Importance of Methionine
Nutritionists agree that livestock diets require supplementation with some essential amino acids to increase the efficiency of protein utilization and to reduce nitrogen excretion. Methionine is an essential amino acid and is necessary for growth. Numerous trials have shown that providing adequate levels of methionine are required to maximize performance of poultry and pigs. Additionally, methionine plays biological roles beyond protein synthesis, through conversion into other sulphur-containing metabolites such as glutathione and taurine, two major cellular non-enzymatic antioxidants, and as a donor of methyl-groups. Therefore, underestimating the importance of methionine in monogastric nutrition has an important economic impact.
To meet the methionine requirements of livestock, diets are supplemented with a synthetic source of methionine. There are currently three sources of methionine available for animal nutrition: L methionine (L-Met), DL-methionine (DL-Met) and DL-OH-Methionine (DL-OH-Met). Animals can only use L Met for protein synthesis and the other sources are precursors and need to be converted by the animal to L Met. Methionine sources are valued in feed formulation not only for the supply of sulphur amino acids (methionine and cysteine), but also for the energy supply. Yet, the energy values of synthetic methionine sources need to be clarified. This paper aims to determine the energy values of synthetic methionine sources based on their respective metabolic pathways. The study also calculates the energy cost of the different conversion steps leading to L-Met from D-Met and DL-OH-Met.
The energy values of L-Methionine
Crystalline L-Met, like other amino synthetic amino acids, is supposed to be 100% digestible and the digestible energy (DE) value is therefore equal to the gross energy (GE) value. If dietary L-Met is used for body protein deposition, its metabolizable energy (ME) value equals the DE and GE value. However, if (part of) L-Met is deaminated, part of the energy of L-Met will be lost as urea or as uric acid. Only the energy retained in the carbon chain can then be used for other purposes. In the INRA tables (Sauvant et al. 2004), it is assumed that pigs retain 50% of the dietary protein in body protein and that the other 50% is excreted in the urine. The value of 50% retention is probably a conservative estimate for L-Met (as a free amino acid) because it is included to reduce the crude protein content of the diet and to increase the efficiency of nitrogen utilization. L-Met has a GE value of 3522 kJ/mol (23.6 kJ/g), while the energy values for urea (2 N-atoms) and uric acid (4 N-atoms) are respectively 635 and 1926 kJ/mol. The ME value of L-Met can thus be calculated as:
Mammals: ME = p · 3522 + (1-p) · (3522 – 635/2)
Birds: ME = p · 3522 + (1-p) · (3522 – 1926/4)
Where p is the fraction of L-Met retained as body methionine. With p=0.5, the ME value of L-Met in birds is 3281kJ/mol, which is 7% lower compared to the value when all dietary L-Met is retained as body methionine.
The calculation of the net energy (NE) value of L-Met is more complex. If L-Met is retained as methionine in body protein, one could think that all of the energy of methionine is retained as well. Although this is true, it requires energy to retain methionine in body protein, because the synthesis of a peptide bond requires at least 4 ATP. Moreover, due to protein turnover, peptide bonds are hydrolysed and resynthesized repeatedly. The hydrolysis of a peptide bond also requires at least 1 ATP. Table 1 shows the energy cost to retain 1 mole of methionine.
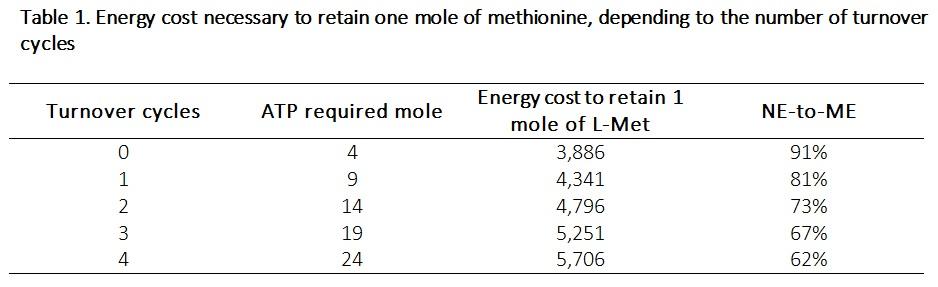
The turnover of body protein varies greatly among tissues (e.g., it ranges from 4% per day in muscle to more than 100% per day in intestinal tissues). It is difficult to provide a theoretical estimate of the energy cost of amino acid turnover. When L-Met is deposited in muscle early on in life, it is likely that it underwent 6 turnover cycles by the time the animal is slaughtered 150 days later (0.04 x 150 days). It is quite similar in poultry where the muscle turnover is faster (20% per day) but for a shorter duration (35 days) resulting in 0.20 × 35 = 7 cycles. On the other hand, an amino acid given a few days before slaughter may only require the ATP cost of peptide synthesis to be retained in muscle protein.
