Introduction
The influence of protein and energy on growth performance and carcass traits in broiler chickens have been intensively studied in the literature and the investigation is ongoing because the performance of modern broiler chickens improves every couple of years as a result of successfully selective breeding program. However, starch and lipid are the two major source of energy in typical broiler diets and little information about the influence of starch and lipid on bird performance and nutrient utilisation has gained in the literature. Starch is the most abundant macronutrient in broiler diets and its primary function is to provide energy for maintenance and growth and escalating dietary starch concentrations would increase lipid concentrations in carcass. Dietary lipid for poultry, including poultry fat and vegetable source oil, has higher energy density but is more expensive than starch. Its concentration is also critical to pellet quality and feed intake. Liu et al. [1] reported a significant 8.8% reduction in feed intake in birds offered diets containing higher lipid concentrations and such responses are often seen in practice. This could be due to dietary lipid negatively impacting on pellet quality [2], and/or delaying gastric emptying [3]. In the present study, the influence of dietary starch to lipid ratios on growth performance, carcass compositions and nutrient utilization was investigated. Amino acids and energy are considered in tandem in the formulation of practical diets for monogastric animals [4] and Lewis [5] suggested grams per unit of energy may be the best method of expressing amino acid requirements when animals are given ad libitum access of feed. Indeed, poultry nutritionists may choose to fix the ratio of protein and energy in practical least-cost feed formulation in order to conveniently change dietary nutrient densities according to the fluctuation of feed ingredient prices. This approach is suitable when broiler chickens tend to eat to a constant energy intake regardless of the energy density of the diet [5]; however, it remains debatable whether modern broilers eat to constant energy intake [6, 7]. Liu et al. [1] reported quadratic relationship between protein to energy ratios and growth performance in broiler chickens. Similarly, Gous et al. [7] reported broken-stick response to protein to energy ratios and efficiency of protein utilisation. Therefore, in order to avoid introducing confounding factors into the present study, all the experimental diets were formulated to consistent ratios of digestible lysine to apparent metabolisable energy (0.87 g digestible lysine/MJ nitrogen–corrected apparent metabolisable energy); however, digestible lysine concentrations were ranged from 9.5 to 12.1 g/kg. The hypothesis was that increasing nutrient density would enhance feed conversion efficiency and the starch to lipid ratio would influence the carcass composition of broiler chickens.
Materials and methods
The present study is one of a series to investigate the influence of macronutrients on feed intake, weight gain and carcass composition in broiler chickens. The details of bird management, diet preparation and sample collection were similar to methodology described in Liu et al. [1].
Diets preparation
The feeding study comprised twelve dietary treatments with three energy density levels (11.25, 12.38 and 13.50 MJ/kg) and four starch to lipid ratios (14, 12, 7, 4) as shown in Table 1. All diets were formulated to contain similar digestible lysine to metabolisable energy (0.87 g digestible lysine/MJ nitrogen–corrected apparent metabolisable energy) and ideal amino acid ratios. The analysed dietary starch concentrations ranged from 276 to 414 g/kg and lipid ranged from 26 to 76 g/kg. There were variations between formulated and analysed macronutrient concentrations as shown in Table 1. The composition and calculated nutrient specifications in experimental diets 1–4 and diets 9–12 for broiler chickens are shown in Table 2. Diets 5–8 contained intermediate levels as they were derived from 50–50 blends of the corresponding low and high nutrient density diets.
Maize was hammer-milled through 6.0 mm screen prior to mixing with the other ingredients and diets were cold-pelleted and then crumbled. Acid insoluble ash (CeliteTM World Minerals, Lompoc, CA, USA) was included in the diets at 20 g/kg as an inert marker to determine nutrient digestibility coefficients at the distal jejunum and distal ileum at 27 days post-hatch. Starch concentration were determined by a procedure based on dimethyl sulfoxide, α-amylase and amyloglucosidase, as described in Mahasukhonthachat et al. [8]. Nitrogen concentrations were determined as outlined by Siriwan et al. [9]. Lipid concentration was determined by using the automated Soxhlet extraction as described in Luque de Castro and Priego-Capote [10].
