Large volumes of wastewater from confined pig production are stored in anaerobic lagoons. Control methods are needed to reduce air pollution by foul odors released from these lagoons. In a pilot-scale experiment, we evaluated the effect of pig wastewater pre-treatment on reducing the concentration of selected malodor compounds in lagoons receiving liquid from: (1) flocculant enhanced solid–liquid separation (SS), and (2) solid–liquid separation plus biological N treatment using nitrification-denitrification (SS+NDN). A conventional anaerobic lagoon was included as a control. Concentrations of five selected malodorous compounds (phenol, p-cresol, 4-ethylphenol, indole, and skatole) and water quality parameters (ammonia-nitrogen and chemical oxygen demand) were determined in lagoon effluents. The SS+NDN pretreatment was more efficient than the SS in reducing odorous compounds in the lagoon liquid. The SS+NDN reduced by about 99% the liquid concentrations of all selected compounds. An odor panel test revealed that SS was ineffective to reduce the human sense of malodor with respect to the control. Whereas the SS+NDN had the significant lowest odor intensity and unpleasantness. These results are supported by the strong correlations found between the sum of odorous compound concentration with odor panel results and concentrations of both ammonium nitrogen and chemical oxygen demand in lagoon liquid samples.
Keywords: Swine; malodor; wastewater treatment; ammonium; chemical oxygen demand
1. Introduction
Anaerobic lagoons are earthen structures designed to provide long-term storage and biological treatment of livestock waste [1]. In North Carolina, with an annual production of about 9 million pigs, anaerobic lagoons are extensively used in pig manure management systems [2,3]. The use of anaerobic lagoons allows the reduction of both organic waste and land area for terminal application of liquid pig manure, and recycling of lagoon liquid for flushing manure from pig houses [1]. Although anaerobic lagoons are designed to degrade organic waste loads [4], still foul odors may be emitted [4,5]. Moreover, odor emissions beyond the boundaries of pig production operations have been associated with the decline of quality of life for rural residents in the proximity of these facilities [6,7]. An alternative to resolve this environmental problem is pre-treatment of liquid pig manure prior to lagoon input to control foul odor emissions from lagoon structures and during land application of lagoon liquid.
Although research on odor mitigation technologies has major focus in reducing air pollutant and improving indoor air quality, there are a set of technologies suitable to reduce air pollutants outside the animal housing [8]. Among these technologies, solid–liquid separation and biological N treatment (nitrification–denitrification) are two methods that were evaluated to treat liquid pig manure prior to lagoon input [9]. An on-farm solid– liquid separation system that combined mechanical and chemical (flocculant addition) processes was reported to reduce solids prior to lagoon input by 85% and decrease annual ammonia emissions by 73% as compared to an anaerobic lagoon not receiving solid– liquid pre-treated wastewater [10,11]. On the other hand, an on-farm multistage wastewater treatment system using solid–liquid separation, nitrification/denitrification (biological N), and soluble phosphorus (P) removal processes was evaluated within an agreement between the North Carolina Government and several pig farming companies, called ‘environmentally superior technology’ (EST), to replace anaerobic lagoons in North Carolina. This multistage treatment system was the only on-farm technology certified to meet the high-performance EST standards to reduce the release of ammonia, phosphorus, heavy metals, pathogens, and odors into the environment [11,12]. It was reported to perform consistently well in wastewater odor removal [13,14]. The EST evaluation also included cost analyses that supported the North Carolina Government to establish a financial assistance program to pig producers for a lagoon conversion program [12,15]. The voluntary adoption of the solid–liquid separation plus biological N technology in Chile led to the conversion of 60% of their swine lagoons (1.3 million pigs) because of its additional advantage over anaerobic digestion systems of providing carbon offsets [16].
In order to better understand how water quality was improved in lagoons receiving liquid from pre-treatment technologies, we performed a replicated 15-month pilot-scale lagoon conversion study, in which we monitored nutrients, heavy metals, and odorous compounds. From this pilot-scale study, we already reported the positive effect of (i) enhanced solid–liquid separation alone and (ii) solid–liquid separation plus biological N pretreatments on water-quality improvements, reduced N gaseous losses, and decrease in accumulation of N, P, Cu, and Zn in lagoon sludge by comparison to a conventional anaerobic pig lagoon not receiving pre-treated liquid [17,18]. Here, we report data on reduction of malodorous compounds after lagoon conversion from that same experimental lagoon study. The objective was to report the effect of pre-treatment technologies on potential odor emissions reduction from the liquid stored in already converted lagoons.