Empirical NE estimates can be used to calculate the NE-to-ME ratio of L-Met. For example, it is often assumed that the NE-to-ME ratio of dietary protein is approximately 60%. Based on the amino acid composition of the whole-body protein, this would correspond to an average of three turnover cycles (i.e., from weaning to slaughter and across tissues). This would mean that the NE-to-ME ratio would be 67% (Table 1). This value is greater than that of whole-body protein because L-Met is a relatively large amino acid.
Once deaminated, the carbon-chain of methionine can be used for different purposes, such as the synthesis of non-essential amino acids, lipid, or ATP. Experimental evidence from van Milgen et al. (2001) indicated that the NE-to-ME ratio was remarkably similar for a well-balanced protein source (casein, most of which is retained as body protein) compared to an unbalanced protein source (zeïn, most of which will be deaminated and used for other purposes). Although this result was coincidental, it allows us to hypothesize that the NE-to-ME ratio of protein and amino acids is not affected by the ultimate fate of the protein and amino acids. Energy values of L-Met assuming that 75% of L-Met is retained as body methionine are presented Table 2.
Calculation of the energy values of synthetic methionine sources
DL-Methionine contains 50% of D-Met and 50% of L-Met whereas OH-Met contains 50% D OH-Met and 50% L- OH-Met. The first step from methionine precursors to L-Met is the conversion to the common intermediate 2?keto-4-methylthio-butanoic acid (KMB). The conversion involves enzymes (oxidases and dehydrogenases), cofactors and release compounds, which differ depending on the precursor considered.
Crystalline amino acids are considered to be absorbed at 100% so that no faecal energy is lost and GE = DE. The ME value is linked to the urinary and gas energy losses. It is assumed that these energy losses are only linked to nitrogen excretion of the amino group (-NH2) from amino acids (Figure 1a). Accordingly, the ME-to-GE ratio is specific for the considered species and depends on the energy cost linked to urea or uric acid excretion in respectively mammals and birds.
The NE-to-ME ratio accounts for energy losses related to the metabolic costs of the conversion. Therefore, the calculation of the NE values considers the energy lost or spared in the conversion of the enantiomers of both precursors of L-Met (D-Met; D and L-OH-Met). This calculation is based on the ATP used or yielded from the direct or side reactions. To translate this into an energy value, we considered that one glucose molecule (2,820 kJ/mol) yields 31 ATP. Therefore, 91 kJ of glucose is required to synthesize 1 ATP. Accordingly, the calculation of energy losses is based on the equivalency of 91 kJ per ATP. This work was based on the metabolic model described by Van Milgen (2002).
To simplify:
- The energy resulting from nitrogen excretion as urea or uric acid allows to calculate the difference between ME from GE.
- The ATP used or yielded during the conversion of the precursors to L-Met allows to calculate NE from ME from precursor to L-Met and then the NE-to-ME ratio used for L-Met is applied.
