Introduction
Swine dysentery (SD) typically manifests as a severe mucohemorrhagic colitis arising from infection of the caecum and colon of grower-finisher pigs with the anaerobic intestinal spirochete Brachyspira hyodysenteriae [1]. SD can severely depress feed conversion efficiency, may require considerable antimicrobial use for its control, and represents an animal welfare issue. Strains of B. hyodysenteriae that are resistant to antimicrobial agents increasing have been recorded, particularly in Europe. This classical form of SD re-emerged as a problem in North America towards the end of the first decade of the 21st century, after being largely absent for 10–15 years, whilst the disease has remained endemic in most other pig producing regions of the world.
Until recently B. hyodysenteriae was believed to be the only causative agent of SD; however, since around 2007 outbreaks of bloody diarrhea indistinguishable from SD have been documented in Canada and the USA in herds where B. hyodysenteriae could not be identified. Investigation of these cases led to the recognition of a novel, strongly beta-hemolytic Brachyspira species that recently has been named Brachyspira hampsonii [2,3]. Experimental inoculations of pigs have established the pathogenic potential of this new species [4–6]. Concurrently, cases of SD caused by B. hampsoniiz were recorded in pigs in Europe, and strains of the species were isolated from migratory water birds in Canada and in Spain [7–10]. A distinct strongly beta-hemolytic species named Brachyspira suanatina that causes an SD-like disease in pigs also has been identified in feral water birds and pigs in Scandinavia [11,12]. In the same time frame interest has increased in strains of B. hyodysenteriae that may have reduced capacity to colonize and cause disease, since strains of this sort can complicate the diagnosis and control of SD [13,14]. In Australia, B. hyodysenteriae has been isolated from apparently healthy herds that gave what were initially thought to be false positive results in a serological ELISA [15].
The re-emergence of Brachyspira spp., including antimicrobial resistant strains of B. hyodysenteriae and novel species like B. hampsoniiz as pathogens, has re-ignited significant concerns for pork-producers worldwide. The aims of this project were to determine whether novel pathogenic Brachyspira species were present but unrecognized in Australian pig herds; to investigate the distribution, diversity, antimicrobial susceptibility and virulence-associated plasmid gene content of contemporary isolates of B. hyodysenteriae from Australian herds, including those with clinical SD, no disease or with uncertain health status; and to compare these attributes with those for Australian isolates recovered in earlier decades.
Materials and Methods
Permissions
This study was conducted with the approval of the Murdoch University Animal Ethics Committee under permit number R2292.
Sample collection
During the period June 2014 to June 2016 a total of 1103 diagnostic samples, comprising 606 faecal and 497 colon samples that had been collected from grower/finisher pigs in 97 pig herds in Australia were submitted for testing by consulting pig veterinarians and through veterinary diagnostic laboratories. Twenty-four of these herds were considered by their veterinarians to be free of SD, and for this study had additional samples taken at the abattoir during routine screening, including samples of the colonic mucosa and faeces from pigs that did not show clinical signs. The other samples were from pigs in 25 herds where SD was believed likely to be present based on past history or the clinical signs in the pigs sampled, and from 48 herds where the health status was unknown, but where diarrhea and/or mild colitis had been identified and/or the veterinarian wanted to exclude the possibility of SD. The 97 herds were located in five Australian States: Victoria (n = 45), South Australia (n = 21), Western Australia (n = 13), Queensland (n = 11), and New South Wales (n = 7).
Historic isolates
In addition to the contemporary field isolates obtained in the study, 53 Australian isolates of B. hyodysenteriae collected in the 1980s through the early 2000s were obtained from the culture collection at the Reference Centre for Intestinal Spirochaetes at Murdoch University, based on their availability. All had been recovered from diagnostic samples submitted from herds with suspected SD. The isolates comprised 21 from the 1980s, 13 from the 1990s, and 19 from the first decade of the 2000s. They had previously been typed by MLST, and in this study they were subjected to the same analyses for the plasmid-encoded genes as were the isolates from 2014/16.
Culture and PCR
The diagnostic samples were swabbed onto selective Trypticase Soy agar (BBL) plates (TSA) containing 5% (vol/vol) defibrinated ovine blood, 400 μg of spectinomycin per ml, and 25 μg each of colistin and vancomycin (Sigma–Aldrich) per ml, and were cultured for 5 to 7 days at 37°C in a culture jar with an anaerobic atmosphere generated by an AnaeroGen™ 2.5L Sachet (Oxoid). Zones of haemolysis around the inoculated area indicated growth, and confirmation was obtained that spirochaetes were present by resuspending surface growth in phosphate buffered saline (PBS) and viewing with a phase-contrast microscope. Samples that contained spirochetes were tested concurrently by previously described polymerase chain reactions (PCRs) for B. hyodysenteriae, Brachyspira pilosicoli [16], B. hampsoniiz [4], Brachyspira intermedia and a genus-specific PCR for Brachyspira spp. [17], as well as by a nox-RFLP assay for B. suanatina [18]. New PCR tests for B. hampsoniiz and B. suanatina were developed for use in this study incorporating unique primers targeting the hemolysin A gene (tlyA) and DNAdependent RNA polymerase gene (rpoC),respectively. The primers for the new B. hampsoniiz PCR were Bham-tlyA-F26 (5’ GTAAAGGCTATACAGAAAGCAGATC 3’) and BhamtlyA-R376 (5’ CTATGGATATTACTCTATTATCATTA 3’), which anneal to position 26 to 50 and position 351 to 376 of the tlyA gene, respectively, giving a product of 351 bp. The primers for the B. suanatina PCR were Bsua-rpoC-F409 (5’ GATCCAGGCGATAGTTCTTATAC 3’) and Bsua-rpoC-R749 (5’ GTCGCAAATCTTCCGCCGTC 3’), and these anneal to the RNA polymerase gene (rpoC) at position 409 to 431 and position 730 to 749, respectively. The PCR product was 341 bp. The specificity of all the PCR assays were confirmed using genomic DNA from 282 Brachyspira strains from seven species or proposed species, obtained from the culture collection at the Reference Centre for Intestinal Spirochaetes at Murdoch University. These included strains of B. hyodysenteriae (n = 81), B. pilosicoli (n = 107), B. intermedia (n = 48), B. innocens (n = 22), B. murdochii (n = 20), B. hampsoniiz (n = 3) and B. suanatina (n = 2). PCR assays were applied to growth harvested from the primary isolation plates and resuspended in sterile water. The PCR assays were performed in 25 μl reactions consisting of 1x PCR buffer, 1.5 mM MgCl2, 0.5 U Taq DNA polymerase, 0.2 mM of each dNTP and 0.5 μM of forward and reverse primers. Cycling conditions involved an initial denaturation at 94°C for 5 min, followed by 30 cycles of denaturation at 94°C for 30 s, annealing at 58°C for 15 s and primer extension at 72°C for 1 min. The products were separated by gel electrophoresis and visualised over UV light after staining with ethidium bromide.
Multilocus sequence typing (MLST) of B. hyodysenteriae isolates
Ninety-six B. hyodysenteriae isolates obtained in 2014/16 in the current study were typed by MLST, and MLST data for the other 53 Australian B. hyodysenteriae isolates that had been recovered from the 1980s to the early 2000s were downloaded from the PubMLST site (http:// pubmlst.org/Brachyspira/). The isolates were cultured on fresh TSA plates at 37°C for 5 days, harvested, counted and resuspended with sterile PBS as described above. High molecular weight DNA was extracted using the DNeasy Blood and Tissue Kit (Qiagen) according to the manufacturer’s instructions. Cells from a 10 ml sample of a 108 cells per ml culture of Brachyspira were harvested by centrifugation at 5,000 g. The cell pellet was resuspended in 180 μl of lysis buffer containing 20 μl of proteinase K (10 mg/ml) and incubated at 55°C for 30 min. After lysis, 180 μl of AL Buffer was added and the sample incubated at 70°C for 10 min. Two hundred μl of absolute ethanol was immediately added to the sample and this was transferred to a DNeasy column. Column wash buffers AW1 and AW2 were added sequentially to the columns and centrifuged at 6,000 g. The flow-through was discarded, and the DNA was eluted with elution buffer and stored at -20°C.