2. Materials and methods
2.1. Experimental setup
The pilot-scale column lagoon study was located in a pig finishing farm with 5145-head distributed in seven barns in Sampson Co., North Carolina, U.S.A. Liquid manure was collected under the barns using slotted floors and a pit recharge with pull plug system filled weekly with recycled lagoon effluent. The column lagoons were installed in the field, adjacent to an anaerobic lagoon retrofitted with a full-scale wastewater treatment plant that treated the entire waste stream from the pig finishing operation in a multi-step system that consisted of three process units: solid–liquid separation, biological nitrogen treatment, and wastewater disinfection/phosphorus removal [12].
Effluents taken from three different points of the fullscale plant were used to provide the pilot-scale column lagoon study with the following three treatments: (i) liquid raw manure as the influent of the conventional lagoon treatment (control); (ii) liquid from the flocculant- enhanced solid–liquid separation module using a rotary press separator as the influent of the solid separation (SS) lagoon pre-treatment; and (iii) liquid from the biological N module as the influent of the solid separation plus nitrification–denitrification lagoon pre-treatment (SS + NDN).
The study was performed in six lagoon columns with two lagoon column replicates per treatment. The lagoon columns were made of 2.1-m length clear polyvinyl chloride pipe (0.153 m i.d.) to reproduce the average depth (2.0 m) of the adjacent anaerobic lagoon. The lagoon depth was above the minimum liquid depth of 1.8 m required for design of anaerobic lagoons in North Carolina [1]. The columns were placed under a carport to simplify the water balance, eliminating the dilution effect from rainfall [3]. To verify how closely columns reproduced the typical seasonal water temperatures of the anaerobic lagoon during the course of the study, the control treatment column and the adjacent anaerobic lagoon were instrumented with temperature data loggers at 0.5 m below the liquid surface (HOBO, Onset Computer Corp., Bourne, MA). The weekly mean water temperatures of the columns (TC) during the study were highly correlated and very similar to the weekly mean water temperatures of the adjacent fullscale anaerobic lagoon (TAL) such that TC = 0.92 × TAL + 3.00 (R2 = 0.95; P < .05) [15].
Initially, the six lagoon columns had the same wastewater composition containing 14.2 L of sludge and 22.6 L of liquid obtained from the adjacent anaerobic lagoon. Thereafter, on a weekly basis, 0.42-L liquid samples were taken from lagoon columns and replaced with equivalent volumes of liquid raw manure leaving the barns (control), and pre-treated using SS or SS + NDN for 15 months (20 February 2007 to 15 May 2008). The volume of column liquid replaced weekly was estimated as the equivalent volume to replace the entire liquid volume of 24,145 m3 of a full-scale anaerobic swine lagoon receiving pre-treated water at a rate of 39 m3 d−1 [10]. The 0.42-L volume sample was taken from each lagoon column with a peristaltic pump at 0.6 m below the liquid level to reproduce liquid sampling from full-scale anaerobic lagoons [10,17]. Tap water was added weekly to each column to replenish evaporation losses. On average, the addition of tap water led to a dilution error of less than 2% of the total volume of liquid (36.8 L) in each lagoon column. According to our previous report [17], it took 11 months for water quality parameter concentrations in the lagoon to decrease and remain stable as a result of the replacement of the anaerobic lagoon liquid with SS or SS + NDN pre-treatments. Therefore, in the last four months (February–May 2008) of the water-quality evaluation, the odor study was performed using 60-mL aliquots from lagoon liquid samples taken every two weeks (seven sampling dates). These samples were analyzed to determine the difference in concentration of aromatic odor compounds in the control versus the lagoon columns receiving SS and SS + NDN pre-treated liquid.
