Trace minerals are a commonly ignored source of nutrients in animal feedstuffs. Their physiological roles are often underestimated and their presence in the feed in adequate quantities taken for granted. However, they are necessary to maintain body function, to optimise growth, reproduction and immune response. They therefore play an important part in determining the health status of the animal. A deficiency of these trace elements can cause a considerable reduction in performance.
Mineral requirementsIt is rather difficult to justify the term ‘requirements’ for minerals in the same way as it is for energy, protein or amino acids. Requirements for minerals are hard to establish and most estimates are based on the minimum level required to overcome a deficiency symptom and not necessarily to promote productivity or indeed, to enhance immunity. Most of the work relating to mineral requirements was carried out in the 1960s and 1970s and may not therefore apply to the modern animal. This is evident from the recent review of NRC (1998) which, with few exceptions, shows only minor differences in mineral requirements proposed by NRC (1988) or ARC (1981) (Table 1). No requirement is provided for chromium. The differences in nutrient requirements are the result of different production targets and the differing physiological status of the animal. There is therefore a paucity of information on mineral requirements for current pig genotypes; and Van Lunen and Cole (1998) have suggested that the mineral needs for growth in the modern fast-growing pig hybrids are about twice the level required by the slower growing pigs of some 20-30 years ago.
Similarly, in a trial involving sows fed to national recommendations (NRC, 1988), Mahan and Newton (1995) have shown that the body mineral content of animals at the end of the third parity was considerably lower when mean litter weaning weight at 21 days was above 60 kg, compared to below 55 kg, and for both groups it was significantly less than at first mating at 9 months of age. This suggests that considerable de-mineralisation of the sow’s skeletal structures occurred to meet the needs at the higher level of production. Thus, the higher the level of production, the greater the mineral needs of the animal.
Table 1. Dietary requirements for trace elements (mg/kg diet)*.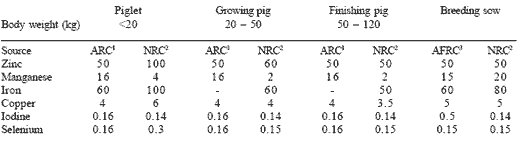
*Values represent the highest value quoted.
1ARC (1981) per kg dry matter
2NRC (1998) 90% dry matter
3AFRC (1990) 90% dry matter
These results raise questions about the actual mineral content in the diet, the availability of the minerals to the animal and the effects of mineral status of the animal on productivity. This is especially pertinent to the breeding sow, and Richards (1999) has shown that already in late gestation, the sow must rely on her liver iron (Fe) reserves to meet foetal demand for the mineral. This loss of minerals from the body is further exacerbated during lactation. If dietary intake during late pregnancy and lactation is insufficient to meet metabolic demands and the sow has to rely on her body stores, this continuous drain on body reserves results in a reduced mineral status, as shown by Damgaard Poulsen (1993). This reduced mineral status is likely to result in poorer performance.
Mineral allowancesBecause of these concerns, minerals are often provided in the diet at levels well above the ‘recommended requirements’. These are called ‘allowances’, and should take account of the class of the animal, its level of performance, as well as the source and bioavailability of the mineral. A survey of the allowances commonly provided in diets in several European countries has recently been carried out by Whittemore and associates (2002, personal communication) (Table 2). This shows the wide variation in inclusion levels, with some as high as 3-4 times those recommended in Table 1. These are provided to ensure good rates of performance and to meet the animal’s needs under the different systems of production and management, as well as to enhance immune and health status.
When determining mineral supplementation, consideration must be given to the quantity and type of raw ingredients, the processing of the diet, the storage and environmental conditions, as well as the inclusion and content of other minerals. Minerals do interact and this must be taken into account. A prime and well known example is the interaction among copper (Cu), zinc (Zn) and iron (Fe); and if high levels of Cu are used for growth-promoting purposes, then the requirements for both Zn and Fe increase. Stranks et al. (1988) proposed that in diets containing 175 mg Cu/kg, the level of Fe should be increased to 200 mg/kg diet, whereas that for Zn should be increased to 150 mg/kg diet. These values are higher than those recommended in many national standards and explain the high allowances in commercial practice. Thus, the provision of minerals is not straightforward.
Sources and bioavailability of mineralsCustomarily, inorganic salts such as sulphates, carbonates, chlorides and oxides are added to the diet to provide the correct levels to meet animal needs. These salts are hydrolysed in the digestive tract to form free ions and are absorbed. However, free ions are very reactive and can form complexes with other dietary molecules, which are difficult to absorb. The availability of the trace mineral to the animal therefore varies considerably, and under extreme conditions it may be unavailable for absorption and therefore of little benefit to the animal. Large quantities of undigested minerals are then excreted and cause environmental pollution.
Table 2. Range of dietary mineral additions in several EU countries (mg/kg feed).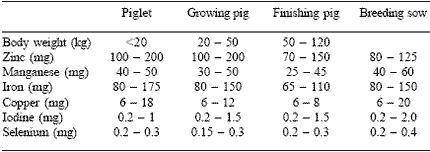
Whittemore, personal communication (2002).
Bioavailability is normally defined as the degree to which an ingested nutrient in a particular source is absorbed in a form that can be utilised or metabolised by the animal. This therefore reflects the absorption and utilisation of the nutrient ingested. Even under similar conditions, there can be quite large differences in availability. For example, in chicks, Sandoval et al. (1997) measured the bioavailability of Zn from carbonate, oxide or metal as 78, 77 and 46%, respectively, relative to that of Zn sulphate. Edwards and Baker (1999) compared the bioavailability of three sources of Zn oxide, all containing between 69 and 80% Zn, against that of Zn sulphate. Relative to that of Zn sulphate, the bioavailability of the oxide sources varied between 22 and 91%, when assessed using tibia Zn content.
