Modern poultry farming is characterized by the confinement of large numbers of birds in controlled environments, ensuring adequate conditions for the development of the birds. However, this environment is also favorable for the development of insects such as the lesser mealworm (Alphitobius diaperinus Panzer 1797) (Coleoptera: Tenebrionidae). This insect is found worldwide, inhabiting poultry houses, congregating under feeders on the broiler litter, where early larvae and adults live and consume spilled bird feed and the bird carcasses, and in the soil, where the last instar larvae pupate (Axtell and Arends 1990, Salin et al. 2000, Lambkin et al., 2007, Japp et al. 2010). In poultry houses, larvae and adults are used by birds as an alternative food to the balanced feed, causing nutritional imbalance, influencing the development and weight gain of the birds, and causing upper digestive tract (crop and gizzard) damage (Despins and Axtell 1995, Japp 2008). In addition, this insect may have associated microorganisms, mainly bacteria pathogenic to birds and humans (Goodwin and Waltman 1996, Chernaki-Leffer et al. 2010, Hazeleger et al. 2008, Agabou and Alloui 2010, Alborzi and Rahbar 2012).
From the economic point of view, a study pointed to losses of US$3 million in broiler production in the state of Virginia, United States, which was attributed to this insect, only in relation to structural damage (Turner 1986). Due to economic losses and effects on bird health, it is important to control this insect. Currently, insecticides are used to control lesser mealworm (notably cypermethrin), but these compounds contaminate the environment and pose health risks to the birds (Umesh et al. 2004) and worker. Pyrethroid insecticides have been used for a long time in poultry houses; however, reports of inefficacy (associated with the development of population resistance to the active ingredients) and of the impaired development of birds exposed to the products have increased (Garg et al. 2004, Lambkin and Rice 2006, Lambkin and Furlon 2011).
Furthermore, pyrethroids, despite being safe to humans, are irritating and toxic for nontarget animals, including fish and aquatic crustaceans, bees, and other natural enemies, due to the high lethal dose required (Santos et al. 2007). Alternative methods for insect control have been sought since the early 1990s and include entomopathogenic fungi and nematodes (Geden and Stainkraus 2003, Rohde et al. 2006, Alves et al. 2012a, 2015), as well as insecticidal plants (Marcomini et al. 2009, Szczepanik et al. 2012, Alves et al. 2012b). Another alternative is physical control with the use of inert powders; diatomaceous earth (DE) presents great viability for the management of populations of the lesser mealworm (Lambkin 2001, Alves et al. 2006) and has been shown effective in controlling the pests of grains stored long term (Korunic 1998). In addition to showing that DE is nontoxic to birds (Japp 2008), laboratory studies have shown the effects of environmental factors (such temperature and humidity), origins of extraction, and physical and morphological characteristics (frustule morphology, granulometry) on DE efficacy in A. diaperinus control (Alves et al. 2008, Japp 2008). Regarding toxicity to humans, Nastrass et al. (2015) evaluated DE samples from Chile, China, Spain, and Mexico for cytotoxic and hemolytic actions in vitro assays. According to the authors, the DE was shown to be safe in the samples evaluated because silica content alone is not a sufficient indicator of the toxicity potential.
Despite the common occurrence of lesser mealworm as a pest in poultry houses worldwide, field studies using DE in the control of this insect are scarce and only one study in broiler houses in Australia is found in the literature (Lambkin et al. 2012).This lack of field studies with DE leads to a lack of efficacy data and to a lack of support for the performance of new studies in poultry houses, since many aspects are still unknown in these environments.
Therefore, this paper reports on this field study and is the first published account of a field assessment with DE to control A. diaperinus in broiler houses in Brazil. The experiments were conducted in two stages with the objectives 1) to determine the concentration to be applied, site and method of application, strategy of use, and sampling method of insects and 2) to define the strategies for use of the product in the control of the lesser mealworm.
Materials and Methods
All experiments were conducted in commercial broiler houses in Cascavel, Paraná, Brazil (24°20′54″S, 53°17′58″W), with an area of 1,200 m2 (100 m × 12 m [long by wide]). The floor was made of compacted soil, and the broiler litter was wood chips. The feed and water supply system was automated. During the experimental period, the management of the poultry houses consisted of housing the birds for 45–50 d, followed by a period between lots of 7–10 d for cleaning and mixing of the broiler litter. No chemical insecticide treatment was applied for pest control. The poultry houses were selected based on their history of high population by the lesser mealworm and were between the second and sixth lots of broiler litter reuse.