Bird management
This feeding study was approved by the Animal Ethics Committee of the University of Sydney (Project No. 601). Male, one day-old chicks (Ross 308) were received from a commercial hatchery and were offered a commercial starter diet to 7 days post-hatch. They were then individually identified (wing-tags), weighed and allocated into bioassay cages, with dimensions of 750 mm in width, 750 mm in length and 510 mm in height, on the basis of body weight in an environmentally-controlled facility. There was no statistical difference on the average body weight for each cage at the beginning of the feeding study. Each of the dietary treatment was offered to four replicate cages (6 birds per cage) or a total of 288 chicks from 7 to 27 days post hatch. Broilers had unlimited access to water and feed under a ‘23-hour-on-1-hour-off’ lighting regime for the first three days and then under a ‘16-hour-on-8-hour-off’ lighting regime for the remainder of the study. Room temperature was maintained at 32°C for the first week, then gradually decreased to 22 ± 1°C by the end of the third week and maintained at the same temperature until the end of the feeding study. Body weight and feed intake were recorded weekly from which feed conversion ratios (FCR) were calculated. The incidence of dead or culled birds was recorded daily and their body-weight was used to adjust FCR calculations.
Axtra PHY TPT was included at the rate of 1000 FTU/kg 2 The vitamin-mineral premix supplied per tonne of feed: [MIU] retinol 12, cholecalciferol 5, [g] tocopherol 50, menadione 3, thiamine 3, riboflavin 9, pyridoxine 5, cobalamin 0.025, niacin 50, pantothenate 18, folate 2, biotin 0.2, copper 20, iron 40, manganese 110, cobalt 0.25, iodine 1, molybdenum 2, zinc 90, selenium 0.3 3 Digestible amino acids.
Sample collection and chemical analysis
Total excreta were collected from 24–26 days post-hatch from each cage to determine parameters of nutrient utilisation which included apparent metabolisable energy (AME), metabolisable energy to gross energy (GE) ratios (AME:GE), nitrogen retention and N-corrected apparent metabolisable energy (AMEn). Excreta were air-forced oven dried for 24 h at 80°C. The GE of diets and excreta were determined by bomb calorimetry using an adiabatic calorimeter (Parr 1281 bomb calorimeter, Parr Instruments Co., Moline, IL).
The jejunum is reported to be the major site of glucose and amino acid absorption but the extent of nutrient digestion at the end of ileum, which is often expressed as apparent ileal digestibility coefficient, is usually reported in the literature [11, 12]. Apparent digestibility coefficients of starch and protein were determined in both distal jejunum and distal ileum in the present study. At day 28, all birds were euthanized by intravenous injection of sodium pentobarbitone and the small intestine was removed and digesta samples were collected in their entirety from the distal jejunum and distal ileum. The jejunum was demarcated by the end of the duodenal loop and Meckel’s diverticulum and the ileum by Meckel’s diverticulum and the ileo-caecal junction. Digesta was taken from the segment posterior to the respective mid-points. Digesta samples from birds within a cage were pooled, homogenized, freeze-dried and ground through 0.5mm screen. Then, the samples were analysed for the content of starch and protein as previously described.
Two birds from each cage whose body weight was close to the cage mean were selected for carcass composition analyses. The carcass was weighed with feathers but without viscera to calculate carcass yield before processing. Then, the carcass was autoclaved, ground and freeze-dried to analyse for nitrogen, lipid, DM concentrations and GE as described previously.
Calculations
The AME values were calculated on a DM basis from the following equation:
AME:GE Ratios were calculated by dividing AME by the GE of the appropriate diets. N contents of diets and excreta were determined using a nitrogen determinator (Leco Corporation, St Joseph, MI) and N retentions calculated from the following equation
N-corrected AME (AMEn MJ/kg DM basis) values were calculated by correcting N retention to zero using the factor of 36.54 kJ/g N retained in the body [13]. Apparent metabolisable energy intakes (MJ/day DM) were calculated from dietary energy densities and average daily feed intakes over the entire feeding period.
