Introduction
The use of in ovo technology is a very innovative field in the research and industry application, including novel means of vaccination and embryonic nutrient supplementation. As defined by the early work developed by Uni and Ferket (2003), in ovo feeding is the administration of exogenous nutrients and other agents into the amnion of the late-term avian embryo, and it can advance the development of the growing embryo before and after hatch. Similarly, in ovo feeding technology could be used to benefit important physiological and biochemical stasis including enhancing oxidative protection, key adaptation function to metabolic stress during the perinatal period of rapid early development. Early perinatal development is a critical period to establish the expression of genetic potential that influence economically important production traits later in birds life (Ohta et al., 2001; Ipek et al., 2004; Uni and Ferket, 2004; Foye et al., 2005, Uni et al., 2005, Pedroso et al., 2006, Leitão et al., 2008, Leandro et al., 2010, Leitão et al., 2010). The ability to improve economically traits, such as average daily gain, feed conversion, meat yield, and disease resistance will lead to increased returns and efficiency for the industry (Schall, 2008). Considering that oxidative protection against reactive oxygen species (ROS) originated during body physiological processes starts in embryo early development, egg content can be a key factor in the extension of ROS generation causing deleterious effects in poultry metabolism (Rutz et al., 2002). The objective of this paper is to explore the potential applications of enhancing the antioxidant status of the chicks during those critical the last days of incubation.
Oxidative distress
Atoms are most stable in the ground state when every electron in the outermost shell has a complimentary electron that spins in the opposite direction. By definition, a free radical is any atom (e.g. oxygen, nitrogen) with at least one unpaired electron in the outermost shell, and is capable of independent existence (Karlsson, 1997). A free radical is easily formed when a covalent bond between entities is broken and one electron remains with each newly formed atom (Karlsson, 1997). Most biologically-relevant free radicals are derived from oxygen and nitrogen. Both these elements are important for animal life, but in some circumstances, they can be converted into free radical molecules (Surai et al., 2003). Free radicals are highly reactive due to the presence of unpaired electron(s). Any free radical involving oxygen can be referred to as reactive oxygen species (ROS) (Figure 1). Oxygen centered free radicals contain two unpaired electrons in the outer shell. When free radicals steal an electron from a surrounding compound or molecule a new free radical is formed in its place. Thus, a chain reaction of free-radical formation continues and can occur over a thousand of events (Goldfarb, 1999). About 2 to 5% of the total oxygen intake during both rest and exercise may form the highly damaging superoxide radical via electron escape (Sjodin et al., 1990).
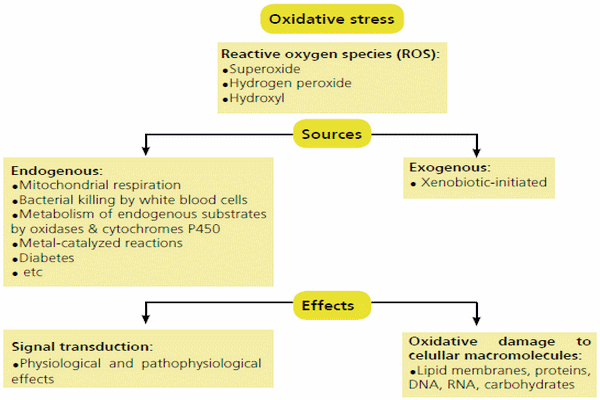
Figure 1 - Source of ROS and the general mechanisms by which oxidative stress can alter cellular function (from Well et al., 2009).
Lipoperoxidation
Polyunsaturated fatty acids (PUFAs) are abundant in cellular membranes and in low-density lipoproteins (LDL) (Dekkers et al., 1996). The PUFAs allows cellular membranes fluidity given passage to non hydro soluble substances. Free radicals prefer to steal electrons from the lipid membranes of a cell because of their proximity to pro-oxidant agents, thus initiating a free radical attack on the cell known as lipid peroxidation (Figure 2). Reactive oxygen species target the carbon-carbon double bond of polyunsaturated fatty acids. The double bond on the carbon weakens the carbon-hydrogen bond, allowing for easy dissociation of the hydrogen by a free radical. A free radical will steal the single electron from the hydrogen associated with the carbon at the double bond. In turn, this leaves the carbon with an unpaired electron and hence becomes a free radical. In an effort to stabilize the carbon-centered free radical molecular rearrangement occurs. The newly arranged molecule is called a conjugated diene (CD). The CD then very easily reacts with oxygen to form a proxy radical which steals an electron from another lipid molecule in a process called propagation. This process then continues in a chain reaction (Halliwell and Chirico, 1993), consisting in a propagation of free radicals and formation of non-radical products such as (ketones, ethers, alkanes, aldehydes, etc.). This intermediates and products can change with reaction conditions and time, representing a big threat to animal health status. The process continues indefinitely until no hydrogen source is available or the chain is intercepted.
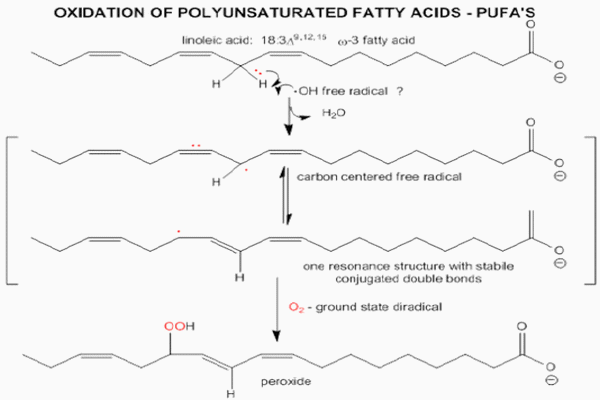
Figure 2 - Oxidative modification of lipids
Free radicals formation
Types of free radicals
There are numerous types of free radicals that can be formed within the animal. The most common ROS include the superoxide anion (O2-), hydroxyl radical (OH), singlet oxygen (O), and hydrogen peroxide (H2O2).