2.2. Water-quality analytical methods
Water-quality analyses were performed according to Standard Methods for Examination of Water and Wastewater [19]. Total solids (TS), volatile solids (VS), and total suspended solids (TSS) were determined according to Methods 2540 B, D, and E, respectively. The closed reflux colorimetric method was used to determine the chemical oxygen demand (COD) (Method 5220 D). Ammonia-nitrogen (NH3-N) was determined by the automated phenate method (Method 4500-NH3 G). Total Kjeldahl N (TKN) was determined using acid digestion and the automated phenate method. The pH was determined electrometrically (Method 4500-H+ B). Column influents were analyzed monthly for all water-quality parameters while column effluents were analyzed biweekly.
2.3. Quantification of selected aromatic odor compounds
Odor compounds (phenol, p-cresol, 4-ethylphenol, indole, and skatole) were extracted from lagoon liquid samples using Twister stir bars (10-mm long × 0.5-mm phase thickness; Gerstel, Baltimore, MD, USA) with a 1- mm polydimethylsiloxane (PDMS) coating. Prior to use, the Twisters were thermally conditioned under a stream of high-purity N2 at 250°C for 60 min. Thereafter, the Twisters were placed in glass vials fitted with Teflon-lined screw caps along with 10 mL of lagoon liquid and stirred at 300 rpm for 1 h. The Twisters were then removed, rinsed with de-ionized water and blotted dry. The volatile compounds retained by the Twisters were desorbed in a Gerstel model TDSA thermal desorption unit interfaced to a Varian model 3800 gas chromatograph (GC) and Varian Star 2200 mass spectrometer (Agilent Technologies, Santa Clara, CA). After an initial time of 0.25 min, desorption of the Twisters was performed with an initial temperature of 100°C, programmed at 60°C min−1 to 260°C, and then held for 30 min. Compounds were transferred in a splitless mode to a glass wool packed injection liner maintained at −50°C with liquid CO2. Compounds were then transferred to the GC column with a 20:1 split ratio by raising the injector temperature at 12°C s−1 to 300°C. Compound separation was performed on a 30 m× 0.25 mm VF-23MS column with a film thickness of 0.25 μm (Varian, Inc.). The gas chromatograph operating conditions were: He carrier constant flow rate of 1 mL min−1 and column oven at 60°C for 1 min, programmed at 7°C min−1 to 115°C, at 1.5°C min−1 to 140°C, and then at 15°C min−1 to 195°C. The mass spectrometer was operated in an electron ionization mode with an emission current of 10 μA, a scan time of 0.35 s per scan, and a scan range of 45–225 amu. Odor compounds were quantified by a 6-point external standard calibration using standards dissolved in 10 mL of water. For each odor compound measured in the wastewater, quantitation was based on its most prominent ion, while identification was based on computer matching of spectra and retention times. Pearson correlation coefficients for each compound averaged above 0.99 while residual standard deviations (RSD) averaged less than 15% for all compounds except phenol, the RSD for which averaged 45.7%.
The overall effect of each treatment on odorous compound reduction was evaluated according to two approaches: (1) the sum of concentrations of the five selected odorous compounds and (2) the sum of odor activity values (OAVs). According to Parker et al. [20] are calculated for each individual compound as OAV = C/SCOT, where C is the concentration of the odorous compound and SCOT is the single-compound threshold value. The threshold value is the lowest concentration of a single compound in air that can be detected by the human olfactory sense when compared to a non-odorous sample.
2.4. Odor panel analysis
Samples were analyzed for both intensity and hedonic tone using a static-scale method by comparison to five standard n-butanol solutions, following the general guidelines of ASTM [21]. Solutions consisted of 0.25, 0.75, 2.25, 6.75, and 20.25 mL n-butanol L−1 in water, which matched to intensities of 1.0, 2.0, 3.0, 4.0, and 5.0, respectively. The intensity of the odor was determined by each of five panelists by comparing the full strength odorous lagoon liquid sample to the known concentrations of n-butanol mixed with water. Scores ranged from 0.5 for an odor sample weaker than the lowest n-butanol concentration to 5.5 for an odor stronger than the highest concentration, in increments of 0.5. The average intensity was calculated for the panel using the arithmetic mean. The hedonic tone was determined in a similar manner by sniffing the full-strength odor sample. Panelists were asked to subjectively assign a score for hedonic tone on a scale of −4 to + 4, with −4 being very unpleasant, 0 being neutral, and + 4 being very pleasant. The average hedonic tone was calculated for the panel using the arithmetic mean.