Acid insoluble ash (AIA) was included in the diets at 20 g/kg as an inert marker. Apparent digestibility coefficients of starch and protein (N) were calculated by the following equation:
Starch and protein (N) disappearance rates (g/bird/day) were deduced from feed intakes over the final phase of the feeding period from the following equation:
Statistical analysis
Experimental data were analysed using JMP 9.0.0 (SAS Institute Inc. JMP Software. Cary, NC) and response surfaces were generated with R 3.1.3 software. The experimental units were replicate cage means and statistical procedures included analyses of variance using the general linear models and a probability level of less than 5% was considered to be statistically significant by Student’s t-test. Additionally, the response surface plots were constructed so that the effects from changing factor levels on the examined responses can be visualized and they were generated by generalized additive model with thin plate regression splines as the smoothing function, the details of analysis and interpretation of the contour plots can be reviewed in SolonBiet et al. [14]. Linear and quadratic regressions were also considered in statistical analyses of the experimental data. Pairwise correlation was conducted to explore the relationships between apparent digestibility coefficients, nutrient utilisations, growth performance and carcass traits and a probability level of less than 5% was considered to be statistically significant.
Results The mortality rate during the experimental period was 1.7% and it was not related to dietary treatments (P > 0.30). The average weight gain and FCR for all the experimental treatments from 7 to 27 days post-hatch was 1591 g/bird and 1.407, respectively, which were clearly superior to the 2014 Ross 308 performance objectives of 1292 g/bird weight gain with and FCR of 1.463. The influence of nutrient densities and starch to lipid ratios on growth performance and carcass traits from 7–27 days post-hatch is shown in Table 3 (S1 Table). There were no interactions between energy densities and starch to lipid ratios on growth performance and carcass weight and yield (P > 0.05). Feed intake was reduced by 6.4% with increased energy densities (2314 versus 2167 g/bird, P < 0.001) and weight gain and FCR were improved with increased dietary energy densities by 10.5% (1511 versus 1670 g/bird) and 15.3% (1.533 versus 1.298), respectively (P < 0.001). There were significant linear relationships between starch to lipid ratios with weight gain (r = 0.448, P = 0.001) and feed intake (r = 0.509, P < 0.001).
. Broiler chickens offered diets with the lowest starch to lipid ratio had significantly inferior weight gain (1498 g/bird), feed intake (2098 g/bird) and carcass weight (1505 g/bird) in comparison to birds offered diets containing the other three levels of starch to lipid ratios. In contrast, FCR was not influenced by dietary starch to lipid ratios (P = 0.794). Both nutrient densities and starch to lipid ratios had significant impacts on carcass weight and yield. Carcass weight (r = 0.441, P = 0.002) and yield (r = 0.277, P = 0.057) decreased with reduced starch to lipid ratios. Broiler chickens offered diets with the highest starch to lipid ratio had significantly higher carcass yield compared to the other treatment groups (P = 0.001). There were treatment interactions on carcass GE (P = 0.002). In low density diets, broiler chickens offered starch: lipid ratio of 14 had significantly higher carcass GE than birds offered diets containing the other three starch: lipid ratios. Differently, in medium density diets, starch: lipid ratios did not influence carcass GE. In high density diets, broiler chickens offered starch: lipid ratio of 4 had significantly lower carcass GE than birds offered diets containing the other three starch: lipid ratios. There were no dietary effects on carcass protein concentrations (P > 0.50) and DM concentrations (P > 0.15). There were interactions on carcass lipid concentrations (P < 0.001).
Broiler chickens offered diet containing the lowest nutrient density and highest starch to lipid ratio had the most carcass lipid (12.94%) whereas broiler chickens offered diet containing the highest nutrient density and lowest starch to lipid ratio had the least carcass lipid (8.25%).