Superoxide anions; are formed when oxygen (O2) acquires an additional electron, leaving the molecule with only one unpaired electron. Superoxide anions (O2-) are continuously being formed within the mitochondria. The rate of formation depends on the amount of oxygen flowing through the mitochondria at any given time.
Hydroxyl radicals; are short-lived, but the most damaging radicals, within the body. This type of free radical can be formed from O2- and H2O2. Copper or iron catalysts and H2O2 also produce OH. These reactions are significant as the substrates are found within the body and could easily interact (Halliwell and Chirico, 1993).
Hydrogen peroxide; produced in vivo by many reactions. Hydrogen peroxide is unique in that it can be converted to the highly damaging hydroxyl radical or be catalyzed and excreted harmlessly as water. Glutathione peroxidase, a seleno-protein enzyme is essential for the conversion of glutathione to oxidized glutathione, during which H2O2 is converted to water (Goldfarb, 1999). If H2O2 is not converted into water, O is formed.
Singlet oxygen; is not a free radical, but can be formed during radical reactions and also cause further reactions. When oxygen is energetically excited one of the electrons can jump to an empty orbital, creating unpaired electrons (Karlsson, 1997). Singlet oxygen can then transfer the energy to a new molecule and act as a catalyst for free radical formation. The molecule can also interact with other molecules leading to the formation of a new free radical.
Factors that affect oxidative stress during incubation
According to Surai (2007), there are many factors that can interfere with the antioxidant status of a chick, and many of these oxidative stress factors act at different stages of development before hatch (Figure 3).
Figure 3 - Factors that can interfere with the antioxidant status of a chick at the end of incubation (Adapted from Surai, 2007).
Temperature, humidity and carbon dioxide concentration fluctuations during incubation can affect embryonic development, oxidation and phosphorylation in tissues, leading to the production of free radicals. For example, high carbon dioxide concentration during incubation have been shown to jeopardize the livability of the embryo. Everaert et al. (2007) demonstrated that broiler embryos when submitted to 4% CO2 pressure inside the incubator between 10th to 18th days, were not able to affect the relative growth of chicks until 7 days, when compared with the control group, however, corticosterone and thyroxine concentrations were significantly higher in the CO2 incubated chicks on 7 days post hatch.
The 19th day of embryonic development is an important point when risk of lipid peroxidation is very high for chickens, especially high meat yield strains of broilers. At this stage of development, the embryonic tissues are characterized by comparatively high levels of polyunsaturated fatty acids (PUFA). At the same time, natural antioxidant reserves have not reached a sufficient level for innate protection. Moreover, “internal piping” occurs at this critical stage and oxygen availability increases as pulmonary respiration begins. Low antioxidant status in combination with high temperatures, humidity, and PUFAs increases the embryo’s susceptibility to lipid peroxidation.
Hatching time is considered as an environmental stress for the chick. At this time, natural antioxidant (Vitamin C and Vitamin E) concentrations have reached a maximum level. However, the risk of peroxidation of the high concentrations of unsaturated lipids in tissues increases as the concentration of ascorbic acid needed to reduce oxidized vitamin E decreases, especially during high temperature and humidity conditions during the time of hatch.
Delay in collecting chicks from incubator can exacerbate oxidative stress of the hatching chick. Since not all chicks are hatched at the same time because the heterogeneous nature of the eggs set (i.e eggs from older breeders hatch earlier than those from young flocks and chicks from smaller eggs hatch earlier than those from larger eggs), some chicks may be in the incubator for 2 – 12 hours longer than others. This wide hatch window puts additional pressure on antioxidant defense capacity. Furthermore, any delay in food and/ or water intake after hatching usually negatively affects a number of performance parameters and delay occurs in the maturation of the enzymatic system that controls metabolism, free radical production and antioxidant protection systems.
Disease challenger of the breeder hens or progeny immediately after hatch significantly affects oxidative stress tolerance in perinatal chicks. Phagocytic immune cells themselves produce free radicals in the process of killing internalized pathogens. Without adequate antioxidant nutrient reserves, cellular membranes and hence, important organelles, can be damaged by the free radicals thereby reducing the effectiveness of the immune cell. In addition, Se is considered to have a specific role in immune system regulation, which could be independent of its antioxidant functions.
Vaccination is a substantial stress; and in some cases using vitamin E as a vaccine adjuvant can help improve vaccination efficiency. In ovo vaccination is a relatively new concept in vaccine administration and has replaced post-hatch injection of Marek’s disease vaccine in broiler chickens. On the other hand, the challenge of the immunization begins sooner in chick’s life. It’s important that the embryo has a good reserve of antioxidants to respond vaccination with high efficiency. Bhattacharyya et al. (2007) showed improvement of immune response in Turkeys with in ovo supplementation of carbohydrates.
Early antioxidant feeding: How this can help?
After the fertile egg is laid, it must contain all the nutrients to nourish and protect the developing embryo until the chick hatches and begins to consume and assimilate sufficient nutrients to support subsequent development and growth. But some adverse events during incubation can either impede embryonic nutrient utilization or increase their demand to maintain metabolic stasis. These adverse incubation events may be related to management of the incubation, environment, air quality, contamination, genetic factors, and many other issues. In ovo administration of some compounds that positively addresses oxidative stress may alleviate the associated adverse effects on chick growth and welfare. Some of these antioxidant compounds could enhance or protect critical physiological or biochemical functions leading to improved health and welfare of the perinatal chick.