MLST was conducted as previously described [19]. PCR assays were performed in 50 μl reactions consisting of 1x PCR buffer, 1.5 mM MgSO4, 0.5 U HotStar HiFidelity DNA polymerase (Qiagen), 0.2 mM of each dNTP and 0.5 μM of forward and reverse primers. Cycling conditions involved an initial enzyme activation step at 95°C for 15 min, followed by 35 cycles of denaturation at 94°C for 30 s, annealing at 50°C for 15 s and primer extension at 72°C for 1 min. The products were separated by gel electrophoresis and visualised after staining with ethidium bromide. PCR products used for sequencing were purified with the UltraClean™ PCR Clean-up Kit (Mo Bio Laboratories) according to the manufacturer’s instructions. The purified PCR products were used for sequencing, with reactions performed in 10 μl volumes using the BigDye1 Terminator v3.1 Cycle Sequencing Kit (Life Technologies) according to the manufacturer’s instructions. An annealing temperature of 50°C was used. The sequencing products were analysed using the Applied Biosystems 3730XL DNA analyser.
The raw sequences were edited and analysed using Geneious version 7.1.7 (Biomatters Ltd). For each locus the consensus sequences were aligned with the B. hyodysenteriae strain WA1 sequence downloaded from the PubMLST database (www.pubmlst.org) and the aligned loci sequences were trimmed for subsequent MLST analysis, as previously reported [19]. Allele designations for each locus were obtained by a query search from the PubMLST website. New sequence types (STs) were deposited at the PubMLST site.
MLST dendograms were constructed from the data matrix of allelic mismatches using the UPGMA (unweighted-pair group method with allelic arithmetic means) method with 1000 bootstrap replicates using the START2 program [20], and Minimum Spanning Trees (MST) were constructed using the PHYLOViZ software [21]. Consensus sequences were used for the MLST from combined individual distance matrices of nucleotide sequences from seven genes adh, alp, est, gdh, glpK, pgm and thi. Clonal complexes (Cc) amongst the isolates were identified by the BURST alogorithm using the eBURST v3 program [22]. Color-coding of STs in the various MSTs was added to distinguish reported health status of the 2014/16 isolates, to compare plasmid gene content, to highlight the decade of isolation, or to show the relationships of isolates from different countries.
Testing B. hyodysenteriae isolates for plasmid genes
High molecular weight DNA was extracted from the 96 B. hyodysenteriae isolates from the field study and 53 historical isolates, as described above. PCR tests were undertaken targeting members of a block of six genes on the 36 kb plasmid of B. hyodysenteriae [23] that recently have been described as possible virulence-associated genes involved in colonization by B. hyodysenteriae [24]. These have been designated as open reading frames (orfs) 11, 12, 13, 14, 15 and 16, respectively. For each orf, three primer pairs were designed for PCR amplification, as previously described [24]. Two PCRs also were conducted to detect the presence of the whole 36 kb plasmid, as previously described [24]. PCR assays were performed in 25 μl reactions, as described previously, with the exception that the annealing temperature used for each primer was set at 5°C less than the optimal annealing temperature to allow for a moderate stringency reaction. Amplification products were electrophoresed through an agarose gel, stained with ethidium bromide and viewed over ultraviolet light.
Each of the six orfs was recorded as being either absent (N) or present (P), and the combination was used to define a “plasmid type”, with numbers designated 1 through 7: 1 = N, N, N, N, N, N; 2 = N, N, N, N, N, P; 3 = N, N, P, N, P, P; 4 = N, N, P, P, P, P; 5 = N, P, P, N, P, P; 6 = P, P, P, N, P, P; and 7 = P, P, P, P, P, P. Assuming no other genomic differences, an isolate with a plasmid type 1, without any of the block of plasmid genes, would be predicted to be less capable of colonizing than one with a plasmid type 7, having all six of the genes. Statistical comparisons between the distribution of isolates with plasmid types 1 and 7 were made using Fisher’s exact test in GraphPad Instat. Pairwise comparisons were made between the three reported herd health statuses for the 2014/16 isolates, and between the isolates from the four decades originating from the 1980s to 2014/16. Comparisons for other plasmid types were not made because the relative significance of the presence/absence of individual genes in the block remains unclear, and there were too few occurrences of these intermediate scores to make statistical analysis meaningful.
Antimicrobial susceptibility testing
The B. hyodysenteriae isolates to be tested for antimicrobial susceptibility were subcultured to ensure purity and then grown on fresh TSA plates at 37°C for 5 days, as described above. The cells were harvested from the agar plate by resuspending the surface growth with 2 ml sterile PBS, transferring this into a microfuge tube and centrifuging the tube at 5,000 g. The pellet was resuspended in sterile PBS, the cells counted using a hemocytometer and then diluted to a density of 106 cells per ml.
Antimicrobial susceptibility of the isolates from 2014/16 was assessed using the agar-dilution method. The test plates consisted of TSA containing 5% defibrinated ovine blood and the appropriate antibiotic concentration. Control plates did not include antibiotics. The plates were incubated for 5days at 37°C in anaerobic jars and then observed for hemolysis. The isolates were tested for susceptibility to varying concentrations of lincomycin (2, 4, 16, 36, and 72 μg/ml), tylosin (1, 5, 25, 50 and 100 μg/ml) and tiamulin (0.25, 0.5, 1, 4 and 8 μg/ml). These antimicrobials and dilutions were selected to replicate earlier studies to allow comparisons of results to be made over time.
A total of 105 cells were drop-inoculated onto the control and sensitivity plates. Each isolate was tested in duplicate and B. hyodysenteriae control strain WA1 was included in each batch of tests. Growth of the strains on the control and sensitivity plates was checked visually after 5 days incubation. Zones of hemolysis present around the growth on the control plates were determined, and isolates were recorded as being susceptible to the antimicrobial concentration in the test plates if no such zones were observed. Surface growth was scraped off the plate and examined under a phase contrast microscope to confirm purity and the endpoint. The first sensitive colony zone and the last resistant colonies were checked for spirochete growth by phase-contrast microscopy. The minimum inhibiting concentration (MIC) of the antimicrobial was reported as the lowest concentration of that inhibited growth. MIC breakpoints used to assist interpretation of the results are presented in Table 1. Results were compared with those reported for Australian isolates for 2002–2006 and 2006–2007 that previously had been tested using the same methodology [25].
Table 1. MIC breakpoints (μg/ml) for in-vitro antimicrobial susceptibility tests performed on Brachyspira hyodysenteriae isolates
Results
PCR assay results
The established and the two newly developed PCRs targeting the new species were shown to amplify the gene from the target species when tested on the collection of 282 Brachyspira species and strains, and did not show any cross-reactivity. The new PCR described here for B. hampsoniiz gave stronger bands with members of this species than did the previously described PCR for this species, whilst the PCR for B. suanatina was quicker and easier to perform than the nox-RFLP assay.
Detection of Brachyspira species
Neither B. hampsoniiz nor B. suanatina were detected in any of the samples tested from the 97 herds, using culture and the previously described and the newly developed PCR methods. B. hyodysenteriae was detected in 159 samples (14.4%) from 34 herds (35.1%), and 96 isolates successfully were obtained in pure culture from 30 of these herds (Table 2). In the cases where B. hyodysenteriae isolates were unable to be recovered for further study from positive plates, this was due to multiple Brachyspira species being present and/or overgrowth with contaminant microorganisms. Two B. hyodysenteriae isolates, one each from herd 28 (ST151) and herd 86 (ST161) respectively, were weakly beta-hemolytic, and both herds were considered to be free of SD. Three unconnected herds that were colonized with B. hyodysenteriae supplied stock to other production herds. Of the original 34 herds identified as being colonized by B. hyodysenteriae, 14 of 24 (58.3%) were identified through heightened abattoir surveillance of apparently healthy herds, 12 of 25 (48%) were already suspected to have SD based on clinical signs or past history, and eight of 48 (33.3%) were of uncertain health status. Isolates were not obtained in pure culture from one of the herds that was identified as infected through abattoir surveillance, one considered to have SD, and two which were originally of uncertain health status.