In all experiments, the product Poultry Sec (Vet Science Nutracêuticos Ltda., Maringá, PR, Brazil) was used, which according to the manufacturer’s information, is composed of amorphous silica dioxide (86%) obtained from mineral deposits in Northeast Brazil and has a mean particle size of 3–50 µm. Considering the nonuniform distribution of the insects in the poultry house (Uemura et al. 2008), the study was carried out in two phases (Fig. 1), with four experiments being carried out in the first stage (Preliminary stage) (Table 1), and four additional experiments in the second stage (Real-scale stage), planned based on the preliminary results and using separate poultry houses as treatment and control (Table 2).
Preliminary Stage
Experiment 1
The poultry house at the fourth housing lot was divided longitudinally in half, with DE being applied to the treatment area. In one of the halves, along the lateral lines of feeders, the DE was manually applied to the surface of the broiler litter at a concentration equivalent to 170 g/m2 . In the adjacent half (Control), no product was applied (Fig. 1). For the assessments, the poultry house was divided into three regions: entrance, middle, and exit of the poultry house; in each region, six samples of 200 ml of broiler litter were collected along both lines of feeders (Treated and Control) at equidistant points (5 m). The collected samples were stored at −20°C for 24 h, for later counting of the insects, when the larvae and adults were separated. The assessment procedure was repeated after 7 and 14 d. The experimental design was randomized into three blocks (entrance, middle, and exit) with two plots each. The data were analyzed in pairs using the Wilcoxon test (P < 0.05).
Experiment 2
The poultry house at the sixth housing lot received the DE application (in the same concentration as above) only in the poultry housing area (Fig. 1). Three areas were delimited for treatment, and other identical areas were not treated (Control). Prior to application, and at 14 and 21 d, 200-ml samples of the broiler litter under the feeders were collected, totaling six equidistant collection points in each area. The design and analysis of the data were as described for the previous experiment.
Experiment 3
In the poultry house with the second lot of birds, the region corresponding to the poultry housing area was divided longitudinally in the middle (Fig. 1). In one half, the DE was applied at a concentration of 170 g/m2 only in the bird housing area, where it was applied uniformly with a 2-mm mesh sieve. The product was then incorporated into the broiler litter with a rotating disc used for broiler litter mixing. The other half did not receive the DE treatment (Control). The assessment procedure was repeated weekly during the whole housing period (42 d), which consisted of sampling 200 ml of the broiler litter under the central feeder, between the pillars at 11 collection points in each area and in a completely randomized design. Data were also analyzed in pairs by the Wilcoxon test (P < 0.05).
Experiment 4
The poultry house for the third lot of birds, with all procedures described above being followed, was divided in half, with DE being applied to the treatment area at a concentration of 250 g/m2 and in a concentrated manner next to the pillars (100 g/pillar) and walls (80 g/linear m) in the referred area (Fig. 1). A corresponding area did not receive the DE treatment (Control). After the application, the assessment and data analysis procedures used in experiment 3 were repeated weekly and throughout the housing period of the birds (42 d), both in the Treated area and in the Control area.
Based on the results obtained in the preliminary stage, new experiments were conducted under field conditions. Separate poultry houses were used for the assessment (Treated and Control), in the evaluation stage herein called real-scale stage, with the population of insects being compared over time (pretreatment and post-treatment between poultry houses and within the same poultry house).
Real-Scale Stage
Experiment 5
Two poultry houses were selected. Both were in the first lot of birds, one of them was subjected to DE treatment, and the Control poultry house received no treatment. The DE was applied homogeneously at a concentration of 280 g/m2 throughout the entire poultry house, covering the broiler litter surface and being stirred for incorporation of the DE (Fig. 1). Prior to the DE application and in the last week of housing of the birds (45 d), the methodology of Godinho and Alves (2009) was adopted, with 12 broiler litter samples being collected from the anterior, middle, and final thirds of the poultry house, near the pillars, wall, and feeder. Samples were taken in an area of 30 × 30 cm at full depth down to the floor. The same assessment procedures were performed on the same days in the Control poultry house. In the comparison of the poultry houses, the data obtained in the preand post-assessments were statistically analyzed by the Wilcoxon test (P <0.05), and the Mann–Whitney test (P <0.05) was used for comparisons among the poultry houses. In all cases, a completely randomized design was adopted. In addition, the re-infestation rate was calculated, with the total number of insects collected pre-application (corresponding to 100%) being compared with the number of insects found post-application. Treatment efficacy was determined using the Henderson and Tilton (1955) formula.