Vitamins
Vitamin E (VE) is the major fat-soluble antioxidant, that quenches the chain reaction of lipid peroxidation. The antioxidant system of the brain is of great importance because of the development of nutritional encephalomalacia, which occurs in young chicks as results of VE deficiency (Dror and Bartov, 1982; Bhanja et al., 2007). The VE is well known for its antioxidant properties and is often used as the standard by which all other antioxidants are compared. The VE is distributed in all compartments in the egg, and the concentrations changes as the incubation period progresses (Figure 4) (Noble et al., 1993). The use of antioxidants, like VE has been used to alleviate the adverse effects of the peroxidation of lipids, and cholesterol, in domestic animals (Singh et al., 2005). Surai et al. (1997, 1999) observed a highly positive correlation between dietary VE levels in the maternal hen’s diet and the levels of VE in egg and subsequent in chick tissues. The VE levels can be correlated with the immune response of chicks. Gore and Qureshi (1997) observed a positive correlation between VE administered in ovo on immune system and immunoglobulin levels in turkey poults and broiler chicks at 7 and 14 days post-hatch. Moreover, Schaall (2008) demonstrated that in ovo VE administration was able to change the total lipid content of tissues at hatch time, especially in the brain and plasma. Schaall (2008) concluded that VE enhances the antioxidant status of hatched chick’s tissues and protects lipid membranes from radical oxygen species.
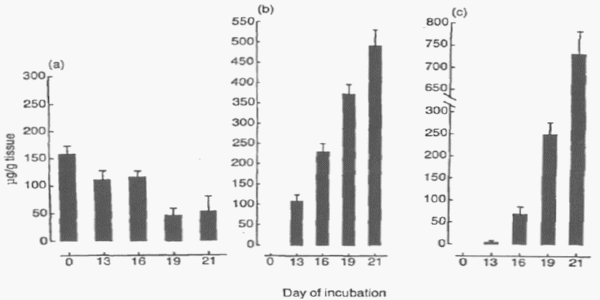
Figure 4 - Alfa-Tocopherol concentrations in: (a) yolk contents; (b) yolk sac membrane; and (c) liver of the chick embryo during incubation. Each result is the mean ±SE of 6 observations (from Noble et al., 1993).
Vitamin C (ascorbic acid, AA) has been demonstrated to improve immunoresponsiveness and increase disease resistance in poultry by optimizing the immune system (Pardue et al., 1985; Rund, 1989). Ascorbic acid has also been shown to have a sparing effect on vitamin E by acting as a redox system reducing tocopheroxyl radicals back to their reduced state tocopherol (Wilson, 1983). Ipek et al. (2003) utilized in ovo injection during the later part of incubation to study the effect of AA and glucose on embryonic mortality, hatchability, chick quality, and chick weight of broiler chickens embryos. These researchers observed that in ovo injection of 3 mg AA per egg resulted in a higher hatchability rate. Zakaria and Al-Anezi (1996) observed the best hatchability results for chickens when 3 mg/egg of vitamin C were injected on day 15 of incubation. Nowaczewski et al. (2011) demonstrated that in ovo injection of 6 mg of vitamin C per egg on the 20th day of incubation (8 days before hatch) improved hatchability rate of ducklings.
Carotenoids
Many studies have already shown that dietary carotenoids have a very participative role in the antioxidant defense mechanisms of live organisms. Carotenoids are lipid-soluble organic compounds that pigment and protect the integument of many organisms by absorbing light and UV radiation, and many carotenoids provide antioxidant protection and immunomodulation (Goodwin, 1986). In humans, several authors suggest an important relationship exist between carotenoids and the prevention of cancer (Mathews-Roth, 1985; Krinsky, 1989; Ziegler, 1991). It has been suggested that carotenoids may act as anti-carcinogenic agents by an antioxidant mechanism independent of their conversion to vitamin A (Peto et al., 1981). Most studies have focused on the antioxidant properties of -carotene and research has been largely conducted in liposomes (Krinsky and Deneke, 1982), lipoproteins (Jialal et al., 1991), and isolated membranes (Palozza et al., 1992). Lawlor and O’Brien (1995) demonstrated the effects of -carotene on chicken embryo fibroblast when challenged with different concentration of b-carotene and with or without a presence of paraquat (Potent oxidative agent). They concluded that b-carotene was effective as an antioxidant in low concentrations of (0.1μM), but it was an effective pro-oxidant at high concentrations (10μM). Koutsos et al. (2006) demonstrated that chicks with a lack of carotenoid exposure, either in ovo or post hatch, increase parameters of systematic inflammation.
L- Carnitine
L-Carnitine acts as an antioxidant that ultimately results in a decrease in reactive oxygen species by removing excessive levels of intracellular acetyl- CoA, which induces mitochondrial reactive oxygen species production (Vicari and Calogero, 2001; Agarwal and Said, 2004; Agarwal et al., 2005). Free radicals responsible for lipid peroxidation are scavenged by L-carnitine (Rebouche, 1992). Other antioxidants, such as ascorbic acid, vitamin E, and antioxidant enzymes, are protected against potential peroxidation by L-carnitine (Kalaiselvi and Panneerselvam, 1998). In addition to its role as an antioxidant, L-carnitine transports long chain fatty acids across mitochondrial membranes for b-oxidation of fatty acids. Under circumstances of increased metabolism, when the demand for energy escalates, L-carnitine availability could become a limiting factor for b-oxidation of fatty acids. In such situations, exogenous supplementation of L-carnitine could prove advantageous (Buyse et al., 2001) and could in turn be used by the chick during hatching. Zhai et al. (2008) concluded that the in ovo injection of dosimetric dosages of L-carnitine in the range of 0.05 to 10 μmol into fertile Leghorn eggs at 17 or 18 d of incubation did not affect hatchability, yolk sac weight, or BW. The possibility exists that dosages of L-carnitine greater than 10 μmol could improve hatchability and hatchling BW because there was no evidence of carnitine toxicity with the dosimetry utilized in the current study. Moreover, the response of broiler and turkey hatching eggs to in ovo carnitine injections may differ from Leghorn embryos. Differences in lipid metabolic rate between egg-laying and meat-type strains of poultry could influence response to in ovo injection of L-carnitine (Sato et al., 2006).