In addition to B. hyodysenteriae, the potential pathogen B. pilosicoli was identified in 127 samples (11.5%) from 39 (40.2%) herds; and B. intermedia in 98 (8.5%) samples from 37 (38.1%) herds. There were 27 (2.4%) samples that were positive for Brachyspira spp. but negative for the species-specific PCRs from 17 (17.5%) herds, and these were presumed to contain B. innocens or B. murdochii. Sixty-seven individual samples collected from 28 herds contained more than one of the above named Brachyspira species, with 57 samples containing two species and ten samples containing all three species.
Multilocus sequence typing of B. hyodysenteriae
The 96 B. hyodysenteriae isolates from 2014/16 belonged to 30 sequence types (STs) (Table 2). Eleven STs contained multiple isolates, and seven of these were identified in more than one herd. The 39 isolates from 13 herds originally considered not to have SD were divided into 12 STs; of these, ST50 was found in two herds and ST150 in five of the 13 herds. One of the latter five was a breeding herd, and the other four herds with this ST are likely to have received pigs from it. This ST also was found in one herd with SD and one of the herds of uncertain health status, making it the most common ST found. The 35 isolates from the 11 herds that originally were believed to have SD belonged to 17 STs, whilst the 22 isolates from the six herds of uncertain SD-status belonged to 8 STs. STs 140, 144 and 150 were found in pigs from all three categories of health status, whilst STs 49 and 143 each were found in two health categories. The other STs each were only found in herds of a single health category. STs 31, 49 and 50 previously have been described in Australian herds sampled in earlier decades, and these were represented amongst the older isolates included in this study. The historic isolate and contemporary isolate in ST31 both came from Western Australia (WA); those in ST49 all came from herds in SA; and those in ST50 came from Queensland, Victoria and Western Australia
Table 2. Comparison of sequence type (ST), plasmid gene profile (PT) and antimicrobial susceptibility profiles of 96 B. hyodysenteriae isolates recovered in 2014/16 belonging to 30 STs present in 30 herds in different Australian States that either were thought to be free of swine dysentery (SD), to have the disease, or to be of uncertain health status
Multiple isolates (2–10) were available for analysis from 20 of the herds, and nine of these herds had more than one ST identified. Of these 20 herds, 10 were reported not to have SD, and four of these had more than one ST identified; five of these herds were reported to have SD, and multiple different STs were found in three of these, with herd 2 having 4 STs and herd 3 having 3 STs; and five of the herds were of unknown health status, of which two had multiple STs.
The 21 isolates from the 1980s were divided into 17 STs; the 13 isolates from the 1990s into 12 STs; and the 19 isolates from the early 2000s into 10 STs (Table 3). As mentioned above, ST31 (1980s), ST50 (1990s) and ST49 (2000s) also were represented amongst the STs from 2014/16 (Table 2).
An MLST dendrogram demonstrating the relationships of the various STs for isolates for the period 2014/16 is shown as Fig 1. Four clonal complexes were identified by the eBURST program and are marked on the tree. Most isolates were relatively closely related, although ST161 and to a lesser extent ST154 were outliers. The former was weakly hemolytic, although the second weakly hemolytic isolate in ST151 was in the main body of the tree and was not closely related to ST161.
Table 3. Australian State of origin, sequence type (ST) and plasmid profile (PT) of 53 B. hyodysenteriae isolates belonging to 39 STs, isolated in the 1980s, 1990s and early 2000s and used for comparative purposes
Fig 1. MSLT dendogram showing the 30 STs of the 96 B. hyodysenteriae isolates from 2014/16. The dendrogram is based on consensus sequences constructed from combined individual distance matrices of nucleotide sequences from seven genes adh, alp, est, gdh, glpK, pgm and thi. STs shared with historic isolates found in the PubMLST database are indicated in bold (these were all of Australian origin). The STs of the two weakly hemolytic B. hyodysenteriae isolates are indicated with an asterisk. The length of the scale bar represents 10-nucleotide substitution in 100 base pairs of the sequenced gene fragment.
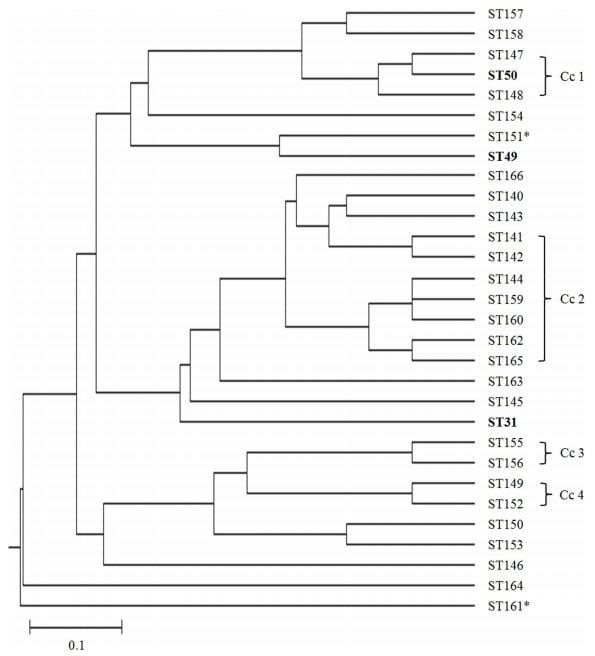
Fig 2. Minimum Spanning Tree (MST) showing relationships of the 96 B. hyodysenteriae isolates from 2014/16 and the three categories of herd health status that were originally reported. In the MST, each labeled node represents a different ST and the color represents the original reported herd health status (negative, positive or of uncertain health status). The size of the node indicates the number of strains having the same ST. Nodes with a yellow margin show the STs that are founder members of clonal complexes. The STs of the weakly hemolytic isolates identified in this study are indicated with black arrows
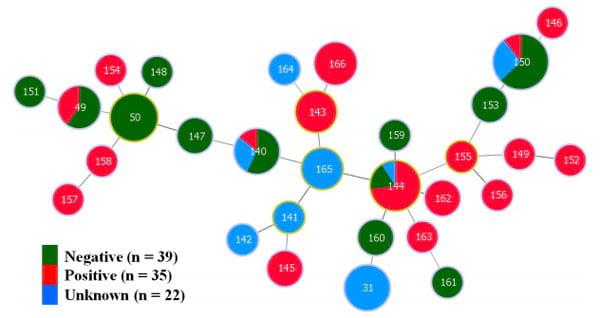
A minimum spanning tree (MST) showing the relationship of the 2014/16 isolates of B. hyodysenteriae belonging to 30 different STs and the reported disease status of their herds of origin is shown as Fig 2. The STs containing isolates from the three disease status categories were dispersed across the tree, with no clear and consistent clustering of STs related to the health status. Four STs (49, 140, 144 and 150) included multiple isolates from herds with different reported health categories.
An MST showing the relationship of the STs for the 2014/16 isolates and the STs of the 53 historic Australia isolates from the 1980s to early 2000s is presented as Fig 3. Most of the STs containing isolates from 2014/16 were located in clusters placed away from the STs containing isolates from previous decades, although there was a tendency for STs containing isolates from consecutive decades to be more closely related to each other than to those from other decades. Six STs contained multiple isolates collected in different decades, and of these STs 31 and 50 contained isolates from the 2014/16, as well as from the 1980s or 1990s, respectively. The plasmid type of the isolates also is shown.
A comparison of all the STs containing Australian isolates with those for isolates from other countries by MST is presented as Fig 4. The majority of the STs containing the Australian isolates were in broad clusters that were generally arrayed apart from the STs containing isolates from other countries. None of the STs containing Australian isolates were shared with previously described STs from other countries.