2.5. Statistical analysis
Summary statistics were performed using PROC MEANS procedure in SAS (Statistical Analysis System, version 9.4). Analysis of variance for the effect of pre-treatments on compositions lagoon liquid was conducted using the MIX model in SAS. Least square means were compared using the pdiff option, and were considered different when the probability values were P < .01. Significant relationships among water-quality parameters were conducted using linear regression analysis PROC REG in SAS.
3. Results and discussion
3.1. Water quality
Water-quality parameters in the pilot-scale lagoon columns were monitored during 15 months. At the onset of the experimental lagoon columns (T0), the lagoon liquid had identical concentrations for all water quality parameters (Table 1). As previously reported [15], differences in water-quality parameters among lagoon columns became statistically significant and diverged over time from their uniform concentration at the onset of the experiment. Water-quality improvement was recognized when concentrations of water-quality parameters of the treatments significantly decreased with respect to the control [10]. These significant differences in water-quality characteristics among treatments justified our work during the last four months (T12–T15) to confirm reduction in odor compound concentrations were related to improved lagoon water quality. Excluding pH, differences in the mean concentrations of TKN, NH4- N, TS, VS, TSS, and COD were significant (P < .01) between the control and each lagoon pre-treatment during the course of the four-month odor study (Table 1). In summary, improvement in TKN, NH4-N, TS, VS, TSS, and COD was moderate with SS pre-treatment but high with the SS + NDN pre-treatment. Of all these water quality parameters, NH4-N is also a contributor to lagoon odors because of ammonia volatilization [15]. In the lagoon columns that received SS and SS + NDN pretreatments, average NH4-N concentrations during the four-month odor study were 28% and 99% lower than the control, respectively (Table 1). The significant waterquality improvements with the SS + NDN pre-treatment are in agreement with the decrease in NH4-N strength by 92% in a full-scale anaerobic lagoon receiving treated liquid from a system that combined solid–liquid separation followed by biological N removal [11].
Table 1. Lagoon liquid composition at the onset of the anaerobic swine lagoon columns (T0), and during the odor study (T12–T15).
3.2. Concentration of odor compounds in the lagoon inflows
There are more than 160 different odor compounds identified in anaerobic pig lagoon liquid [22]. Although there is not a single odor compound that is a reliable indicator of offensive odor from pig manure, the five selected aromatic odor compounds (phenol, p-cresol, 4-ethylphenol, indole, and skatole) have been used in other studies as indicators of malodors from both raw pig manure and anaerobic lagoon liquid [20,23]. Previous studies reported that the use of polymer-enhanced solid–liquid separation of pig manure did not strongly affect the strength of the selected odor compounds with respect to untreated pig manure [13,14]. During our odor study, SS pre-treatment reduced the influent average concentrations of phenol, p-cresol, 4-ethylphenol, indole, and skatole by 87%, 18%, 15%, 28%, and 52%, respectively, with regard to the untreated control (Table 2). These reductions in odorous compound concentrations with the SS pre-treatment were likely related to the adsorption of odor compounds to separated solids [14,24]. However, remarkable reductions of 98–99% in concentration of the selected aromatic compounds with respect to an untreated control were reported after solid–liquid separation followed by biological nitrification–denitrification treatment in full-scale wastewater treatment systems [13,14]. According to Loughrin et al. [14], the largest reduction of malodorous compounds after nitrification–denitrification treatment was due to their utilization by the suspended denitrification bacteria as a soluble carbon source in anaerobic respiration while aerobic respiration in the nitrification was responsible for further reductions. Likewise, the SS + NDN pre-treatment influent had reduced concentrations of all five aromatic odor compounds by about 99% with respect to the influent of the control. Reductions in concentration of selected odor compounds corresponded, except for pH, with water-quality parameter concentration reductions trend of Control > SS > SS + NDN (Table 2).
Table 2. Average concentrations of selected aromatic odor compounds and water-quality parameters in the influent of the lagoon columns during the odor study (n = 3).a
3.3. Effect of influent pre-treatment on odor compound concentration in lagoon liquid
Differences in odor compound concentrations were significant among treatment combinations of this study as a result of continuous replacement of the lagoon liquid with processed pig manure using SS and SS + NDN pretreatments (Table 3). Differences in least square mean concentrations of odor compound concentrations pulled by sampling date were highly significant (P < .01) with respect to the conventional anaerobic lagoon (control) treatment (Table 3). With the exception for phenol, the lagoon with the SS pre-treatment had reductions in concentrations of p-cresol, 4-ethylphenol, indole, and skatole by 99%, 51%, 58%, and 61%, respectively. The greatest improvements in odorous compounds occurred with the SS + NDN pre-treatment with reductions with respect to the control of about 99% in concentrations of all selected compounds.