Glucose
According to Bhattacharyya et al. (2007), the maintenance of glucose homeostasis during few days pre and post-hatch is among greatest challenge of a chick’s life. The primary source of glucose need for hatching activities is the liver and gluconeogenesis from protein of amnion and muscle. Glycolysis, rather than fatty acid oxidation, is needed during hatching to provide energy, as oxygen supply is limited during the transition from chorio-allantois to pulmonary respiration (Hoiby et al., 1987). At day of hatch glycogen stores decrease substantially, and remain low until the newly hatched chick has the reserves access to oxygen, necessary to mobilize and utilize body fat reserves and assimilate external diet (Hazelwood, 2000). Just after hatch, the chick undergoes rapid physical and functional development of the gastrointestinal tract for digestion and assimilation of nutrients. However, in most commercial operations, the chicks get access to feed only after several hours after hatching. This may decrease hatchling weight and immune-competence of chicks. These limitations in early energy status may be alleviated by administering refined carbohydrates or amino acids into the amnion or yolk sac (in ovo feeding) at different days of incubation, thereby improving chick livability and growth (Uni and Ferket, 2004; Branja et al., 2004). It has already been noted that glucose added to drinking water helps to suppress gluconeogenic enzyme activity (Donaldson, 1995), which is necessary to sustain blood glucose homeostasis. The digestive tract of the hatchlings has limited ability for digestion and utilization of diet rich in proteins and carbohydrates (Uni and Ferket, 2004). Hence, early access to highly digestible feed is beneficial but the benefits are solely dependent on the initiation of feed consumption unlike in ovo feeding. It has been observed that in ovo feeding of carbohydrates into the amnion increase hatchling weight in broiler and turkeys (Ferket and Uni, 2002).
Regulatory peptides
Some regulatory peptides could have some antioxidant properties. Zheng et al. (2010) reported that leptin has a considerable role in anti-oxidant defense of body by activating super oxide dismutase. Ghrelin is another multifunctional regulatory peptide. After identification of ghrelin in mammals by Kojima et al. (1999) many relative studies were conducted to better understand it’s metabolic functions. Studies conducted during last decade revealed various homeostatic functions for ghrelin, such as the release of growth hormone (Hashizume et al., 2005), osteogenesis (Fukushima et al., 2005; Delhanty, 2008), hematopoiesis (Akarsu, et al., 2007; Dixit et al., 2007), food intake and energy balance and regulation (Nakazato et al., 2001; Toshinai et al., 2003), endocrine/ paracrine roles in pancreas (Stevanovi! et al., 2007, Dezaki et al., 2008), and currently antioxidative effects (Kheradmand et al., 2008; Kheradmand et al., 2010). Peptide structure of chicken ghrelin has 26 amino acids with 54% similarity to rat ghrelin (Kaiya et al., 2008). Ghrelin has been identified in albumen and yolk of fertilized chicken egg (Yoshimura et al., 2009). Malondialdehyde (MDA) (CH2(CHO)2) is a biomarker of oxidative stress in an organism and an increase of MDA level demonstrates oxidative stress (Nielsena et al., 1997). Anti-oxidative activity of ghrelin has been reported in numerous studies (Kheradmand et al., 2009; Kheradmand et al., 2010; Íseri et al., 2005). Íseri et al. (2005) reported the anti-oxidative activity of ghrelin and its ameliorative ability for alendronate-induced oxidative stress, as MDA comes back to the control level after ghrelin infusion. The chick embryo attempts to increase capacity of its antioxidant defenses as incubation progresses towards hatch (Stock et al., 1990). Although the anti-oxidative effects of ghrelin have been studied in mammalian models, its antioxidant function in birds is unclear. Lotfi et al. (2012) described in ovo administration of ghrelin on day 5 of incubation was more efficient in reducing MDA than when administered on day 10 (4.10 and 4.60 nmol/mL in comparison with 9.53 and 9.50 nmol/mL, respectively). Apparently in ovo ghrelin supplementation during early embryonic development (i.e. day 5 of incubation) had an antioxidant protective effect on the embryo as indicated by a significant decrease in serum MDA level of newly-hatched chicks. These researchers concluded that in ovo administration of ghrelin on day 5 and day 10 of incubation at the dosages of 50 and 100 ng/egg can have anti-oxidative protection and decrease serum MDA level. Further studies with measuring specific enzymes (superoxide dismutase, glutathione peroxidase, etc) activity in blood or tissues of newly-hatched chicks following in ovo administration of ghrelin on different incubation days are suggested for further clearness of antioxidant properties.
Elements to use in ovo with potential antioxidant effects
Thiamin (Vitamin B1) Thiamin (Vitamin B1) is a co-factor for several enzymes catalyzing descarboxylation and transkeletolation type reactions. Deficiency of thiamin in eggs causes high mortality of embryos just prior to hatching, and chicks that hatch exhibit symptoms of polyneuritis (Charles et al., 1972). Deficiency of vitamin B6 (Pyridoxine) leads to early embryonic death (Landauer, 1967) and decreases IgM and IgG response to antibody challenger (Blalock et al., 1984).