Virulence-associated plasmid genes
The distribution of members of the block of six plasmid genes in STs of B. hyodysenteriae isolates from 2014/16 is shown in Table 4 for each of the three health statuses that were originally reported. The distribution of STs with different numbers of the block of six plasmid genes was not obviously different across the three reported health statuses. Between half and three quarters of the STs in the three categories had plasmid profile 1, lacking all six genes, whilst few STs had isolates with all six of the block of plasmid genes. In Fisher’s exact test no significant differences were found between the distribution of isolates with plasmid types 1 and 7 in the three reported health statuses. Three isolates, one each from herd 73 (ST155), herd 28 (ST151), and herd 86 (ST161) respectively lacked the entire plasmid. The latter two isolates also were weakly hemolytic. Herds 5, 63, 2, 71 and 98 contained isolates with different STs that had different plasmid types (eg types 1 and 7 both were found in isolates from herds 5 and 63).
Fig 3. Minimum Spanning Tree (MST) showing relationships of the 96 B. hyodysenteriae isolates from 2014/16 and 53 previously described Australian isolates whose data was obtained from PubMLST. In the MST, each labelled node represents a different ST and the color represents the decade of origin. The size of the node indicates the number of strains having the same ST. Nodes with a yellow margin show the STs that are founder members of clonal complexes. The plasmid types of the isolates in the STs are shown with a numeral, labelled 1 to 7 as defined in Table 4. The STs of the weakly hemolytic isolates identified in this study are indicated with black arrows.
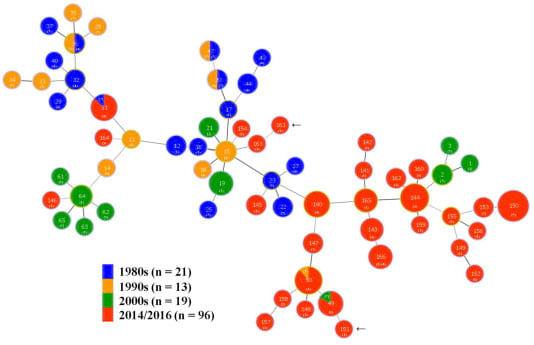
The distribution of STs with respect to members of the block of six plasmid genes in B. hyodysenteriae isolates from the 1980s, 1990s, early 2000s and 2014/16 is summarized in Table 5, and also shown in Fig 3. Apart from three isolates recovered in 2014/16, all possessed the 36 kb plasmid. A greater percentage of isolates from the 1980s and 1990s possessed all six plasmid genes (plasmid type 7) than did isolates in the early 2000s and in 2014/16, where plasmid type 1 (none of the genes present) was more common. Results of the statistical comparison between the distributions of isolates with these two plasmid types over these decades are presented in Table 6. The isolates from 2014/16 had highly significantly more isolates with plasmid type 1 compared to type 7 than did those for the 1980s and for the 1990s. The isolates from the 2000s had significantly more isolates with type 1 compared to type 7 than did the isolates from 1990s. No other differences were significant. Three herds (herds 2, 3 and 63) sampled in 2014/ 16 had multiple isolates that belonged to different STs with very different plasmid gene types (type 1 in all three herds, together with isolates of either type 7 or 4). Comparison of the three STs that included isolates both from 2014/16 and previous decades showed that the isolate in ST31 from the 1980s had plasmid type 7, as did the isolates in 2014/16; the isolate in ST50 from the 1990s had plasmid type 7 whilst the isolates from 2014/16 had plasmid type 1; and the isolate in ST49 from the early 2000s had plasmid type 7 whilst the isolates from 2014/16 had plasmid type 1. Again this is consistent with a tendency for isolates to have progressively lost plasmid genes since the 1980s.
Fig 4. Minimum Spanning Tree showing relationships of the 96 Australian B. hyodysenteriae isolates from 2014/16 in this study and 375 previously described isolates, data for which was obtained from PubMLST. The isolates that are shown originated from Australia, Austria, Belgium, Brazil, Canada, Denmark, Germany, Italy, Japan, Korea, Poland, Serbia, Sweden, the United Kingdom and the United States of America. In the MST, each labelled node represents a different ST and the color represents the country of origin. The size of the node indicates the number of strains having the same ST. The STs identified from the current study are indicated with an asterisk. Newly recognised STs are indicated with a white asterisk and previously described STs are indicated with a yellow asterisk. The positions of the weakly hemolytic isolates identified in this study are indicated with a black arrow. A red arrow indicates the position of previously described weakly hemolytic B. hyodysenteriae isolates from Germany [14] and Belgium [28].
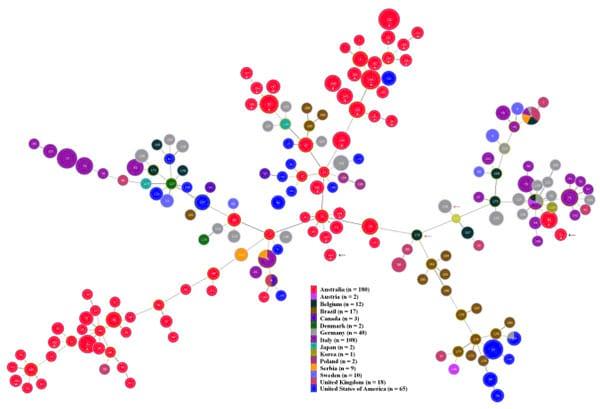
Table 4. Number and percentage of the 30 STs of B. hyodysenteriae isolates from the 2014/16 period possessing different combinations of the six plasmid-borne virulence-associated genes (plasmid types)
Table 5. Number and percentage of STs of recent and historical B. hyodysenteriae isolates possessing different combinations of the six plasmidborne virulence-associated genes
Antimicrobial susceptibility of B. hyodysenteriae isolates
The antimicrobial susceptibility status to lincomycin, tylosin and tiamulin for isolates in STs of B. hyodysenteriae isolated in 2014/16 is shown in Table 2, and a summary comparing results for 2014/16 with previously reported results for Australian isolates from 2002–2006 and 2006– 2007 [25] is presented in Table 7. Most or all isolates from all three periods were resistant to tylosin. Approximately 61% of isolates from 2014/16 were resistant to lincomycin, and this figure was considerably increased compared the results from both earlier periods. Tiamulin resistance in 2014/16 was more common (15.2%) than amongst the isolates from 2006–2007, and there was a high percentage (58.7%) of isolates of intermediate susceptibility in 2014/16. The distribution of susceptibility for tiamulin for the isolates from 2002–2006 was more similar to the data for the 2014/16 isolates than for the 2006–2007 isolates.
Isolates belonging to 4 STs (STs 150, 158, 159 and 166) were not susceptible to all three of the antimicrobials that were tested (ie were multi-drug resistant isolates), and were identified in five Victorian herds, with ST150 being present in two herds. Two of these herds supplied pigs to other herds. Other isolates of ST 150 in five other herds had different patterns of susceptibility to the three antimicrobials. Furthermore, there were examples where multiple isolates of the same ST in a single herd had different susceptibility patterns (ST 50, herd 86), or where isolates of different STs in the same herd had different susceptibility patterns (eg in herds 2 and 86)
Table 6. Matrix showing two-tailed P values of significance of differences in distribution of plasmid types 1 and 7 between isolates from the four decades using Fisher’s exact test
Table 7. Classification of the B. hyodysenteriae isolates collected in 2014–2016 as being susceptible, intermediate or resistant to the three antimicrobials, and comparison with reported results for Australian isolates from previous periods
Discussion
It is thought that pigs were introduced into Australia in 1788, arriving on ships of the first fleet from England. Subsequent imports of live pigs from different parts of the world occurred until the mid-1980s, when importation was banned. It has been presumed that carrier pigs introduced SD into Australia on one or more occasions, with subsequent spread throughout the country. In the northern hemisphere, movement of migratory water birds also has been shown to be a potential source of infection, as they may carry strains of B. hyodysenteriae, B. suanatina and B. hampsoniiz [7,9,11,29,30]: potentially such migratory avian species may be another means by which Brachyspira species have been introduced into Australia and/or transmitted between herds.