Table 3. Analysis variance for the effect of pre-treatment on the concentration of selected odor compounds in liquid of lagoon columns, and comparison of least square means across sampling dates (n = 7).
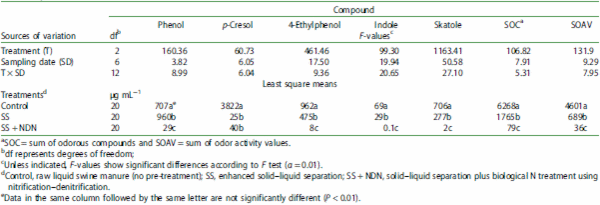
Sampling dates and the interaction with treatment (Treatment × Sampling Date) had significant effects on odorous compound concentrations assumed to be related to seasonal variation of water quality and temperatures. Seasonal variations of water temperatures were reported to affect microbial activity, evaporation losses, and magnitude of both ammonia and malodor emissions from conventional anaerobic lagoons [3,10,25]. According to Loughrin et al. [25], malodor emissions from conventional anaerobic lagoons were much higher during the cool season (February–April) than the warm season (May–June). Along with increased malodor emissions, the highest concentrations of malodor compounds in lagoon liquid were observed during the months of March and April and declined to very low levels thereafter. Therefore, our study was performed during the transition between the cool and warm weather in order to have a viable control treatment to verify differences in malodor levels of conventional lagoons versus converted lagoons. Our continuous water temperature monitoring of the lagoon columns revealed a weekly average temperature (February– May) in the range of 2.9–20.8°C, typical of anaerobic lagoons in North Carolina during the transition from cool to warm seasons [10,17]. The response pattern of each odorous compound over time for the three treatments is presented in Figure 1. The lagoon columns receiving SS pre-treatment did not consistently reduce the concentration of each odorous compound. Phenol concentrations in the SS pre-treatment were significantly higher than the control in five out of seven sampling dates. Cresol, skatole, and six sampling dates of 4-ethylphenol had significantly lower concentrations than the control while significant reductions for indole were measured only in three out of seven sampling dates. Given that adsorption to separated solids is a rather inefficient mechanism to reliably reduce all odorous compounds in wastewater treatment systems [24], lagoons storing SS pre-treated liquid could be a significant source of odors. Instead, the lagoon columns receiving the SS + NDN pre-treatment had very low and statistically different concentrations with respect to the control for each odorous compound in all sampling dates. These consistent reductions in odorous compound concentration observed in the lagoons with SS + NDN pre-treatment were the result of the effectiveness of SS + NDN to biodegrade odorous compounds from pig wastewater prior to lagoon input. Therefore, the use of SS + NDN could provide a lower potential of odor emissions from converted lagoons.
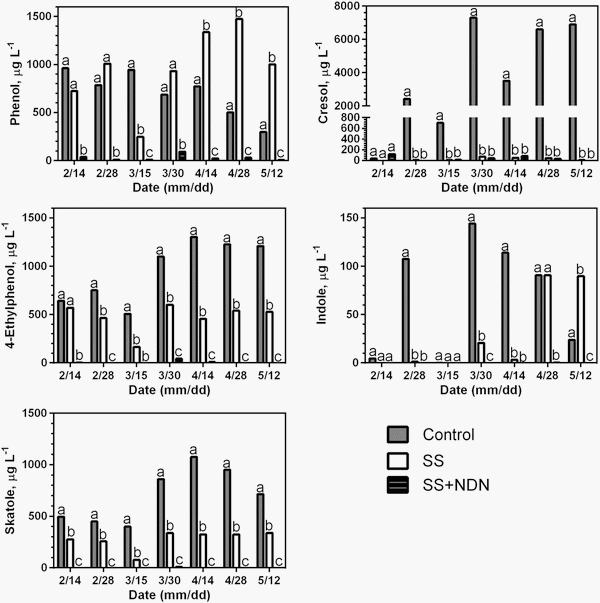
Figure 1. Odorous compound concentration in liquid of pilot lagoon columns over the seven sampling dates of the study. Each bar is the least square mean of two replicates. Control, conventional anaerobic lagoon (no pre-treatment); SS, enhanced solid–liquid separation; SS + NDN, solid–liquid separation plus biological N treatment using nitrification–denitrification. The same letter indicates that least square means are not significantly different (P < .01).