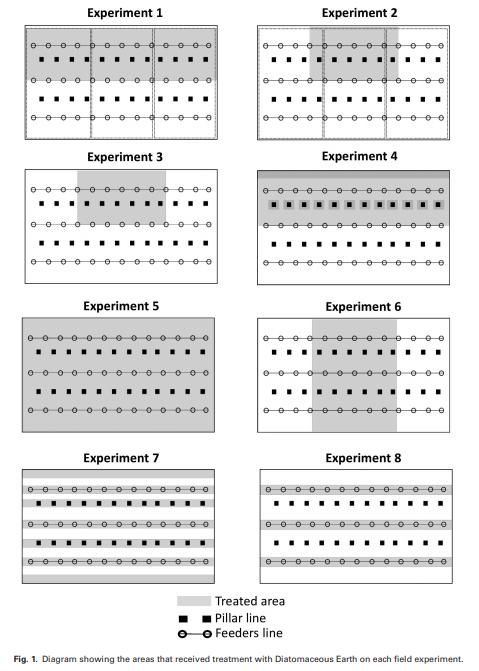
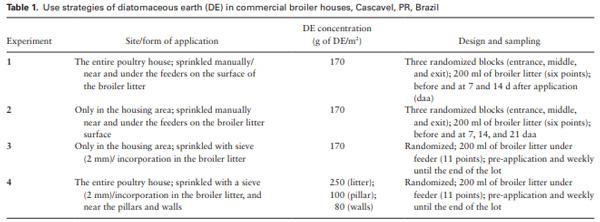
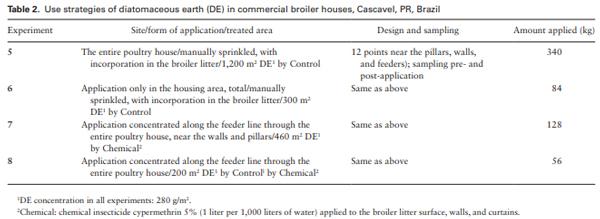
To reduce the amount of product to be applied (and consequently the cost of the DE treatment), experiments 6, 7, and 8 were conducted with concentrated applications in certain areas of the poultry house (Fig. 1). Thus, in experiment 6, the application was concentrated in the housing area; in experiment 7, DE was applied to the abrangence area of the feeders, along the walls and pillars, and this house was compared to a poultry house that received chemical insecticide (cypermethrin 5%: 1 liter per 1,000 liters of water) (broiler litter surface, walls, and curtains). In experiment 8, the DE was applied to the abrangence area of the feeders and compared with a Control poultry house (without any application) and with a third poultry house that received the normal chemical treatment (cypermethrin 5%: 1 liter per 1,000 liters of water) (broiler litter surface, walls, and curtains) (Table 2). For experiments 6, 7, and 8, the same procedures as described in experiment 5 were used for the experimental design, sampling, and data analysis.
Results
Preliminary Stage
Experiment 1
The insect population in the DE-treated poultry house was twice the population size found in the Control poultry house, when evaluated pre-application (2,072 insects vs. 1,190 insects on average, respectively) (P = 0.037). After application, however, the populations were statistically equal at 7 and 14 d of evaluation due to the smaller number of insects in the treated area (Fig. 2).
Experiment 2
In the pretreatment assessment, the insect population in the poultry house was very low both in the Treated area and in the Control area (between 100 and 400 insects, respectively); in addition, these areas did not differ from each other. In the assessment at 14 d after application, the insect population increased in the Control area and in the DE-treated area, and no significant difference was observed between the two. However, after 21 d, the population was significantly lower in the Treated area, demonstrating the efficacy of the treatment (P = 0.013) (Fig. 2).
Experiment 3
The pretreatment population was significantly higher in the DE-treated area (P = 0.031). However, after application of the DE, the insect population remained similar in the entire poultry house, in most of the assessments performed. Only in the assessment at 35 d after the application did the DE-treated area show a significant reduction in the pest population (P = 0.025) (Fig. 2).
Experiment 4
For sanitary reasons, the integrating company did not allow entry into the aviary for pre-application sampling. A significant difference was verified in the insect population between the treated and untreated areas from the first assessments at 7 (P = 0.031) and 14 d (P = 0.016), being significantly lower in the DE-treated area. In addition, in the Treated area, after 7 d of application, the insect population consisted almost exclusively of adults (only one larva collected at 11 sampling points in the two areas). At the 14-d assessment, larvae were recorded only in the Control area. Despite the lack of difference in the population at 21 and 28 d between DE-treated and Control areas, after 35 d of application, unexpectedly, the population in the Treated area was higher than that found in the Control (P = 0.016). In addition, more larvae were observed in the Control area. However, in the assessment at 42 d, the population found in the DE-treated area was significantly lower than in the control area (P = 0.033) (Fig. 2). Thus, the results obtained allowed better definition of the sampling method, period and sites, strategy and form of application, concentration of the applied product, etc.