In the current study neither B. suanatina nor B. hampsoniiz were identified in the 97 herds examined, whether or not they had clinical signs of disease. These findings do not exclude the possibility that these species occur in Australia, but it shows that if they are present they are uncommon, and so are not currently an important issue for the Australian Pig Industry. Although it seems that introduction of these species by migratory water birds is unlikely to have occurred, nevertheless it would be useful to sample such species as they arrive into Australia from Asia to determine whether they carry these and other Brachyspira species. Another implication from the findings is that those strains of B. hyodysenteriae that currently are present in Australia are more likely to have evolved from strains imported with pigs before the mid-1980s, rather than having been introduced by migratory birds since then. Furthermore, the colonization of feral pigs in Australia by B. hyodysenteriae is likely to have arisen by direct or indirect transfer of strains to them from commercial pigs with SD [31].
Interestingly, members of another novel strongly beta-hemolytic spirochete that was recovered from pigs with an SD-like disease on an Australian piggery in 2007 also were not identified, suggesting that this too is an uncommon species infecting pigs [32]. On the other hand, the potentially pathogenic B. pilosicoli was identified in 40% of the herds, whilst B. intermedia was identified in 37%, and other presumed non-pathogenic weakly hemolytic Brachyspira spp in 17.5% of the herds. The latter were not investigated further by sequencing the PCR products, but are likely to have included B. innocens, B. murdochii and possibly “B. pulli” [33]. The prevalence rates for B. pilosicoli and for B. intermedia were somewhat higher than those recorded in Danish finisher pigs in herds with diarrhea (B. pilosicoli 19% of herds; B. intermedia 13%; B. innocens 34%) [34]. These results suggest that the methodologies used for isolation and identification of the Brachyspira species in this study were appropriate, and show that both B. pilosicoli and B. intermedia are widespread amongst Australian pig herds.
An important finding was the identification of B. hyodysenteriae in diagnostic samples from 14 of 24 (58.3%) herds where the consultant veterinarian/management initially considered that infection was not present. These included breeding herds that underwent heightened surveillance as part of this study, including collection of colonic mucosa from healthy pigs at the abattoir. Results for six of these herds that were initially identified as infected through serological screening have been reported previously [15]. A similar situation was reported in an early Australian study, where an isolate of B. hyodysenteriae was recovered from a pig in a highhealth status herd with minimal antimicrobial usage and no disease [35]. In this case the isolate caused SD in pigs that subsequently were experimentally challenged with it in a research facility. The occurrence of unrecognized colonization of herds by B. hyodysenteriae is not limited to Australia, as recently colonization has been demonstrated in apparently healthy breeding herds in both Switzerland [36] and Germany [14]. In addition, eight of the 24 herds (33.3%) of uncertain health status in the current study were identified as being colonized with B. hyodysenteriae. Some of these herds had pigs with mild diarrhea or colitis, but these signs had not been attributed to SD because of their mild nature. Together these findings emphasize that colonization with B. hyodysenteriae in apparently healthy herds and herds with mild disease not necessarily attributed to SD may be much more common than previously has been realized. On the other hand, in the current study only 12 of 25 (48%) herds that were already suspected to have SD based on clinical signs or past history were successfully identified as being colonized by culture and PCR of feces and/or colonic samples. This raises the possibility that even more of the “SD free” herds or those of uncertain health status might have been colonized than were detected. The results emphasize the importance of all herds routinely and regularly undertaking abattoir checks, including taking colonic mucosal samples and using specific laboratory testing to confirm their colonization status, and particularly so if they supply pigs to other herds.
The MLST method was shown to be very useful for studying the molecular epidemiology of B. hyodysenteriae in Australia, as has previously been reported [37–39]. Sequence types (STs) were equated to strains, although it was recognized that minor differences can occur between isolates in the same ST. Transfer of isolates with the same STs between herds was seen in the case of ST150 where isolates were present in a breeding herd and in other herds that received pigs from it. The finding of multiple different strains in some herds was significant, and rarely has been reported previously [40]. It is problematic as it could complicate control measures in herds where the isolates have mixed phenotypes, as for example with the different antimicrobial susceptibility patterns found amongst the different STs present in herd 86. Indeed, in this herd different isolates of ST50 also had different antimicrobial susceptibility profiles.
Overall the MLST results show that B. hyodysenteriae isolates present in Australian herds in 2014/16 were genetically diverse. Importantly, the MST presented as Fig 2 shows that isolates from herds with the three different reported health statuses were not clustered according to this status. Consequently, as previously reported [14], the disease outcome following colonization is not necessarily related to the genetic background of the isolate as reflected by its ST; instead, relative virulence potential is more likely to reside with strain-specific attributes that are not part of the core genome functions.
When comparing the Australian isolates from 20014/16 with those from previous decades, only six STs were shared in different decades (Fig 3) and there was an apparent evolutionary drift of strains over this period. Evidence for micro-evolution of B. hyodysenteriae strains over short periods of time previously has been obtained on Australian farms by comparing DNA patterns of isolates using pulsed field gel electrophoresis [40]. An accumulation of such genetic drift over time presumably contributes to the current diversity of the isolates in Australia. These changes are overlain with transmission of strains between herds through infected pigs and by indirect routes such as by movement of trucks, personnel or potential reservoir species such as feral pigs, rodents, dogs and birds. The STs containing Australian isolates all were distinct from those so far identified in other countries (Fig 4). This and the diversity found emphasizes that the current Australian strains are most likely to have originated from a number of importations of infected pigs, and that these strains have evolved considerably and independently since they were introduced.
An important practical question that arises from the study is why some herds that were colonization, including breeding herds, apparently did not have clinical signs of SD. In some cases antimicrobial use might be controlling and masking disease, although none of the herds in this study reported this as being a potential contributing factor, and it is unlikely to apply in all cases. Diet is another potential explanation, as different ingredients have been reported to influence the occurrence and severity of SD in infected pigs and herds, presumably by altering the physicochemical environment and the composition of the microbiota in the large intestine [41–48]. In this case, should isolates from healthy herds be transmitted to other herds where different diets are used then disease eventually may develop [13]. In the current study, however, the majority of herds that were tested were fed standard Australian commercial diets mainly based on wheat and plant protein, so it seems unlikely that diet is an important common contributor to the current observations.
Another possible explanation for the apparent lack of disease is that the isolates of B. hyodysenteriae recovered from healthy herds were avirulent or had reduced virulence potential, as has been described previously for some isolates in Europe and North America [49–52]. Hemolytic activity is believed to be an important virulence factor in B. hyodysenteriae [1], and genetically distinct isolates from two of the herds initially believed to be free of SD were weakly betahemolytic. This phenotype also has been reported in isolates from European pigs [28], including in an apparently avirulent isolate recovered from a healthy German breeding herd [14]. Although this finding may help to explain lack of disease in these two herds, in herd 86 another ST containing strongly hemolytic B. hyodysenteriae isolates also was found with the weakly hemolytic isolate. In this case the isolates from the other ST also might be predicted to have reduced virulence potential for other reasons, as no disease was observed.
Besides hemolysis, the block of six plasmid genes may encode other potential virulence factors that have been loosely associated with facilitating colonization [15,24,53]. Analysis of the distribution of this block of genes showed that most of the STs in the herds that were either thought to be free of SD or where the disease status was unclear did lack them, but this also commonly was the case for the isolates in STs in the herds with a history of SD (Table 4). Indeed, the relative distribution of isolates with plasmid types 1 and 7 was not significantly different between the herds from the three reported health statuses. If these genes were essential for virulence it would have been expected that they were more likely to be present in herds where disease has been reported, which they were not. One possible interpretation is that although they are not essential, their activity may tend to increase the likelihood of successful colonization by B. hyodysenteriae to levels where lesions and/or disease starts to occur [14]. Isolates belonging to ST150 were present in a breeding herd and in six production herds connected to it, only one of which had reported disease. These isolates had all six plasmid genes, but it cannot be excluded that they were defective in other genes or gene expression that reduced their ability to cause disease [14]. Herds 5 and 63, where disease was not observed, each had isolates of two different STs, and in both cases one had plasmid type 7 and the other had plasmid type 1.