The overall effect of each pre-treatment versus the control was further evaluated for potential reduction of odor emissions according to both the sum of the five odorous concentrations (SOC) and the sum of OAV (SOAV). Differences in SOC were significant with respect to the control (Table 3). On average, the SS pre-treatment reduced the SOC by 72% while SS + NDN reduced the SOC by 99%. The same significant differences were obtained with the SOAV (Table 3). Whereas SS pre-treatment reduced the SOAV by 85%, SS + NDN pre-treatment reduced the SOAV by 99%. The OAV approach is useful to compare odor emissions by normalizing the potential impact of each odorous compound concentration in air with respect to their odor thresholds concentrations [20]. However, in our study, each OAV was calculated as the odorous compound concentration in water with respect to its threshold concentration in air. Meanwhile, each OAV in our study was not according to its strict definition, the calculated SOAV values were still highly correlated to SOC (SOAV = 0.78, SOC – 341, R2 = 0.98, n = 42, P < .01). Therefore, we chose to use the simpler SOC approach to relate them to odor panel test results and water-quality parameters.
The trained odor panel analysis of lagoon column samples presented in Figure 2 further support the significant differences in the odorous compound concentrations found among treatments. Differences between the control and the SS pre-treatment for both odor intensity and hedonic tone means were not statistically significant, indicating that the SS pre-treatment was inefficient to significantly reduce the human sense of malodor. Instead, samples from lagoon columns receiving the SS + NDN pre-treatment resulted with the lowest odor intensity and hedonic tone (Figure 2). The highly significant differences in odor compound concentrations with the SS + NDN pre-treatment versus both the SS and the untreated control are supported by the correlations of SOC with odor intensity (R2 = 0.65; P < .05) and hedonic tone (R2 = 0.60; P < .05) of the set of samples tested by the odor panel. These results confirm the potential benefit of the SS + NDN pre-treatment of lagoon influent to hugely reduce or even eliminate malodor emissions from converted lagoons.
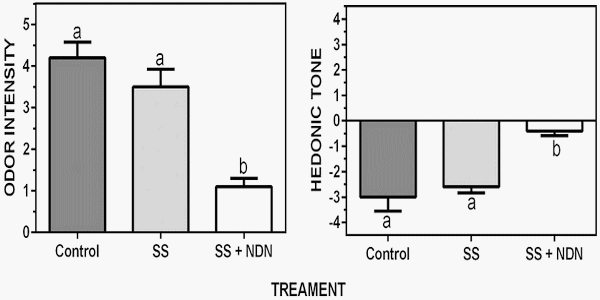
Figure 2. Odor panel assessment of the effect of pre-treatments: Control, conventional anaerobic lagoon (no pre-treatment); SS, enhanced solid–liquid separation; SS + NDN, solid–liquid separation plus biological N treatment using nitrification–denitrification. Error bars represent one standard error of the mean (n = 5), and the same letter indicates that means are not significantly different (P < .01).
3.4. Relationship between water-quality parameters and odor compound concentration
A few studies related water-quality characteristics to the odor of liquid swine waste. For instance, the logarithm of biological oxygen demand (BOD) was correlated linearly with the offensiveness of pig waste odor [26]. In another study, BOD was linearly related to total odor compound concentration in pig lagoon liquid [23]. Several other commonly used water-quality indicators, such as TSS, VS, NH4-N, COD, and alkalinity, correlated well with the concentration of odor compounds in liquid during wastewater treatment [13,14]. The correlation coefficients between four water-quality parameters (TSS, VS, NH4-N, and COD) versus individual compounds in our study are presented in Table 4. Except for phenol versus VS and indole versus TSS, the correlations coefficients were highly significant for single compounds (P < 0.01) but limited for consistently describing the pre-treatment effect on odor reductions.