The influence of energy densities and starch to lipid ratios on distal jejunal and distal ileal apparent digestibility coefficients and disappearance rates of starch and protein are shown in Table 4 (S1 Table). Escalating energy densities increased protein (N) digestibilities in the distal jejunum (P <0.001) and distal ileum (P < 0.0001). Broiler chickens offered high density diets had significantly higher apparent protein digestibility coefficients in the distal jejunum and distal ileum than broiler chickens offered low and medium density diets. Boiler chickens offered diets containing starch: lipid ratio of 4 had significantly lower apparent protein digestibility coefficient in the distal ileum (P = 0.004) in comparison to birds offered diets with all the other three ratios. Dietary nutrient density did not influence apparent starch digestibility coefficients in the distal jejunum (P = 0.497) and distal ileum (P = 0.639). Starch to lipid ratios significantly influenced apparent starch digestibility coefficient in the distal jejunum (P = 0.023) and the only significant difference was observed between birds offered diets with starch: lipid ratio of 14 and 12 (0.967 versus 0.934). There was an interaction between nutrient densities and starch to lipid ratios on apparent digestibility coefficients of starch in the distal ileum (P < 0.001). In low and medium density diets, broiler chickens offered diets containing starch: lipid ratio of 7 had significantly lower apparent starch digestibility coefficient in the distal ileum in comparison to birds offered diets with all the other three ratios. However, in high density diets, broiler chickens offered diets containing starch: lipid ratio of 4 had significantly lower apparent ileal starch digestibility coefficient compared to birds offered diets with all the other three ratios.
Disappearance rates of starch and protein in the distal jejunum and distal ileum were influenced by nutrient densities (P < 0.001) and starch to lipid ratios (P < 0.02). Generally, disappearance rates of starch and protein were improved with increased nutrient densities regardless of the site of small intestine. There were positive correlations between starch to lipid ratios and protein (N) disappearance rate in the distal jejunum (r = 0.306, P = 0.034) and distal ileum (r = 0.580, P < 0.0001); starch disappearance rate in the distal jejunum (r = 0.354, P = 0.015) and distal ileum (r = 0.542, P < 0.0001).
The influence of nutrient densities and starch to lipid ratios on nutrient utilisation in broiler chickens is shown in Table 5 (S1 Table). There were treatment interactions between nutrient densities and starch to lipid ratios for AME, AME:GE ratios and AMEn (P < 0.005). Starch: lipid ratios did not influence AME in broiler chickens offered low density diets; whereas, in high density diets, birds offered starch: lipid ratios of 4 and 7 significantly higher AME than broiler chickens offered diets with the other two ratios. Broiler chickens offered the lowest nutrient density diet and the lowest starch to lipid ratio had the lowest AME (13.11 MJ/ kg, P < 0.0001) and AMEn (12.28 MJ/kg, P < 0.0001). N retention reduced with starch to lipid ratios (r = 0.474, P = 0.001) and the lowest N retention (68.2%) was observed when the starch to lipid ratio was equal to 4 (P = 0.012). Increasing energy densities from 11.25 to 13.50 MJ/kg significantly reduced water intake by 14.6% (378 versus 323 ml/day/bird, P = 0.004).
Discussions
Macronutrients and feed intake
The response surface plots were constructed so that the effects from changing factor levels on the examined responses can be visualized and they were generated by generalized additive model with thin plate regression splines as the smoothing function. The influence of analysed dietary starch, protein and lipid concentrations on feed intake in broiler chickens from 7–27 days post-hatch is shown in Fig 1. Both lipid and protein are more influential on feed intake in poultry than dietary starch concentrations [1, 15]. When dietary lipid and starch concentrations were compared, contours were almost parallel to the x-axis and this indicates that dietary lipid concentration had a greater impact on feed intake than starch and feed intake was reduced with increased lipid concentrations. This observation is consistent with previous findings [1, 15]. Response surface graphs generated in Liu et al. [15] showed consistent patterns and when lipid was compared with starch and protein, contours were almost parallel to x-axis which suggested that feed intake decreased with increased lipid concentration in broiler diets. Subsequently, Liu et al. [1] showed that increasing dietary lipid concentration from 40 to 75 g/ kg generated an 8.8% reduction in feed intake (1999 versus 1823 g/bird, P < 0.0001), a 15.9% reduction in weight gain (1526 versus 1284 g/bird, P < 0.0001) and FCR was compromised by 13.8% FCR (1.360 versus 1.547, P < 0.0001). The lipid-induced triggering of the ‘ileal brake’ may be the cause of feed intake reductions in diets with higher lipid concentrations. Martinez et al. [3] reported that the intraluminal infusion of lipids modulates gastrointestinal motility and delays gastric emptying by decreasing the frequency of the gastric cycle, increasing duodenogastric refluxes, and elongating the migrating myoelectric complex. Subsequently, Maljaars et al. [16] proposed that perfusion of fat into the ileum inhibits small intestinal digesta transit, gastric emptying, pancreaticobiliary and gastric acid secretion and food intake in mammals.