Substantially more isolates from the 1980s and 1990s possessed all or most of the plasmid genes than did isolates from the early 2000s and 2014/16 (Table 5), and there were highly significant differences over this period between the number of isolates with plasmid types 1 and 7 (Table 6). In the case of the three STs that were present both in 2014/16 and in previous decades, two had plasmid type 7 in the earlier decades and plasmid type 1 in 2014/16, again supporting an evolutionary trend to lose these genes. If these genes are important in facilitating colonization this might explain the apparent tendency for the reported occurrence and severity of SD in Australia to have been reduced in recent years, despite the spirochete being widespread. This conclusion is tempered by the fact that relatively small numbers of isolates were examined, and that the isolates recovered from the 1980s and 1990s were from diagnostic samples recovered from herds with disease. Nevertheless, the isolates from the early 2000s and some of the isolates from 2014/16 also were from herds where SD had been reported. Ultimately it will be important to test both the current and historic Australian isolates in a standardized experimental infection model to evaluate their potential to colonize and cause disease.
From the trends observed it seems likely that the full plasmid was present in the strains originally introduced into Australia, and that genes gradually have been lost from the plasmid in different strains over the last 40 years. Indeed, three isolates from 2014/2016 did not have the plasmid at all. Loss of the plasmid genes by the spirochete suggests that they are not essential for viability, and indeed it may be that a loss of these genes improves and selects for survival by making the spirochete less likely to proliferate to levels that provoke inflammation and disease: in turn this would result in less antimicrobial usage to eliminate the infection. It was interesting that two of the isolates lacking the whole plasmid also were weakly hemolytic. Where collections of suitable isolates are available in other countries, studies are required to determine whether similar temporal changes in plasmid gene content and phenotype of B. hyodysenteriae strains have occurred.
The methodology used for detection of susceptibility to the three key antimicrobials used to control SD in Australia was selected to mirror that used in earlier studies, and so allow temporal comparisons of trends. It was recognized that more robust definition could have been achieved had additional antimicrobials and antimicrobial dilutions been used, but this was not the main objective of the study. The antimicrobial susceptibility patterns observed were variable, even amongst isolates with the same ST, and even where these were isolated from the same herd. For example, ST150 contained multi-resistant isolates as well as others that were susceptible to one or more of the antimicrobials tested. In this case, assuming that an otherwise identical strain was present in all the herds, it could have come under different selection pressures depending on antimicrobial exposure.
Resistance to lincomycin was substantially more common than amongst the Australian isolates collected 10–15 years earlier. An increasing trend for resistance to lincomycin also has been recorded in Australian isolates collected before 2002 [54]. As is found worldwide, resistance of B. hyodysenteriae isolates to tylosin was almost universal. The point mutation that causes tylosin resistance at position 2058 in the 23S rRNA gene is known also to increase the MICs for the lincosamide antibiotics [55], and if this occurred it also might help to explain the trend for the increased MICs to lincomycin that were found.
Resistance of B. hyodysenteriae isolates to tiamulin is an increasing problem in a number of European countries, and where it occurs it severely reduces options for control of SD [56]. In an analysis of Australian isolates collected before 2002 none were resistant to tiamulin [54], but in this study isolates belonging to four of the 30 STs (13.3%) from 2014/16 were resistant to tiamulin, with 59% showing intermediate susceptibility. This was substantially more common than amongst Australian isolates from 2006–2007, but only slightly more common than amongst isolates from 2002–2006 (Table 7). This difference may reflect the origins of the isolates: ten of the 11 resistant isolates from 2002–2006 originated from herds in Queensland, but isolates from only two Queensland herds were obtained in the 2014/16 study. The single resistant isolate from the 2006–2007 set came from Victoria, and all four of the resistant isolates from 2014/16 also came from Victorian herds (the same State where most of the sampled herds were located). These isolates also were resistant to lincomycin and tylosin (ie they were multi-drug resistant), and three of them were recovered from herds that reported having SD. Multi-resistant isolates of this type represent a significant threat to the pig industry. Given the increasing trend in resistance to these drugs, it is important that detailed information is obtained about the susceptibilities of isolates present in individual herds before control measures are implemented. Equally where susceptible isolates are present consideration should be made to eradicate the infection using the drugs that are still effective.
Conclusions
This study has demonstrated that B. hampsoniiz and B. suanatina, the newly described agents of SD in North America and Europe, are unlikely to be present in Australia. On the other hand the classical agent B. hyodysenteriae is relatively common and widespread. The strains that are currently circulating generally are different from those found in the past, are less likely to carry the block of six virulence-associated plasmid genes, and are different from strains from other countries. Evidence was found for the likely transmission of strains between piggeries that were epidemiologically linked. More of the recently isolated strains showed antimicrobial resistance than in the past, and the identification of four different multi-drug resistant strains was concerning. An important finding was the identification of B. hyodysenteriae in a large number of herds that had no disease, or only mild disease of previously unknown etiology.
Acknowledgments
The authors thank Patrick Daniel and Patricia Holyoake of the Department of Environment, Land, Water and Planning, Victoria, and consulting veterinarians from Chris Richards and Associates, Portec Veterinary Services, Ross Cutler and Associates, Rivalea Pty Ltd, Scolexia Animal & Avian Health Consultancy, SunPork Farm Supplies Pty Ltd, and ACE Laboratories for selecting and collected samples.
Author Contributions
Conceptualization:DJH TL NDP.
Data curation:TL NDP DJH.
Formal analysis:DJH.
Funding acquisition:DJH.
Investigation:TL NDP DJH.
Methodology:TL NDP DJH.
Project administration:DJH TL NDP.
Resources:TL NDP DJH.
Supervision:DJH.
Validation:TL NDP.
Visualization:DJH TL NDP.
Writing – original draft:DJH.
Writing – review & editing:DJH TL NDP.
This article was originally published in PLoS ONE 11 (12): e0167424. doi:10.1371/journal. pone.0167424. This is an Open Access article distributed under the terms of the Creative Commons Attribution License. References
1. Hampson DJ. Brachyspiral colitis. In: Zimmerman JJ, Karriker LA, Ramirez A, Schwartz KJ, Stevenson GW, (editors). Diseases of swine. Chichester: Wiley-Blackwell; 2012. p. 680–96.
2. Chander Y, Primus A, Oliveira S, Gebhart CJ. Phenotypic and molecular characterization of a novel strongly hemolytic Brachyspira species, provisionally designated “Brachyspira hampsonii”. J Vet Diagn Invest. 2012; 24(5):903–10. doi: 10.1177/1040638712456975 PMID: 22914820
3. Mirajkar NS, Phillips ND, La T, Hampson DJ, Gebhart CJ. Characterization and recognition of Brachyspira hampsonii sp. nov., a novel intestinal spirochete that is pathogenic to pigs. J Clin Microbiol. 2016; Sep 14. pii: JCM.01717-16. [Epub ahead of print].