Essential oils
The essential oils (EOs) also called volatile or ethereal oils, are aromatic oily liquids obtained from plant material. EOs are complex mixtures of secondary plant metabolites consisting of low boiling-phenylpropenes and terpenes. Many sources of antioxidant of plant origin and antioxidant properties of many aromatic plants and spices have been studied in recent years.. Among these, the antioxidant properties of many aromatic plants and spices have shown to be effective in retarding the process of lipid peroxidation in oils and fatty acids. Particularly rosemary, oregano and sage have been extensively studied for their antioxidant activity (Brenes and Roura, 2010). Cinammon has also been demonstrated by Kamel (1999), to have a similar antioxidant activity as the synthetic antioxidant BHT. Due to the oily characteristics of these products, the use in ovo supplementation has some restrictions mainly because of liposoluble characteristics of this substances.
Trace minerals
Individual trace minerals levels in the avian egg are variable and the total amount deposited in the egg depends on the mineral chemical form and the amount fed to the hen (Naber, 1979; Stadelman and Pratt, 1989). Changes in environmental conditions during incubation can impact avian embryonic trace mineral metabolism. Briefs periods of hypoxia (reduced oxygen tension, 15% O2) or hyperoxia (increased oxygen tension, 60% O2) during the late stages of incubation will alter growth, weight of the extra-embryonic membranes, and tissue trace mineral homeostasis in the chick embryo (Richards et al., 1991). Wilson et al. (1992) studied hepatic zinc, copper, and iron levels in the chick embryo and the levels of specific antioxidant enzymes that require these metals as co-factors. They found a correlation between catalase activity and its co-factor, iron, in liver and reported a positive relationship between hepatic zinc and oxygen consumption by the embryo, as well as changes in brain levels of superoxide dismutase (CuZn-SOD), a copper and zinc-containing enzyme. Marked differences in the developmental profiles of tissue antioxidant enzyme system were found for brain versus liver; however, the relative abundance of metal cofactors did not seem to influence the activity of most antioxidant enzymes in these two tissues during development (Richards, 1997).
Selenium also can provide beneficial impact in redox system, since this mineral act in glutathione peroxidase activity, an important enzyme of body oxidative system protection. Sefton and Edens (2004) showed that hatch can be improved by selenium supplementation in breeder’s diets, since this mineral is transferred to the eggs after layer. According to Surai (2006), selenium in the liver of newly hatched bird is rapidly diluted in post hatch. In ovo supplementation of selenium might be represent a good alternative to prevent this depletion in young broilers preventing possible injuries due to oxidative peroxidation in body system.
Conclusion
In conclusion, oxidative stress is among the most limiting threatening conditions that the avian embryo must overcome as it develops towards hatch through to the initiation of feed consumption. Although the embryo is endowed with various antioxidant compounds deposited by the hen to combat oxidative damage, incubation distress can place a significant drain on these limited antioxidants, and consequently adversely affect critical physiological and metabolic functions. The antioxidant status of the embryonated egg is determined by what the hen deposits, which is influenced by her diet, nutritional and physiological state, and her own antioxidant status. Because antioxidant status of hatching eggs are variable and unpredictable, in ovo supplementation of antioxidants by in ovo feeding technology may prove to yield significant benefits. Indeed, the few preliminary studies indicate the potential benefits of in ovo feeding solutions containing various antioxidant compounds, including vitamin E, ascorbic acid, L-carnitine, organic trace minerals, carotenoids, and antioxidant peptides. However, more research is necessary to better assess the practical application of antioxidants by in ovo feeding on subsequent health and growth potential of commercial broilers and turkeys.
Presented at the World´s Poultry Congress 5 - 9 August - 2012, Salvador, Bahia, Brazil.
References
AGARWAL, A., PRABAKARAN, S.A. and SAID, T.M. (2005) Prevention of oxidative stress injury to sperm. Journal of Andrology 26:654-660.
AGARWAL, A. and SAID, T.M. (2004) Carnitines and male infertility. Reproductive BioMedicine Online 8:376- 384.
AKARSU, S., USTUNDAG, B., GURGOZE, M.K., SEN, Y. and AYGUN, A.D. (2007) Plasma Ghrelin levels in various stages of development of iron deficiency anemia. Journal of Pediatric Hematology Oncology 29:384-387.
ALESSIO, H.M. and BIASI, E.R. (1997) Physical activity as a natural antioxidant booster and its effects on a healthy lifestyle. Research Quarterly for Exercise & Sport 68:292-302.
BHANJA, S.K., MANDAL., A.B. and GOSWAMI, T.K. (2004) Effect of in ovo injection of amino acids on growth, immune reponse, development of digestive organs and carcass yield of broiler. Indian Journal of Poultry Science 39:212-218.
BHANLA, S.K., MANDAL, A.B., AGARWAL, S.K., MAJUMDAR, S., and BHATTACHARYYA, A. (2007) 16th European Symposium on Poultry Nutrition, Strasbourg, France, August, pp. 26-30.
BHATTACHARYYA, A. MAJUMDAR, S., BHANJA, S.K., MANDAL, A.B., DASH, B.B. and AGARWAL, S.K. (2007) Effects of in ovo injection of glucose on growth, immunocompetence and development of digestive organs in turkey poults. 16th European Symposium on Poultry Nutrition, Strasbourg, France, pp. 26-30.