For the SOC and SOAV, the four selected water quality parameters (TSS, VS, NH4-N, and COD) were found to be significantly related to reductions in malodor (Table 4). The relationships of SOC with each of these four water-quality parameters are summarized by treatment in Figure 3. Of the four water-quality parameters, COD distinctly described the relationship between water-quality improvements and SOC for the control and the pre-treatments with the highest regression coefficient (R2 = 0.70). As a result of the SS and SS + NDN pre-treatments, COD decreased markedly in the lagoon liquid effluent as compared to the control. While the use of water-quality parameters as a measure of odor reduction indicators is empirical, our results indicate that commonly measured water-quality parameters, such as NH4-N and COD, can be used in the absence of appropriate analytical methods for semiquantitative evaluations of odor control measures such as the SS and the SS + NDN pre-treatments tested in our odor study.
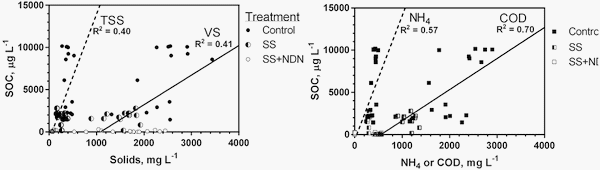
Figure 3. Linear regressions of total suspended solids (TSS), volatile solids (VS), ammonium-N (NH4), and chemical oxygen demand (COD) versus sum of the concentrations of odorous compounds(SOC) in water samples from lagoon columns receiving pre-treated liquid manure. Control, raw liquid swine manure (no pre-treatment); SS, enhanced solid–liquid separation; SS + NDN, solid–liquid separation plus biological N treatment using nitrification–denitrification.
Table 4. Linear correlation coefficients between concentrations of water-quality parameters and selected odor compounds identified in the liquid of the experimental lagoon columns.
4. Conclusions
Results on water-quality changes and odorous compound concentration of our column study showed that both SS and SS + NDN pre-treatments significantly reduced malodor concentrations in lagoon liquid with respect to the conventional lagoon storage used as a control treatment. However, the SS and SS + NDN pretreatments differed in their efficiencies to reduce inflow concentrations of phenol, p-cresol, 4-ethylphenol, indole, and skatole. These differences in efficiencies were confirmed by the correlation of SOC to odor panel analysis tests results and water-quality parameters concentrations (NH4-N and COD) in liquid sampled from the columns. The odor panel analysis revealed that differences between the control and the SS pre-treatment on both odor intensity and hedonic tone means were not statistically significant, indicating that the SS pre-treatment was inefficient to significantly reduce the human sense of malodor. The SS + NDN pre-treatment had the greatest improvements in reducing by about 99% the concentrations of all selected compounds in liquid stored in the lagoon that resulted with the lowest odor intensity and hedonic tone. These results support the use of pre-treatment technologies such as SS + NDN to simultaneously reduce malodor compound concentrations and nutrients such as NH4-N from liquid stored in lagoon structures. Ultimately, the SS + NDN pre-treatment would eliminate associated ammonia and malodor emissions during both storage and land application of lagoon liquid.
Acknowledgements
This research was part of USDA-ARS National Program 212: Soil and Air; ARS Project 6082-12630-001-00D ‘Improvement of Soil Management Practices and Manure Treatment/Handling Systems of the Southern Coastal Plain’. Mention of trade names or commercial products in this article is solely for the purpose of providing specific information and does not imply recommendation or endorsement by the U.S. Department of Agriculture.
Disclosure statement
No potential conflict of interest was reported by the authors.
This article was originally published in Environmental Technology, 2017. https://doi.org/10.1080/09593330.2017.1363294. This work was authored as part of the Contributor's official duties as an Employee of the United States Government and is, therefore, a work of the United States Government. In accordance with 17 USC. 105, no copyright protection is available for such works under US Law.
References
[1] Barker JC. Lagoon design and management for livestock waste treatment and storage. North Carolina Coperative Extension Service; 1996.
[2] North Carolina Agricultural Statistics. Section 3: Livestock, dairy & poultry; 2014. Available from: https://www.nass. usda.gov/Statistics_by_State/North_Carolina/Publications/ Annual_Statistical_Bulletin/AgStat/Section04.pdf.