Dietary factors influencing lipid utilisation in poultry include the degree of saturation of fatty acids, the inclusion of lipid, the positional distribution of fatty acids within the glyceride molecule, the feed grain on which the diet is based, dietary calcium levels and feed processing [17]. Consistently, Maljaars et al. [16] concluded that the factors influencing inhibitory effects of lipid on gastric emptying which include fatty acid or triacylglycerol, chain length of fatty acids, degree of saturation of fatty acids and quantity of lipid. In humans, a dose-dependent decrease in pancreatic and biliary secretion was observed in response to doses of lipid of 0, 50 or 100 mg/min perfused into the ileum [18]. Therefore, it is possible that higher lipid intake triggered the ‘ileal brake’ and reduced feed intake. Another possible reason for reduced feed intakes with higher dietary lipid concentration could be the inferior pellet quality. It is recognised in practice that dietary lipid is required to maintain the throughput of feed-mill; however, high inclusion of lipid, especially vegetable oils, may compromise pellet hardness and quality. Kleyn [2] suggested a non-linear formulation approach for diets with high lipid levels because dietary energy densities may not increase with dietary lipid concentrations in a linear manner.
With the dietary protein and starch concentration comparisons in Fig 1, the contours almost parallel the y-axis and this indicates dietary protein concentration had a more pronounced impact on feed intake than starch and feed intakes were reduced with increased protein concentrations. Suthama et al. [19] found that broiler chickens offered diets containing high protein concentrations (306 g/kg) had their feed intakes significantly reduced by 26.9% (588 versus 804 g/bird) in comparison to birds offered a low protein (201 g/kg) but iso-energetic diet from 15 to 27 day post-hatch. Liu et al. [1] reported quadratic effects of dietary protein concentration on feed intake in broiler chickens from 7–28 days post-hatch. When crude protein concentration was less than 200 g/kg, feed intake increased with increasing dietary protein concentrations; whereas, when crude protein concentration was higher than 240 g/kg, feed intake decreased with increasing dietary protein concentrations. The authors suggested that a balance between protein and non-protein energy was required for optimal growth performance in broiler chickens. In the present study, the dietary digestible lysine to energy ratios were fixed across all the experimental diets; however, non-protein energy was derived from either starch or lipid. Both lipid and protein had more pronounced impact on feed intake than starch (Fig 1).
Protein intake target
Simpson and Raubenheimer [20] advanced the protein leverage hypothesis and suggested humans and animals prioritised protein intake when they were forced to exchange protein intake against that of carbohydrate and lipid derived from nutritionally unbalanced diets. This means they tended to maintain a constant protein intake relative to starch and lipid intakes. The relationship between protein intake from 7 to 27 days post-hatch and protein to non-protein ratios in diets in the present study is shown in Fig 2. Protein intake maintained constant when the ratio of dietary protein and non-protein concentrations increased (R2 = 0.005) and this suggests that broiler chickens had a constant protein intake of approximately 450 g/bird during the experimental period regardless of the dietary protein proportions. In contrast, starch intakes increased linearly (R2 = 0.841, P < 0.0001) with its proportion in the diets. When the ratio of starch to non-starch dietary components increased, there was also an increase in the intake of starch.
There was a quadratic relationship between lipid intake and ratios of lipid to non-lipid components. Lipid intake increased with the ratio of lipid to nonlipid components in the diets and it reached maximum of 167 g/bird when the ratio of lipid to non-lipid components equaled 0.198. This suggests that broiler chickens do not eat to constant starch and lipid intake target; however, that lipid intake reached a plateau may be due to the impact of lipid on total feed intake in broiler chickens. Increasing dietary lipid concentrations from 23.5 to 71.0 g/kg or decreasing starch to lipid ratios significantly depressed feed intake by 10.5% (2343 versus 2098 g/bird, P = 0.007). This observation accords with the findings of Liu et al. [1] as they reported an 8.8% reduction in feed intake (1823 versus 1999 g/bird; P < 0.0001) in broiler chickens offered diets containing the higher lipid concentration. Consistently, high lipid diets in the present study contained higher fibre concentrations (mean values: 24.8 versus 53.4 g/kg) as shown in Table 2. Therefore, it appears that higher fibre concentrations in high lipid diets had negative impacts on feed intake in broiler chickens. Another possible explanation for the reduction of feed intake is the lipid-induced triggering of the ‘ileal brake’ as suggested in Martinez et al. [3]. These researchers found that intraluminal infusion of lipids in poultry modulates gastrointestinal motility including an increase in duodenogastric refluxes or episodes of reverse peristalsis. The researchers suggested that these actions could delay gastric emptying and increase transit time, which is consistent with the “ileal brake” mechanism similar to that described in mammals.
