brush border enzymes in the next few days after inoculation, as indicated by increases of 44% and 36% in alkaline phosphatase and sucrase isomaltase activities, respectively. Indeed, MOS caused higher maturation status in the enterocytes (CheledShoval et al., 2011). The early maturation of intestinal epithelium cells in the LM group of this study, as indicated by more activity of ALP and AAP or deeper crypts, is a remarkable finding.
It is assumed that increased villus height is paralleled by increased intestinal digestive and absorptive functions as a result of increased absorptive surface area, secretion of brush border enzymes, nutrient transport systems (Viveros et al., 2011), consequently increasing body weight gain (Zijlstra et al., 1996). Our results showed that betaine administration to broiler chickens fed a basal diet (B1 and B2 ) or a methionine-deficient diet (LM+B) increased jejunal and ileal villus height when compared with those fed the basal and the methionine-deficient diet (Tables 3 and 4). However, this effect was not seen in the duodenum. The reason might be that villus volume changed little in the first 2 d after hatch but increased rapidly in all segments thereafter. In the duodenum, this increase is limited to day7, whereas in the jejunum and ileum, villus size continues to increase up to day14 (Uni et al., 1998). In the study of Marchini et al. (2011), duodenal villus height increased by 82% until day 7 posthatch and 44% between days 7 and 14, while crypt depth increased by 73% until day 14 post hatch. Moreover, in broilers with delayed access to feed after hatch, duodenal villus volume after day 5 was close to that observed in the control birds, whereas in the jejunum, villus volume decrease was still evident after day11, whereas little effect of fasting on villus volume was noted in the ileum (Uni et al., 1998).
In the present study, jejunal and ileal crypt depth increased in B1 and B2 groups on 7 d (Table 2 and 3). Decreased crypt depth was observed in broiler chicks with delayed access to feed, and in those submitted to duodenectomy in the first week post-hatch as well as to heat stress (Uni et al., 1998, Song et al., 2013). However, duodenal crypt depth was decreased in group B1, but not in B2. This result is controversial and should be further studied using a higher number of birds before day 7 post hatch. In the LM group, lower crypt depth values were determined in duodenum and ileum compared with groups C and LM+B, respectively. Interestingly, significantly deeper crypts were obtained in the jejunum of the LM group than both in the C and LM+B groups (Table 3). According to Uni et al. (1998), crypt depth decreased and reached similar values as the controls on days 8 to 10 posthatch in the duodenum and jejunum and on day 5 in the ileum. It seems that day 7 posthatch may be too late to investigate the effect of betaine or methionine deficiency on crypt depth of different segments of the small intestine. Crypt development is crucial for intestinal development, because, as crypt size increases, the number of enterocytes and villus growth also increase, resulting in a larger area for intestinal absorption (Geyra et al., 2001).
There was a marked increase of villus-crypt ratio the duodenum of the B1 group compared with the control group, but not in the jejunum or the ileum (Table 2). In agreement with our data, this change has been reported in the jejunum of 21-d-old healthy or coccidiosis-affected broiler chickens previously fed betaine (Kettunen et al., 2001b). Betaine supplementation to LM group increased jejunal villuscrypt ratio (Table 3), while lower ileal villus-crypt ratio was obtained in the LM+B group compared to the control group (Table 4). Along the small intestine, the osmotic pressure in the duodenum is higher than in the ileum (Mongin, 1976). Accordingly, the highest level of betaine was found in the duodenum, whereas the betaine concentration in the ileum was very low (Kettunen et al., 2001a;Klasinget al., 2002). Therefore, the beneficial effect of betaine in the ileum may not be evident. Villus-crypt ratio has been used as an indicator of intestinal inflammation, as it is associated with increased epithelial proliferation (Kettunen et al., 2001b). In the small intestine, the proliferation increases the crypt proliferative compartment, while the absorptive villus compartment becomes smaller. The sensitivity of this ratio has been demonstrated when evaluating changes in dietary lipid composition (Galluser et al., 1993) and Zn deficiency (Livesey et al., 1985). It has been suggested that dietary betaine improves villus-crypt ratio in coccidia-challenged birds and also stabilizes the mucosal structure in birds with no apparent intestinal disease (Kettunen et al., 2001b). The significant increase in villus height and villus to crypt ratio of birds receiving the betaine generally indicates the ability of this supplement to accelerate the maturation of the broiler chicken’s gut. Early gut maturation is closely associated with an overall improvement of intestinal digestion and nutrient absorption, as villi play a crucial role in these processes (Chee et al., 2010).
During the early period of intense growth of broiler chickens, the transition from the yolk absorption by the embryo to the feed utilization by the chick is accompanied by many changes in developmental processes, particularly in the growth of the digestive organs (Nitsan et al., 1991). In particular, the intestinal epithelium undergoes profound anatomical adaptations during this period (Bohórquez et al., 2011). However, this phase has been generally neglected in poultry research, despite its association with body weight at marketing (Nitsan et al., 1991).
Muscularis mucosa thickness in the different segments of small intestine was affected by administration of betaine in different manners. Chickens in the B1 and B2 groups had thicker duodenal and ileal muscularis mucosa layer compared with the C group (Table 4). The addition of betaine to the low-methionine diet (LM+B group) also increased jejunal and ileal muscularis mucosa thickness (Tables 3 and 4). In the study of Viveros et al. (2011), the dietary inclusion of grape-derived concentrates and avoparcin increased jejunal muscularis mucosa of 21-d-old broilers. The intestinal muscle thickness of the jejunum of day-old chicks injected in ovo with a mannan oligosaccharide (MOS) was significantly higher compared with the control group (Cheled-Shoval et al., 2011). On the other hand, a previous study (Chee et al., 2010) showed that neither MOS nor bacitracin influenced the thickness of the ileal muscularis mucosa layer, crypt depth or villus height to crypt ratio. In the present study, jejunal muscularis mucosa thickness decreased in the B1 and B2 groups compared with the C and LM+B groups (Table 3). That is in agreement with Miles et al. (2006), who reported that the inclusion of antibiotics in broiler diets decreased the thickness of muscularis walls. Thicker muscularis layer and higher villi along the small intestine are related with the maturity of the intestinal mucosa (Cheled-Shoval et al., 2011). In addition, the intestinal mucosa layer, which includes the epithelium, lamina propria and muscularis mucosa, acts as the first major barrier against pathogenic bacteria in the lumen and plays an important role in the protective scheme against bacterial adherence (Chee et al., 2010).
In conclusion, betaine is a promising agent for the acceleration of the intestinal development of broiler chickens fed low-methionine or standard diets. It seems that betaine enhances intestinal development by promoting both mucosal growth and digestive brush border enzyme activities. However, further studies, using a larger broiler sample size, should be performed to evaluate the detailed effects of betaine.
This article was originally published in Revista Brasileira de Ciência Avícola, Jul - Sept 2018 / v.20 / n.3 / 403-412. http://dx.doi.org/10.1590/1806-9061-2017-0468. This is an Open Access article under a Creative Commons Attribution License.