BLALOCK, T.L., HAXTON, J.P. and GARLICH, J.D. (1984) Humoral immunity in chicks experiencing marginal vitamin B6 deficiency. The Journal of Nutrition 114: 12-322.
BRENES, A. and ROURA, E. (2010) Essential oils in poultry nutrition: Main effects and modes of action. Animal Feed Science and Technology 158:1-14.
BUYSE, J., JANSSENS, G.P. and DECUYPERE, E. (2001) The effects of dietary l-carnitine supplementation on the performance, organs weights and circulating hormone and metabolite concentration of broiler chickens reared under a normal or low temperature schedule. British Poultry Science. 42:230-241.
CHARLES, O.W., RONALD, D.A. and EDWARDS JR, H.M. (1972) Thiamine deficiency identification and treatment in commercial turley and Coturnix quail. Poultry Science 51:419-423.
DEKKERS, J.C., VAN DOORNEN, L.J.P. and KEMPER, C.G. (1996) The role of antioxidant vitamins and enzymes in the prevention of exercise-induced muscle damage. Sports Medicine 21, 213-238.
DELHANTY, P. (2008) Ghrelin and bone. Proceedings of 10th European Congress of Endocrinology, Berlin, pp. S7.3.
DEZAKI, K., SONE, H. and YADA, T. (2008) Ghrelin is a physiological regulator of insulin release in pancreatic islets and glucose homeostasis. Pharmacology & Therapeutics 118:239-249.
DIXIT V.D., YANG, H., SUN, Y., WEERARATNA, A.T., OUM, Y.H., SMITH, R.G. and TAUB, D.D. (2007) Ghrelin promotes thymopoiesis during aging. The Journal of Clinical Investigation 117:2778-2790.
DROR, Y. and BARTOV, I. (1982) Dietary factors affecting experimental models of nutritional encephalomalacia. Poultry Science 61:84-93.
EVERAERT, N., KAMERS, B., WITTERS, A., De SMIT, L., DEBONNE, M., DECUYPERE, E. and BRUGGEMAN, V. (2007) Effect of four percent carbon dioxide during the second half of incubation on embryonic development, hatching parameters, and posthatch growth. Poultry Science 86:1372-1379.
FERKET, P.R. and UNI, Z. (2002) Early enteric development of turkeys. Proceedings of the 25th technical turkey conference held at Shrigley Hall Hotel on 24-26 April, pp. 59-64.
FOYE, O., FERKET, P. and UNI, Z. (2005) The effects of in ovo feeding of protein and beta-methyl-beta hidroxybutyrate (HMB) on nutrient digestion and absorption in neonatal turkey poults. International Poultry Scientific Forum, Atlanta, pp. 5.
FUKUSHIMA, N., HANADA, R., TERANISHI, H., FUKUE, Y., TACHIBANA, Y., ISHIKAWA, H., TAKEDA, S., TAKEUCHI, Y., FUKUMOTO, S., KANAGAWA, K., NAGATA, K. and KOJIMA, M. (2005) Ghrelin direct regulates bone formation. Journal of Bone and Mineral Research 20:790-798.
GOLDFARB, A.H. (1999) Nutritional antioxidant as therapeutic and preventive modalities in exerciseinduced muscle damage. Canadian Journal of Applied Physiology 24:249-266.
GOODWIN, T.W. (1986) Metabolism, nutrition, and function of carotenoids. Annual Review of Nutrition 6, 273-297.
HALLIWELL, B. and CHIRICO, S. (1993) Lipid peroxidation: Its mechanism, measurement, and significance. The American Journal of Clinical Nutrition 57, 715S-724S.
HASHIZUME, T., HORIUCHIA, M., NONAKAA, S., KASUYA, E., KOJIMA, M., HOSODA, H. and KANGAWA, K. (2005) Effects of ghrelin on growth hormone secretion in vivo in ruminants. Regulatory Peptides 126:61-65. HAZELWOOD, R.L. (2000) Pancreas, in: WHITTOW, G.C. (Ed) Sturkie Avian Physiology 5th, pp. 539-555 (San Diego, Academic Press).
HOIBY, M., AULIE, A. and BJONEES, P.D. (1987) Anaerobic metabolism in fowl embryos during normal incubation. Comparative Biochemistry Physiology 86:91-94.
IPEK, A., SAHAN, U. and YILMAZ, B. (2004) The effect of in ovo ascorbic acid and glucose injection in broiler breeders eggs on hatchability and chick weight. Archiv für Geflügelkunde, 68:132-135.
ÍSERI, S.O., SQENER, G., YÜKSEL, M., CONTUK, G., ÇETINEL, "., GEDIK, N., and YE"EN B.Ç. (2005) Ghrelin against alendronate-induced gastric damage in rats. Journal of endocrinology 187:399-406.
JIALAL, I., NORKUS, E.P., CRISTOL, L. and GRUNDY, S.M. (1991) "-Carotene inhibits the oxidative modification of low-density lipoproteins. Biochimica et Biophisica Acta 1086:134-138.
KAIYA, H., MIYAZATO, M., KANGAWA, K., PETER, R.E. and UNNIAPPAN, S. (2008) Ghrelin a multifunctional hormone in non-mammalian vertebrates. Comparative Biochemistry and Physiology Part A: Molecular & Integrative Physiology 149:109-128.
KALAISELVI, C.J. and PANNEERSELVAM, C. (1998) Effects of L-carnitine on the status of lipid peroxidation and antioxidants in aging rats. The Journal of Nutritional Biochemistry 9:575-581.
KAMEL, C. (1999) Use of plants extracts in European pig diets. Feed Compounder 19:23-27.
KARLSSON, J. (1997) Exercise, muscle metabolism and the antioxidant defense. World Review of Nutrition and Dietetics 82, 81-100.