Protein and energy balance
It is straightforward that increased nutrient density improved feed conversion and energy utilisation (Table 5). A further possibility is the higher inclusions of crystalline amino acids in the high nutrient density diets. On average, the low nutrient density diets (1–4) contained 2.35 g/ kg crystalline amino acids; whereas, the high nutrient density diets (9–12) contained 9.55 g/kg crystalline amino acids. In addition to lysine, methionine and threonine, high nutrient density diets were also supplemented with tryptophan, valine, arginine and isoleucine. Melnick et al. [21] suggested that all essential amino acids must be liberated from protein at appropriate rates to optimise protein utilisation. Goldberg and Guggenheim [22] determined concentrations of lysine, methionine and tryptophan in the portal blood stream after feeding rats with different protein sources. Ten minutes after feeding casein, the lysine concentration of 153 μg/ ml exceeded the lysine concentration of 71 μg/ml from overheated soy flour by more than a two-fold factor. Thus the rate of protein digestion, absorption and transition of amino acids into the portal blood stream from ‘intact’ proteins can vary enormously. Crystalline or synthetic amino acids do not require digestion and are rapidly absorbed [23]. This was perhaps reflected in Table 4 as broiler chickens offered high density diets had significantly higher protein digestibilities and protein disappearance rates. On average, diet 9, 10 and 11 contained 11.4 g/kg synthetic amino acids which represented 5.8% of total dietary protein. Consequently, broiler chickens offered high nutrient density diets had significantly higher apparent protein digestibilities in the jejunum and ileum (P < 0.001).
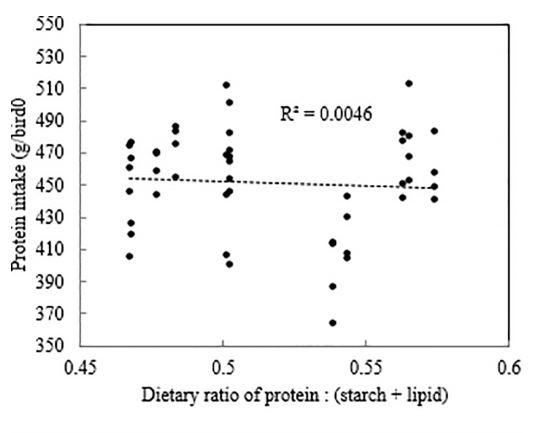
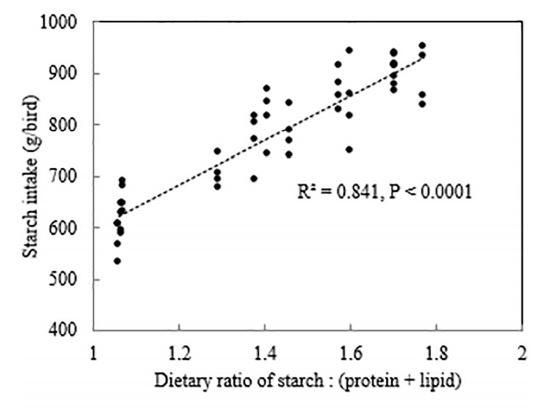
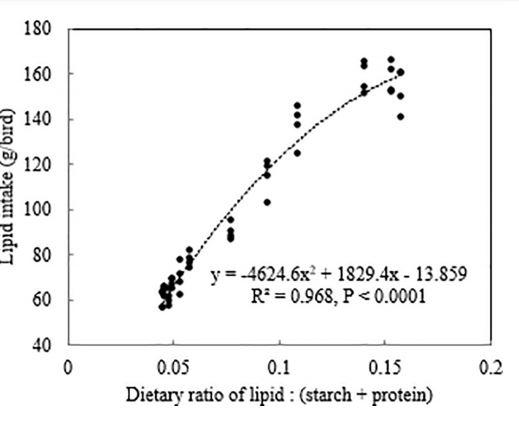
b -.02wTable 6 summaries the findings that feed intake was influenced by the balance of protein and energy and once the balance was achieved feed conversion efficiency may be improved. When the ratio between protein and energy was held constant, increasing nutrient density increased weight gain, reduced feed intake and improved feed conversion efficiency. When dietary protein was constant, increasing energy may increase or depress feed intake depending on the protein concentration in the diet. For example, Liu et al. [24] offered broiler chickens three diets to select from and all three diets contained 17.5 g/kg digestible lysine and 300 g/kg crude protein but different energy levels (11.04, 12.58 and 14.12 MJ/kg). Broiler chickens strongly preferred diets containing the highest energy to balance the high protein concentration and achieved an FCR of 1.217 compared to the average FCR of 1.473 from 10 to 31 days post-hatch. However, Gous et al. [25] reported reductions in feed intake when either dietary protein or energy were increased while the other was held constant when broiler chickens were offered only one diet at one time. This is consistent with the protein intake target theory discussed above and, broiler chickens consumed to constant protein intake and feed intake would be depressed once this target is attained.