4. Rubin JE, Costa MO, Hill JE, Kittrell HE, Fernando C, Huang Y, et al. Reproduction of mucohaemorrhagic diarrhea and colitis indistinguishable from swine dysentery following experimental inoculation with "Brachyspira hampsonii" strain 30446. PLoS ONE 2013; 8(2):e57146. doi: 10.1371/journal.pone. 0057146 PMID: 23460829
5. Costa MO, Hill JE, Fernando C, Lemieux HD, Detmer SE, Rubin JE, et al. Confirmation that "Brachyspira hampsonii" clade I (Canadian strain 30599) causes mucohemorrhagic diarrhea and colitis in experimentally infected pigs. BMC Vet Res. 2014; 10:129. doi: 10.1186/1746-6148-10-129 PMID: 24917084
6. Wilberts BL, Arruda PH, Kinyon JM, Madson DM, Frana TS, Burrough ER. Comparison of lesion severity, distribution, and colonic mucin expression in pigs with acute swine dysentery following oral inoculation with "Brachyspira hampsonii" or Brachyspira hyodysenteriae.Vet Pathol. 2014; 51(6):1096–1108. doi: 10.1177/0300985813516646 PMID: 24577722
7. Marti´nez-Lobo FJ, Hidalgo A´, Garci´a M, Argu¨ello H, Naharro G, Carvajal A, et al. First identification of "Brachyspira hampsonii" in wild European waterfowl. PLoS ONE 2013; 8:e82626. doi: 10.1371/journal. pone.0082626 PMID: 24349322
8. Mahu M, de Jong E, De Pauw N, Vande Maele L, Vandenbroucke V, Vandersmissen T, et al. First isolation of "Brachyspira hampsonii" from pigs in Europe. Vet Rec. 2014; 174(2):47.
9. Rubin JE, Harms NJ, Fernando C, Soos C, Detmer SE, Harding JC, et al. Isolation and characterization of Brachyspira spp. including "Brachyspira hampsonii" from lesser snow geese (Chen caerulescens caerulescens) in the Canadian Arctic. Microb Ecol. 2013; 66(4):813–22. doi: 10.1007/s00248-013- 0273-5 PMID: 23933825
10. Rohde J, Habighorst-Blome K, Seehusen F. "Brachyspira hampsonii" clade I isolated from Belgian pigs imported to Germany. Vet Microbiol. 2014; 168(2–4):432–35. doi: 10.1016/j.vetmic.2013.11.016 PMID: 24332829
11. Råsba¨ck T, Jansson DS, Johansson KE, Fellstro¨m C. A novel enteropathogenic, strongly haemolytic spirochaete isolated from pig and mallard, provisionally designated ’Brachyspira suanatina’ sp. nov. Environ Microbiol. 2007; 9(4):983–91. doi: 10.1111/j.1462-2920.2006.01220.x PMID: 17359270
12. Mushtaq M, Zubair S, Råsba¨ck T, Bongcam-Rudloff E, Jansson DS. Brachyspira suanatina sp. nov., an enteropathogenic intestinal spirochaete isolated from pigs and mallards: genomic and phenotypic characteristics. BMC Microbiol. 2015; 15(1):208.
13. Hampson DJ, La T, Phillips ND. Emergence of Brachyspira species and strains: reinforcing the need for surveillance. Porc Health Manag. 2015; 1:8.
14. La T, Rohde J, Phillips ND, Hampson DJ. Comparison of Brachyspira hyodysenteriae isolates recovered from pigs in apparently healthy multiplier herds with isolates from herds with swine dysentery. PLoS ONE 2016; 11(8):e0160362. 0362. doi: 10.1371/journal.pone.0160362 PMID: 27489956
15. Hampson DJ, La T, Phillips ND, Holyoake PK. Brachyspira hyodysenteriae isolated from apparently healthy pig herds following an evaluation of a prototype commercial serological ELISA. Vet Microbiol. 2016; 191:15–9. doi: 10.1016/j.vetmic.2016.05.016 PMID: 27374902
16. La T, Phillips ND, Hampson DJ. Development of a duplex PCR assay for detection of Brachyspira hyodysenteriae and Brachyspira pilosicoli in pig feces. J Clin Microbiol. 2003; 41(7):3372–5. doi: 10.1128/ JCM.41.7.3372-3375.2003 PMID: 12843096
17. Phillips ND, La T, Hampson DJ. Development of a two-step nested duplex PCR assay for the rapid detection of Brachyspira pilosicoli and Brachyspira intermedia in chicken faeces. Vet Microbiol. 2006; 116(1–3):239–45. doi: 10.1016/j.vetmic.2006.03.020 PMID: 16677782
18. Rohde J, Habighorst-Blome K. An up-date on the differentiation of Brachyspira species from pigs with nox-PCR-based restriction fragment length polymorphism. Vet Microbiol. 2012; 158(1–2):211–15. doi: 10.1016/j.vetmic.2012.02.008 PMID: 22386675
19. La T, Phillips ND, Harland BL, Wanchanthuek P, Bellgard MI, Hampson DJ. Multilocus sequence typing as a tool for studying the molecular epidemiology and population structure of Brachyspira hyodysenteriae. Vet Microbiol. 2009; 138(3–4):330–8. doi: 10.1016/j.vetmic.2009.03.025 PMID: 19369014
20. Jolley KA, Feil EJ, Chan MS, Maiden MC. Sequence type analysis and recombinational tests (START). Bioinformatics 2001; 17(12):1230–1. PMID: 11751234
21. Francisco AP, Vaz C, Monteiro PT, Melo-Cristino J, Ramirez M, Carric¸o JA. PHYLOViZ: phylogenetic inference and data visualization for sequence based typing methods. BMC Bioinform. 2012; 13:87.
22. Feil EJ, Li BC, Aanensen DM, Hanage WP, Spratt BG. eBURST: inferring patterns of evolutionary descent among clusters of related bacterial genotypes from multilocus sequence typing data. J Bacteriol. 2004; 186(5):1518–30. doi: 10.1128/JB.186.5.1518-1530.2004 PMID: 14973027
23. Bellgard MI, Wanchanthuek P, La T, Ryan K, Moolhuijzen P, Albertyn Z, et al. Genome sequence of the pathogenic intestinal spirochete Brachyspira hyodysenteriae reveals adaptations to its lifestyle in the porcine large intestine. PLoS One 2009; 4(3):e4641. doi: 10.1371/journal.pone.0004641 PMID: 19262690
24. La T, Phillips ND, Thomson JR, Hampson DJ. Absence of a set of plasmid-encoded genes is predictive of reduced pathogenic potential in Brachyspira hyodysenteriae. Vet Res. 2014; 45:131 doi: 10.1186/ s13567-014-0131-6 PMID: 25512147
25. Hampson DJ. Detection and strain typing of Brachyspira hyodysenteriae to support swine dysentery eradication and control. Report prepared for the Co-operative Research Centre for High Integrity Australian Pork. Roseworthy, South Australia. 2008; 1–39.
26. Rønne H, Szancer J. In vitro susceptibility of Danish field isolates of Treponema hyodysenteriae to chemotherapeutics in swine dysentery (SD) therapy. Interpretation of MIC results based on the pharmacokinetic properties of the antibacterial agents. In: Proceedings of the International Pig Veterinary Society Congress; 1990; Lausanne, Switzerland. p. 126.
27. Pringle M, Lande´n A, Unnerstad HE, Molander B, Bengtsson B. Antimicrobial susceptibility of porcine Brachyspira hyodysenteriae and Brachyspira pilosicoli isolated in Sweden between 1990 and 2010. Acta Vet Scand. 2012; 54:54. doi: 10.1186/1751-0147-54-54 PMID: 22998753
28. Mahu M, De Pauw N, Vande Maele L, Verlinden M, Boyen F, Ducatelle R, et al. Variation in hemolytic activity of Brachyspira hyodysenteriae strains from pigs. Vet Res. 2016; 47:66. doi: 10.1186/s13567- 016-0353-x PMID: 27338265
29. Jansson DS, Johansson KE, Olofsson T, Råsba¨ck T, Vågsholm I, Pettersson B, et al. Brachyspira hyodysenteriae and other strongly beta-haemolytic and indole-positive spirochaetes isolated from mallards (Anas platyrhynchos). J Med Microbiol. 2004; 53(Pt. 4):293–300. doi: 10.1099/jmm.0.05488-0 PMID: 15017285
30. Jansson DS, Persson M, Zimmerman U, Johansson KE. Phenotypic and genetic diversity among intestinal spirochaetes (genus Brachyspira) in free-living wild mallards (Anas platyrhynchos) sampled in southern Sweden. Syst Appl Microbiol. 2011; 34(8): 566–75. doi: 10.1016/j.syapm.2011.10.001 PMID: 22036527
31. Phillips ND, La T, Adams PJ, Harland BL, Fenwick SG, Hampson DJ. Detection of Lawsonia intracellularis, Brachyspira hyodysenteriae and/or Brachyspira pilosicoli in feral pigs. Vet Microbiol. 2009; 134 (3–4):294–99. doi: 10.1016/j.vetmic.2008.08.006 PMID: 18818030
32. Phillips ND, La T, Dunlop H, Hampson DJ. An unusual strongly haemolytic spirochaete isolated from an Australian herd with suspected swine dysentery. In: Smola J, Cizek A, Lobova D, Celer V, Prasek J, editors. Proceedings of the Fourth International Conference on Colonic Spirochaetal Infections of Animals and Humans: 20–22 May 2007. Prague, Czech Republic: The Society; 2007. p. 19.