KHERADMAND, A., ALIREZAEI, M., ASADIAN, P. RAFIEI ALAVI, E., JOORABI, S. (2009) Antioxidant enzyme activity and MDA level in the rat testis following chronic administration of ghrelin. Andrologia. 41:335- 340.
KHERADMAND, A., ALIREZAEI, M. and BIRJANDI, M. (2010) Ghrelin promotes antioxidant enxyme activity and reduces lipid peroxidation in the rat ovary. Regulatory Peptides 162:384-389.
KINSKY, N.I. (1989) Carotenoids and cancer in animal models. The Journal of Nutrition 119:123-126.
KOJIMA, M., HOSODA, H., DATE, Y., NAKAZATO, M., MATSUO, H., and KANGAWA, K. (1999) Gherlin is a growth-hormone-releasing acylated peptide from stomach. Nature 402:656-660.
KOUTSOS, E.A., LOPES, J.C.G. and KLASING, K.C. (2006) Carotenoids from in ovo or dietary sources blunt systematic indices of the inflammatory response in growing chicks (Gallus gallus domesticus). The Journal of Nutrition, 136:1027-1031.
KRINSKY N.I. and DENEKE, S.M. (1982) Interaction of oxygen and oxy-radicals with carotenoids. Journal of the National Cancer Institute 69:205-210.
LANDAUER, W. (1967) The hatchability of chicken eggs as influenced by environmental and hereditary. Monograph, Storrs University of Connecticut Agricultural Experimental Station.
LAWLOR, S.M. and O’BRIEN, N.M. (1995) Modulation of oxidative stress by!-carotene in chicken embryo fibroblasts. British Journal of Nutrition, 73:841-850.
LEANDRO, N.S.M., OLIVEIRA, A.S.C, GONZALES, E, CAFÉ, M.B., STRIGHINI, J.H. and ANDRADE, M.A. (2010) Probiótico na ração ou inoculado em ovos embrionados: Desempenho de pintos de corte desafiados com Salmonella Enteritidis. Revista Brasileira de Zootecnia 39:1509-1516.
LEITÃO, A.R., LEANDRO, N.S.M., STRINGHINI, H.J., CAFÉ, M.B. and ANDRADE, M.A. (2010) Inoculação de maltose, sacarose ou glicose em ovos embrionados de baixo peso. Acta Scientiarum. Animal Sciences 32, 93-100.
LEITÃO, R.A., LEANDRO, N.S.M., CAFÉ, M.B., STRINGHINI J.H., PEDROSO, A.A. and CHAVES, L.S. (2008) Inoculação de glicose em ovos embrionados de frango de corte: parâmetros de incubação e desempenho inicial. Ciência Animal Brasileira 9:847-855.
LOTFI, A., SHAHRYAR, H.A., EBRAHIMNESHAD, Y. and SHAYEGH, J. (2012) Effects of in ovo ghrelin administration on serum malondialdehyde level in newly-hatched chickens. Asian Pacific Journal of Tropical Biomedicine 2:47-49.
MATHEWS-ROTH, M.M. (1985) Carotenoids and cancer prevention - experimental and epidemiological studies. Pure and Applied Chemistry 57:717-722.
NABER, E.C. (1979) The effect of nutrition on the composition of eggs. Poultry Science 58:518-528.
NAKASATO, M., MURAKAMI, N., DATE, Y., KOJIMA, M., MATSUO, H., Kenji KANGAWA, K. and MATSUKURA, S. (2001) Role of ghrelin in the central regulation of feeding. Nature 409:194-198.
NIELSENA, F., MIKKELSEN B.B., NIELSEN, J.B., ANDERSEN, H.R. and GRANDJEAN, P. (1997) Plasmamalondialdehyde as biomarker for oxidative stress: reference interval and effects of life-style factors. Clinical Chemistry 43:1209-1214.
NOBLE, R.C., COCCHI, M. and BATH, H.M. (1993) !-Tocopherol absortion and polyunsaturated fatty acid metabolism in developing chick embryo. British Poultry Science 34:815-818.
NOWACZEWSKI, S., KONTECKA, H. and KRYSTIANIAK, S. (2012) Effect of in ovo injection of vitamin C during incubation on hatchability of chickens and ducks. Folia Biologica 60:93-97.
OHTA, Y., KIDD, M.P. and ISHIHASHI, T. (2001) Embryo growth and aminoacid concentration profiles of broiler breeder eggs, embryos and chicks after in ovo administration of aminoacids. Poultry Science 80:1430-1436.
PALOZZA, P. and KRINSKY, N.I. (1992) !-Carotene and !- Tocopherol are synergistic antioxidants. Archives of Biochemistry and Biophysics 297:184-187.
PARDUE, S.L., THAXTON, J.P. and BRAKE, J. (1985) Role of ascorbic acid in chicks exposed to high environmental temperatures. Journal of Applied Physiology 58:1511- 1516.
PEDROSO, A.A., CHAVES, L.S., LOPES, K.L.A.M., LEANDRO, N.S.M., CAFÉ M.B. and STRIGHINI J.H. (2006) Inoculação de nutrientes em ovos de matrizes pesadas. Revista Brasileira de Zootecnia 35:2018- 2026.
PETO, R. DOLL, R.J., BUCKLEY, J.D. and SPORN, M.B. (1981) Can dietary !-Catotene materially reduce human cancer rates? Nature 290:201-208.
QURESHI, M.A. and GORE, A.B. (1997) Vitamin E exposure modulates prostaglandin and thromboxane production by avian cells of the mononuclear phagocytic system. Immunopharmacol Immunotoxicol. 19:473-487.