Real-Scale Stage
Experiment 5
In the Control poultry house, no significant difference existed in the adult population between the pre- and post-application assessment, unlike that observed in the DE-treated poultry house, with a significant difference (P = 0.006). In the comparison of the population size pre-application between both poultry houses, no significant difference was observed, but after treatment, the insect population in the Treated poultry house was significantly lower (P = 0.018). In regard to the re-infestation rate, a 76% reduction occurred in the Treated poultry house, with an efficacy of 75.8%, according to the Henderson and Tilton (1955) formula. A slight reduction was observed in the population size of the control treatment compared to the original population (1.7%) (Table 3).
Experiment 6
In the comparison of the population size pre- and post-application, in each poultry house, significant variation was observed for both. In the Control poultry house, the population size was significantly larger in the final assessment (P = 0.006), with a 65% increase in the number of individuals, whereas in the DE-treated poultry house, a reduction of 67% was observed in the population size compared to the initial assessment (P = 0.006), with 80.5% efficacy. In the comparison between the poultry houses, no significant difference was initially observed, but in the final assessment, the population in the Treated poultry house was significantly smaller than in the Control (P = 0.0004), so the population decrease in the Treated area (relative to the entire housing area) did not affect the treatment efficacy (Table 4).
Experiment 7
The efficacy of treatments was very similar in this assessment. In the comparison of the pre- and post-application population, in each poultry house separately, significant population reduction after treatment was observed in both evaluated poultry houses (chemical: P = 0.006, DE: P = 0.012). In addition, based on the re-infestation rate, both treatments had a noticeable effect, reducing the initial population by 80 and 89%, respectively (Table 5).
Experiment 8
In the comparison of the pre- and post-application population, only the poultry house treated with the chemical insecticide presented a significant difference (P = 0.006), being superior to the others (Control by Chemical, P = 0.002; DE by Chemical, P = 0.0002) (Table 6). After treatments, a significant population reduction was observed only in the poultry house treated with the chemical insecticide (P = 0.002). With regard to the re-infestation rate, a greater effect of the chemical insecticide was observed, which reduced the population by 91%, resulting in an efficacy rate of 93.9%. In the DE treatment, the population reduction was approximately 10%, with an efficacy rate of 32.5%. In the Control poultry house, the population increased 34% compared the previous assessment (Table 6).
Discussion
In the preliminary stage experiments, the DE showed low action on the insect, since in most assessments, regardless of the strategy used, no significant difference was observed in the insect population between the Control- and DE-treated areas. However, some factors must be considered to understand the results obtained. In experiments 2 and 3, for example, the efficacy of the product was observed only after a longer period of contact, more than 20 d after treatment, compared to experiment 1. This corroborates the results of Athanassiou et al. (2005), who performed a laboratory study of DE and Sitophilus oryzae (Coleoptera: Curculionidae) and Tribolium confusum (Coleoptera: Tenebrionidae) and demonstrated that, due to the mode of action of DE in insect death, contact time is a preponderant factor.
In the experiment 4, a significant reduction of the insect population was observed in the area treated with DE in the first two assessments. Furthermore, even after a population increase observed at 35 d after application, a significant reduction was again observed after 42 d. Such significant action was probably due to the application of DE to the total area of the poultry house and to specific points (pillars and walls), differing from other experiments and agreeing with the observations of Alves et al. (2006), who demonstrated a direct relation between the increased concentration and mortality rate when evaluating the efficacy of the DE on the lesser mealworm in the laboratory.
Thus, in accordance with the objective of the preliminary stage, the methodology for field assessments was adjusted, and in the subsequent experiments, the DE concentration (a minimum of 280 g/m2 ) to be applied was increased to standardize the sampling method following methodology adapted from Godinho and Alves (2009) and sampling time (previous and considering the entire period of the lot). Distinct poultry houses were also used to evaluate the treatment and Control, which may have influenced the results obtained in experiments 1–4, since Uemura et al. (2008) had shown earlier that the migration and dispersal of the lesser mealworms inside the poultry house is also subject to the influence of environmental factors, and Alves et al. (2008) and Oliveira et al. (2009) had shown the repellent action of DE on the lesser mealworm, emphasizing the importance of this action in the establishment of control strategies using DE.
Concerning the real-scale stage, regardless of the strategy/ area treated, the use of DE in the poultry house was effective in reducing the re-infestation by insects of the Treated poultry houses (Tables 3–6).