33. Osorio J, Carvajal A, Naharro G, Rubio P, La T, Phillips ND, et al. Identification of weakly haemolytic Brachyspira isolates recovered from pigs with diarrhoea in Spain and Portugal and comparison with results from other countries. Res Vet Sci. 2013; 95(3):861–9. doi: 10.1016/j.rvsc.2013.07.014 PMID: 23928181
34. Stege H, Jensen TK, Møller K, Baekbo P, Jorsal SE. Prevalence of intestinal pathogens in Danish finishing pig herds. Prev Vet Med. 2000; 46(4):279–92. PMID: 10960714
35. Hampson DJ, Cutler R, Lee BJ. Virulent Serpulina hyodysenteriae from a pig in a herd free of clinical swine dysentery. Vet Rec. 1992; 131(14):318–9. PMID: 1441137
36. Lo¨bert S, Zimmermann W, Bu¨rki S, Frey J, Nathues H, Scheer P, et al. Occurrence of Brachyspira hyodysenteriae in multiplier pig herds in Switzerland. Tiera¨rztl Prax. 2016; 44(1):13–8.
37. Osorio J, Carvajal A, Naharro G, La T, Phillips ND, Rubio P, et al. Dissemination of clonal groups of Brachyspira hyodysenteriae between pig herds in Spain, and their relationships to isolates from other countries. PLoS ONE 2012; 7(6):e39082. doi: 10.1371/journal.pone.0039082 PMID: 22723934
38. Mirajkar NS, Gebhart CJ. Understanding the molecular epidemiology and global relationships of Brachyspira hyodysenteriae from swine herds in the United States: a multi-locus sequence typing approach. PLoS ONE 2014; 9(9):e107176. doi: 10.1371/journal.pone.0107176 PMID: 25192199
39. Rugna G, Bonilaura P, Carra E, Bergamini F, Luppi A, Gherpelli Y, et al. Sequence types and pleuromutilin susceptibility of Brachyspira hyodysenteriae isolates recovered between 2003 and 2012 from Italian pigs with swine dysentery. Vet J. 2015; 203(1):115–19.
40. Atyeo RF, Oxberry SL, Hampson DJ. Analysis of Serpulina hyodysenteriae strain variation and its molecular epidemiology using pulsed-field gel electrophoresis. Epidemiol Infect. 1999; 123(1):133–8. PMID: 10487649
41. Siba PM, Pethick DW, Hampson DJ. Pigs experimentally infected with Serpulina hyodysenteriae can be protected from developing swine dysentery by feeding them a highly digestible diet. Epidemiol Infect. 1996; 116(2):207–16. PMID: 8620913
42. Pluske JR, Siba PM, Pethick DW, Durmic Z, Mullan BP, Hampson DJ. The incidence of swine dysentery in pigs can be reduced by feeding diets that limit the amount of fermen substrate entering the large intestine. J Nutr. 1996; 116(2):2920–33.
43. Durmic Z, Pethick DW, Pluske JR, Hampson DJ. Changes in bacterial populations in the colon of pigs fed different sources of dietary fibre, and the development of swine dysentery after experimental challenge. J Appl Microbiol. 1998; 85(3):574–82. PMID: 9750287
44. Jacobson M, Fellstro¨m C, Lindberg R, Wallgren P, Jensen-Waern M. Experimental swine dysentery: comparison between infection models. J Med Microbiol. 2004; 53(Pt 4):273–80. doi: 10.1099/jmm.0. 05323-0 PMID: 15017282
45. Thomsen LE, Knudsen KE, Jensen TK, Christensen AS, Møller K, Roepstorff A. The effect of fermentable carbohydrates on experimental swine dysentery and whip worm infections in pigs. Vet Microbiol. 2007; 119(2–4):152–63. doi: 10.1016/j.vetmic.2006.09.004 PMID: 17049759
46. Hansen CF, Phillips ND, La T, Hernandez A, Mansfield J, Kim JC, et al. Diets containing inulin but not lupins help to prevent swine dysentery in experimentally infected pigs. J Anim Sci. 2010; 88(10):3327– 36. doi: 10.2527/jas.2009-2719 PMID: 20525927
47. Hansen CF, Hernandez A, Mansfield J, Hildago A, La T, Phillips ND, et al. A high dietary concentration of inulin is necessary to reduce the incidence of swine dysentery in pigs experimentally challenged with Brachyspira hyodysenteriae. Br J Nutr. 2011; 106(10):1506–13. doi: 10.1017/S000711451100208X PMID: 21736788
48. Wilberts BL, Arruda PH, Kinyon JM, Frana TS, Wang C, Magstadt DR, et al. (2014) Investigation of the impact of increased dietary insoluble fiber through the feeding of distillers dried grains with solubles (DDGS) on the incidence and severity of Brachyspira-associated colitis in pigs. PLoS ONE 9(12): e114741. doi: 10.1371/journal.pone.0114741 PMID: 25485776
49. Lysons RJ, Lemcke RM, Bew J, Burrows MR, Alexander TJL. An avirulent strain of Treponema hyodysenteriae isolated from herds free of swine dysentery. In: Proceedings of the 7th International Pig Veterinary Society Congress; 1982; Mexico City, Mexico. p. 40.
50. Jensen NS, Stanton TB. Comparison of Serpulina hyodysenteriae B78, the type strain of the species, with other S. hyodysenteriae strains using enteropathogenicity studies and restriction fragment length polymorphism analysis. Vet Microbiol. 1993; 36(3–4):221–31. PMID: 7903832
51. Achacha M, Messier S, Mittal KR. Development of an experimental model allowing discrimination between virulent and avirulent isolates of Serpulina (Treponema) hyodysenteriae. Can J Vet Res. 1996; 60(1):45–9. PMID: 8825993
52. Thomson JR, Murray BP, Henderson LE, Moore L, Meikle CS. Virulence studies in porcine Brachyspira species by experimental challenge of pigs. In: Proceedings of the Second International Conference on Colonic Spirochaetal Infections in Animals and Humans; 2003; Eddleston, Scotland. Abstract 10.
53. La T, Phillips ND, Wanchanthuek P, Bellgard MI, O’Hara AJ, Hampson DJ. Evidence that the 36kb plasmid of Brachyspira hyodysenteriae contributes to virulence. Vet Microbiol. 2011; 153(1–2):150–5. doi: 10.1016/j.vetmic.2011.02.053 PMID: 21458173
54. Karlsson M, Oxberry SL, Hampson DJ. Antimicrobial susceptibility testing of Australian isolates of Brachyspira hyodysenteriae using a new broth dilution method. Vet Microbiol. 2002; 84(1–2):123–33. PMID: 11731165
55. Karlsson M, Fellstro¨m C, Heldtander MU, Johansson E, Franklin A. Genetic basis of macrolide and lincosamide resistance in Brachyspira (Serpulina) hyodysenteriae. FEMS Microbiol Letts. 1999; 172 (2):255–60.
56. van Duijkeren E, Greko C, Pringle M, Baptiste KE, Catry B, Jukes H, et al. Pleuromutilins: use in foodproducing animals in the European Union, development of resistance and impact on human and animal health. J Antimicrob Chemother. 2014; 69(8):2022–31. doi: 10.1093/jac/dku123 PMID: 24793902