REBOUCHE, C.J. (1992) Carnitine function and requeriments during the life cycle. FASEB Journal 6:3379-3386.
RICHARDS, M.P. (1991) Mineral metabolism in the developing turkey embryo I. The effects of developmental age and shell-less culture on trace elements content of selected tissues. Comparative Biochemistry and Physiology 100:1009-1016.
RICHARDS. M.P. (1997) Trace mineral metabolism in the avian embryo. Poultry science 76:152-164.
RUND, B. (1989) Vitamin C plays a role in immunity. Poultry Digest. 48:44-55.
RUTZ, F., BERMUDEZ, V., PAN, E.A. and FISCHER, G. (2002) Impacto da nutrição vitamínica sobre a resposta imunológica das aves. Simpósio Brasil Sul deAvicultura, Chapecó, pp. 105-117.
SATO, M., TACHIBANA, T. and FURUSE, M. (2006) Heat production and lipid metabolism in broiler and layer chicken during embryonic development. Comparative Biochemistry and Physiology Part A: Molecular & Integrative Physiology 143:382-388.
SCHAAL, T.P. (2008) The effects of in ovo feeding of fatty acids and aAntioxidants on broiler chicken hatchability and chick tissue lipids. Honors Scholar, Oregon State University.
SJODIN, T., WESTING, Y.H. and APPLE, F.S. (1990) Biochemical mechanisms for oxygen free radical formation during exercise. Sports Medicine 10:236- 254.
STADELMAN, J.L. and PRATT, D.E. (1989) Factors influencing composition of the hen’s eggs. World’s Poultry Science Journal 45:247-266.
STEVANOVIC, D., MILOSEVIC, V., STARCEVIC, V.P. and SEVERS, W.B. (2007) The effect of centrally administered ghrelin on pituitary ACTH cells and circulating ACTH and corticosterone in rats. Life Sciences 80:867-872.
STOCK, M.K., SILVERNAIL, K.K. and METCALF, J. (1990) Prenatal oxidative stress: I. Malondialdehyde in hypoxic and hyperoxic chick embryos. Free Radical Biology and Medicine 8:313-318.
SURAI, P.F. (2002). Natural antioxidants in avian nutrition and reproduction. Nottingham University Press, Nottingham: 616 p.
SURAI, P.F. (2006) Selenium in nutrition and health, Nottingham University Press, Nottingham: 974 p.
SURAI, P.F. (2007) Natural Antioxidant in poultry nutrition: new developments. 16th European Symposium on Poultry Nutrition, Strasbourg, pp. 26-30.
SURAI, P.F., KARADAS, F. and SPARKS, N.H. (2003) The importance of antioxidants in poultry. Annual North Carolina Poultry Nutrition Conference, p.38-56.
TOSHINAI, K., DATE, Y., MURAKAMI, N., SHIMADA, M., MONDAL, M.S., SHIMBARA, T., GUAN, J.L., WANG, Q.P., FUNAHASHI, H., SAKURAI, T., SHIODA, S., MATSUKURA, S., KANGAWA, K. and NAKAZATO, M. (2003) Ghrelin-induced food intake is mediated via the orexin pathway. Endocrinology 144:1506-1512.
UNI, Z., FERKET P.R., TAKO, E. and KEDAR, O. (2005) In ovo feeding improves energy status of late-term chicken embryos. Poultry Science 84:764-770.
UNI, Z. and FERKET, P.R. (2004) Methods of early nutrition and their potential. World’s Poultry Science Journal 60:101-111.
UNI, Z. and FERKET. P.R. (2003) Enhancement of development of oviparous species by in ovo feeding. North Carolina State University and Yissum Research Development Company Assignees, US Pat No 6,592,878.
VICARI, E. and CALOGERO, A.E. (2001) Effects of treatment with carnitines in infertile patients with prostate-vesiculo-epididymitis. Human Reproduction 16:2338-2342.
WELL P.G., MCCALLUM, G.P., CHEN, C.S., HENDERSON, J.T., LEE, C.J.J., PERSTIN, J., PRESTON, T.J., WILEY, M.J. and WONG, A.W. (2009) Oxidative stress in development origins of disease: Teratogenesis neurodevelopmental deficits, and cancer. Toxicology Sciences, 18:4-18.
WILSON, J.X., LUI, E.M.K. and DEL MAESTRO, R.F. (1992) Developmental profiles of antioxidant enzymes and trace metals in the chick embryo. Mechanisms of Ageing and Development 65:51-64.
WILSON, R.L. (1983) Free radicals protection: Why vitamin E not vitamin C, b-carotene, or gluthathione? Biology of vitamin E. Ciba Foundation Symposium 101:19-37.
ZAKARIA, A.H. and AL-ANEZI, M.A. (1996) Effects of ascorbic acid and coolong during egg incubation on hatchability culling, mortality, and body weights of broilers chickens. Poultry Science, 75:1204-1209.
ZHAI, W., NEUMAN, S., LATOUR, M.A. and HESTER, P.Y. (2008) The effect of in ovo injection of L-carnitine on hatchability of white leghorns. Poultry Science 87:569-572.
ZHENG, J., FANG, J., YIN, Y.J., WANG, X.C., REN, A.J., BAI, J., SUN, X.J., YUAN, W.J. and LIN, L. (2010) Leptin protects cardiomyocytes from serum-deprivationinduced apoptosis by increasing anti-oxidant defense. Clinical and Experimental Pharmacology and Physiology 37:955-962.
ZIEGLER, R.G. (1991) Vegetables, fruits, and carotenoids and the risk of cancer. American Journal of Clinical Nutrition 53:251S-259S.