Carcass composition
In the present study, weight gain and FCR were correlated (P < 0.05) with apparent digestibility coefficients of protein in the distal jejunum and distal ileum (Table 7). Increasing protein digestibilities in the distal jejunum and distal ileum increased weight gain and improved feed conversion efficiency. Consistently, weight gain and FCR were correlated (P < 0.05) with energy utilisation (AME, AME:GE and AMEn). There were no treatment effects on protein concentrations in carcass (P > 0.30). The balance between protein and energy is required for muscle protein deposition [12] and the lack of treatment response on carcass protein concentrations could be due to the fixed ratio of protein to metabolisable energy in the diets. Amino acid and glucose are both required for muscle protein deposition and a surplus of glucose may be deposit as body lipid [12]. On average, increasing dietary starch to lipid ratio from 4 to 14 increased lipid concentration in carcass from 9.61 to 11.17% (P = 0.018).
Conclusions
In the present study, increasing nutrient density increased weight gain, decreased feed intake and improved feed conversion efficiency in broiler chickens from 7 to 27 days post-hatch. Lipid had a more pronounced impact on feed intake than dietary starch concentrations and as predicted in Fig 1, increasing dietary lipid concentrations from 25 to 75 g/kg depressed feed intake from 2350 to 2100 g/bird. Broiler chickens tended to consume to a constant protein intake because protein intake did not change with the protein proportions in the experimental diets. A surplus of energy derived from starch may increase carcass lipid concentrations and the balance between protein and energy is pivotal for optimal feed conversion and body protein deposition. Protein and energy need to be considered in tandem in practical diet formulation, as diets containing high crystalline amino acid inclusions with low crude protein contents may require a lesser energy expenditure along the digestive tract. The impact of lipid on pellet durability and feed intake and the impact of starch on carcass lipid concentrations should also be taken into consideration.
Supporting information
S1 Table. Individual cage raw data. (XLSX).
Acknowledgments
We appreciate comments made by Mr Greg Hargreave and Professor Rob Gous for designing this experiment. We also acknowledge the technical assistance of Ms Joy Gill, Ms Melinda Hayter, Ms Kylie Warr and Mr Duwei Chen from Poultry Research Foundation for their assistance with bird management, sample collection and laboratory analyses.
Author Contributions
Conceptualization: Sonia Yun Liu.
Investigation: Ali Khoddami.
Methodology: Ali Khoddami, Peter V. Chrystal, Peter H. Selle, Sonia Yun Liu.
Project administration: Sonia Yun Liu.
Supervision: Sonia Yun Liu.
Writing – original draft: Sonia Yun Liu.
Writing – review & editing: Ali Khoddami, Peter V. Chrystal, Peter H. Selle, Sonia Yun Liu.
This article was originally published in PLoS ONE 13(10): e0205272. https://doi.org/10.1371/journal. pone.0205272. This is an Open Access article distributed under the terms of the Creative Commons Attribution License.