[3] Westerman P, Arogo Ogejo J, Grabow GL. Swine anaerobic lagoon nutrient concentration variation with season, lagoon level, and rainfall. Appl Eng Agric. 2010;26:147–152.
[4] Tyson T. Planning and managing lagoons for swine waste treatment. Alabama Cooperative Extension System, ANR- 9731992.
[5] Zhang XL, Yan S, Tyagi RD, et al. Odor control in lagoons. J Environ Manage. 2013;124:62–71.
[6] Wing S, Horton R, Marshall S, et al. Air pollution and odor in communities near industrial swine operations. Environ Health Perspec. 2008;116:1362–1368.
[7] Schiffman S, Williams C. Science of odor as a potential health issue. J Environ Qual. 2005;34:129–138.
[8] Ni JQ. Research and demonstration to improve air quality for the US animal feeding operations in the 21st century – A critical review. Environ Pollut. 2015; 200:105–119.
[9] Szogi AA, Vanotti MB, Ro KS. Methods for treatment of animal manures to reduce nutrient pollution prior to soil application. Curr Pollution Rep. 2015;1:47–56.
[10] Szogi A, Vanotti M. Abatement of ammonia emissions from swine lagoons using polymer-enhanced solidliquid separation. Appl Eng Agric. 2007;23:837–845.
[11] Vanotti M, Szogi A. Water quality improvements of wastewater from confined animal feeding operations after advanced treatment. J Environ Qual. 2008;37:S- 86–S-96.
[12] Vanotti MB, Szogi AA, Millner PD, et al. Development of a second-generation environmentally superior technology for treatment of swine manure in the USA. Bioresour Technol. 2009;100:5406–5416.
[13] Loughrin JH, Szogi AA, Vanotti MB. Reduction of malodorous compounds from liquid swine manure by a multi-stage treatment system. Appl Eng Agric. 2006; 22:867–873.
[14] Loughrin JH, Vanotti MB, Szogi AA, et al. Evaluation of second-generation multistage wastewater treatment system for the removal of malodors from liquid swine waste. J Environ Qual. 2009;38:1739–1748.
[15] Williams C. Development of environmentally superior technologies in the US and policy. Bioresour Technol. 2009;100:5512–5518.
[16] Vanotti M, Szogi AA, Vives CA. Greenhouse gas emission reduction and environmental quality improvement from implementation of aerobic waste treatment systems in swine farms. Waste Manage 2008;28:759–766.
[17] Szogi AA, Vanotti MB. Water quality and nitrogen mass loss from anaerobic lagoon columns receiving pretreated influent. J Environ Qual. 2014;43:1219–1226.
[18] Szogi AA, Vanotti MB. Decline in phosphorus, copper, and zinc in anaerobic swine lagoon columns receiving pretreated influent. Scientia Agricola. 2016;73:417–423.
[19] APHA. Standard methods for examination of water and wastewater. 20th ed. Washington (DC): American Public Health Association; 1998.
[20] Parker DB, Koziel JA, Cai L, et al. Odor and odorous chemical emissions from animal buildings: part 6. Odor activity value. Trans ASABE. 2012;55:2357–2368.
[21] ASTM. E544-99: standard practices for referencing suprathreshold odor intensity: procedure B, static-scale method. Philadelphia (PA): American Society of Testing Materials; 2001.
[22] Schiffman SS, Bennett JL, Raymer JH. Quantification of odors and odorants from swine operations in North Carolina. Agric Forest Meteorol. 2001;108:213–240.
[23] Loughrin JH, Szogi AA, Vanotti MB. Reduction of malodrous compounds from treated swine anaerobic lagoons. J Environ Qual. 2006;35:194–199.
[24] Whitherspoon J, Desing W, Tata P. Unit processes and emissions: an overview. In: Tata P, Whitherspoon J, Lue-Hing C, editors. VOC emissions from wastewater treatment plants. Boca Raton (FL): Lewis Publishers; 2003. p. 73–95.
[25] Loughrin JH, Quintanar AI, Cook KL, et al. Seasonal variation in heat fluxes, predicted emissions of malodorants, and wastewater quality of an anaerobic swine waste lagoon. Water Air Soil Pollut. 2012;223:3611–3618.
[26] Williams AG. Indicators of piggery slurry odour offensiveness. Agric Wastes. 1984;10:15–36.