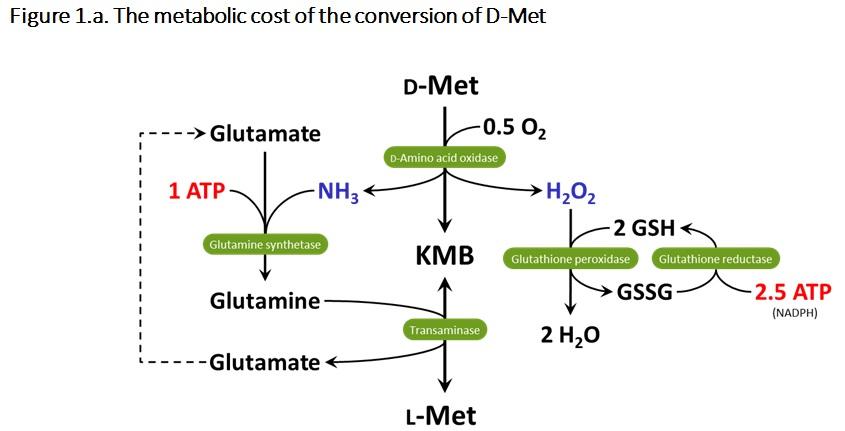
Metabolizable energy values of L-Methionine precursors
The conversion of D-Met to KMB releases an amino group, which is recovered after transamination of KMB to L-Met and has therefore no effect on the nitrogen balance (Figure 1a). Therefore, the ME values of D-Met and L-Met are the same.
D- and L-OH-Met do not have an amino group and they need this amino group from another amino acid (given in excess of protein deposition) to transaminate KMB into L-Met (Figure 1b). This means that OH-Met has a nitrogen-sparing effect, because the nitrogen otherwise excreted as urea or uric acid is now used for L-Met synthesis. This nitrogen-sparing effect is associated with a corresponding energy-sparing effect. The ME-to-GE can therefore be calculated as the output (energy in L-Met) divided by the energy input (energy in D- and L-OH-Met plus the energy not excreted as urea or uric acid). These ME-to-GE values are 109% for pigs and 114% for birds, relative of the ME value of L- Met.
Net energy values of L-Methionine precursors
To calculate the NE values, we refer to the Figures 1a and 1b describing the conversion steps of methionine precursors and the cost or gain of the metabolic side pathways. D-Met and L-OH-Met are converted to KMB by an oxidase and yield a molecule of hydrogen peroxide (H2O2) as “by-product”. This molecule needs to be catabolised through the oxidation of reduced glutathione (GSH) to oxidised glutathione (GSSG). This reaction requires 1 NADPH, which is equivalent to 2.5 ATP. Moreover, the conversion of D-Met to KMB results in the release of an amino group. Glutamate can be used as a receptor for this amino group, but it requires one additional ATP to synthesize glutamine from glutamate and the released amino group. On the other hand, D-OH-Met is converted to KMB through a dehydrogenase, which leads to the formation of 1 NADH that is oxidized in the mitochondria to produce 2.5 ATP/mol (van Milgen, 2002).
From these results, the theoretical energy costs of converting D-Met, D-OH-Met, and L-OH-Met to L-Met were calculated (van Milgen et al. 2019). The conversion of DL-Met to L-Met costs 1.75 ATP (-3.5 ATP for D-Met and 0 ATP for L-Met) while there is no net cost for the conversion of DL-OH-Met to L-Met ( 2.5 ATP for L-OH-Met and +2.5 ATP for D-OH-Met).
Summary
The ME-to-GE and NE-to-ME ratios of DL-Met and DL-OH-Met, relatively to L-Met are summarized in Table 3.
Overall, by combining the two sources of information (Table 2 and 3), we can estimate the ME and NE values of the different sources of methionine that could be used for feed formulation (Table 4).
Conclusion
The energy value for amino acids is based on assumption of the retained proportion of the given amino acid for ME calculation and the energetical costs for their incorporation as protein and the number of synthesis/proteolysis cycles for the given amino acid. In case of L-methionine precursors, the conversion cost must be taken under consideration. It is also interesting to note that the NE-to-ME ratio for an amino acid seems to be independent of its metabolic fate of carbon chain when deaminated.
These calculations showed that, although more metabolic conversions are needed for OH-Met to L-Met, it does not result in an extra energy cost compared to other methionine sources based on the metabolic pathways. On the contrary, due to the nitrogen-sparing effect of OH-Met, its energy value is even higher than that of the other methionine sources when expressed as ME. From a practical point of view, supplemented methionine contributes little to the energy value of the diet and differences between methionine sources will not have a large impact for feed formulation. Considering the dietary incorporation rates of synthetic Met sources (around 3 kg/ton) and given the metabolizable energy values of these molecules (in average 5356 kcal/kg), their contribution counts for 16.1 kcal/ton of feed.