In experiment 5, the application of DE in a concentration higher than used in the preliminary stage (280 g/m2 ) and in the entire poultry house (340 kg in total) intensified the contact between the insects and the product, guaranteeing a significant reduction after approximately 45 d and reducing the re-infestation rate by 76%. This strategy proved to be effective, and the concentration and amount of DE used can be considered economically viable in view of a single application per broiler litter cycle. The DE presents good persistence in broiler litter, and as observed by Oliveira et al. (2009), even after 270 d, the death of lesser mealworms that had contact with broiler litter treated under laboratory conditions was observed. Under field conditions, the persistence of insecticidal action of DE is unlikely to be so prolonged; however, these results indicate that DE can respond well to the different detrimental factors in the environment.
Considering that the reduction in the amount of product applied represents not only considerable savings but also ease of application, in the subsequent experiments, we tried to evaluate the use of DE in smaller quantities and by concentrating the application in areas where insects are known to be more abundant, such as in the housing area, near the feeders, pillars, and walls (Uemura et al. 2008).
Thus, in experiment 6, notably, even with a concentration of DE four times lower than that used in experiment 5, the application of 84 kg of DE in the housing area (300 m2 ) was an effective treatment for managing the insect population, as shown by a comparison of the poultry houses and in the reduction of the re-infestation rate. In experiment 7, the application was performed by concentrating the treatment under the feeders, along the walls, and near the pillars, in the entire poultry house, totaling 128 kg of DE. In this assessment, as already mentioned, the treated areas were compared with a poultry house treated with chemical insecticide in the concentration and use strategy recommended by the manufacturer. Both treatments were effective in the reduction of the lesser mealworm population, with the DE treatment performing similarly to the chemical treatment with cypermethrin 5%. The efficacy of DE for the control of the lesser mealworm compared to the treatment of poultry broiler litter with chemical insecticide becomes even greater if the risks of using the chemicals are compared.
In experiment 8, a different efficacy was observed between the treatments with DE and chemical insecticide. The population of insects sampled in the DE-treated poultry house did not suffer a significant reduction, and the decrease in re-infestation rate was only 9%, compared to 91% of the chemical treatment. The DE use strategy tested in this experiment probably led to the low efficacy, since the concentrated application of 56 kg only along the feeder line through the poultry house (200 m2 in total) was not as effective as the applications in the total area of experiment 5; the application concentrated in the housing area of experiment 6; or the application concentrated under the feeders, along the walls, and near the pillars of experiment 7, despite the higher concentration.
In the only comparable study available in the literature, Lambkin et al. (2012) evaluated different strategies for the use of DE and chemical insecticides and obtained better results in the treatment in the total area of the poultry house with DE than with the treatment concentrated along the feeder line (≈1-m bands). Additionally, based on their results, those authors do not recommend DE to control the lesser mealworm because DE was applied in 1.4 times higher concentration than used here (400 g/m2 ). To explain the DE failure, the authors highlight that all DE used in the world did not originate from the same place, which implies variation in the DE composition.
In this sense, Japp (2008) evaluated the application of DE samples from different regions of Argentina to lesser mealworm (63 g/ m2 ) and observed mortality ranging from 7 to 98%. In addition, the author also analyzed these samples and observed large particles (500 to 3000 µm). As noted by Korunic (1998), particles up to 10 µm and higher amounts of amorphous silica are essential for high insecticidal activity because small particles can adhere more strongly to the insect surface, which is essential to DE action. The product tested in this work showed particles with approximately 120 µm (Alves et al. 2017), which explains the results obtained in this study.
In our study, the promising results obtained with the DE product in the successive experiments demonstrated the great potential of DE for use in the control of A. diaperinus, with the possibility of varying the application strategy. DE, besides not causing environmental and other damages as previously reported, has the advantage of reducing transport and handling costs, making its use feasible as a control strategy when used in a concentrated form in poultry houses.
The recommended method is its application in the total area at the beginning of the broiler litter cycle in the poultry house, at minimum concentration of 280 g/m2 . Throughout the lots, the DE could be applied by concentrating it only in the housing area or focusing under the feeders, along the walls, and near the pillars. Other forms of control could be used in combination, either with chemical insecticides, biological alternatives such as entomopathogenic fungi (Alves et al. 2015), or their combination, seeking the rational and joint use for the integrated pest management of poultry farming.
Acknowledgments
The authors are grateful to Vet Science Nutracêuticos Ltda. for providing the DE product tested in this work. The authors would also like to thank CNPq for their financial support and scholarships.
This article was originally published in Journal of Economic Entomology, 110(6), 2017, 2716–2723. doi: 10.1093/jee/tox287. © The Author(s) 2017. Published by Oxford University Press on behalf of Entomological Society of America.