They concluded that such differences have implications for animal nutrition, not only because of the higher costs per unit of available Zn, but also because unabsorbed Zn could contribute to the buildup of Zn in the soil, causing environmental pollution. In more recent studies with piglets, Damgaard Poulsen and Carlson (2001) evaluated the bioavailability of several Zn sources by regressing the rate of Zn retention and net absorption against the rate of Zn intake, provided from Zn oxide, Zn sulphate and Zn acetate. Surprisingly, the difference in bioavailability among the different sources was small: 22% for Zn oxide, 23% for Zn sulphate and 19% for Zn acetate. It was anticipated that the bioavailability of Zn from the sulphate and acetate sources would have been considerably higher than that from the oxide source. In fact, daily Zn retention reached a plateau of ~40 mg/day at a dietary intake of 180 mg Zn/day.
Relative to Zn sulphate, the bioavailability of Zn from the oxide and acetate sources was 95 and 85%, respectively. These compare with values of 67-87% determined by Wedekind et al. (1994) based on metacarpal, coccyginal and plasma Zn content. Thus, the estimate of bioavailability may depend on the response trait measured.
Nevertheless, the bioavailability values in the studies of Damgaard Poulsen and Carlson (2001) show that there were no major differences in the bioavailability of Zn from the different inorganic sources, implying that 75-80% of the ingested Zn is excreted by the animals.
For this reason, there is growing interest in organic, that is proteinated or chelated trace minerals. In this form, the trace element is chemically bound to a chelating agent or ligand, usually a mixture of amino acids or small peptides. This makes them more bioavailable and bioactive and provides the animal with a metabolic advantage that often results in improved performance. They can therefore be included at much lower levels without compromising performance, thus minimising nutrient excretion and environmental impact.
Environmental implicationsCopper and Zn are of particular concern as regards environmental impact, since inorganic sources (Cu sulphate and Zn oxide) are often fed at pharmacological levels that are well above the physiological requirements of the animals in order to promote growth rate and to prevent bacterial diarrhoea. Their excretion contributes to soil and water pollution and may well be toxic to plants and animals. They are also the most likely to be toxic to the microflora in the soil. It is for this reason that the Animal Feed Committee of the European Union has proposed maximum inclusion levels that are well below current authorised levels (Table 3). These values refer to the total content in the feed, including that present in the raw ingredients.
Table 3. Current and proposed maximum levels of dietary Cu and Zn in the EU.
CopperCopper is required for the proper functioning of the central nervous-, the immune- and cardiovascular systems, as well as for pigmentation of the skin. Although the minimum requirement is only 5-10 ppm, higher levels stimulate growth. Copper, and Cu sulphate in particular, has therefore been added to pig diets as a growth enhancer. However, recent studies suggest that organic sources of Cu may be more effective in promoting growth than Cu sulphate, as well as minimising nutrient excretion.
Cereal grains normally contain between 4-6 ppm Cu, whereas oilseeds have a higher content (15-30 ppm). Fish meals normally contain less than 10 ppm. Smits and Henman (2000) evaluated the performance of grower and finisher pigs fed diets supplemented with either Cu sulphate (150 ppm Cu) or organic Cu (40 ppm Cu as Bioplex
TM Cu). Those pigs fed the diets with the organic Cu at 40 ppm achieved similar levels of performance (P>0.05) to those fed 150 ppm Cu from Cu sulphate. However, there was a significant reduction in the quantity of Cu excreted in the faeces. In faeces from pigs fed 40 ppm Bioplex
TM Cu, Cu concentration was 3 to 4 times lower than when fed Cu sulphate (Table 4).
Table 4. The growth performance and faecal Cu excretion of pigs fed different Cu sources.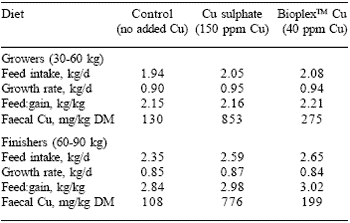
Smits and Henman (2000)
In a subsequent study, they further confirmed the significant reduction in faecal Cu when Bioplex
TM Cu (50 ppm Cu) was compared with Cu sulphate (160 ppm Cu). They concluded that by replacing high levels of Cu sulphate with lower levels of organic (Bioplex
TM) Cu it is possible to maintain the growth-enhancing effect of the diet, but since the excretion of Cu was dramatically reduced, it will be achieved in an eco-friendly and responsible way.
Studies by Carlson (2001) and Wu et al. (2001) also reported that piglets in the post-weaning period were able to maintain growth performance when 50-100 ppm Cu was provided from Bioplex
TM Cu, compared with the customary level of 250 ppm Cu from Cu sulphate. Additionally, they measured the rates of absorption and retention not only of Cu, but also of Zn and Fe. It is well known that Cu can interact negatively with Zn and Fe, and vice versa.
As expected, increasing the dietary content of Cu increased both the rate of absorption and retention of Cu, but again, faecal excretion of Cu was lower with Bioplex
TM Cu than with Cu sulphate. Piglets fed the 50 or 100 ppm Bioplex
TM Cu also had a higher rate of Zn absorption and retention, with lower faecal Zn excretion (P<0.05), probably due to the higher availability of the organic Cu. The rate of absorption and retention of Fe was also higher on the diets containing Bioplex
TM Cu compared with Cu sulphate. This indicated that the use of organic sources of Cu did not interfere with Zn or Fe metabolism, unlike the inorganic Cu sources (Table 5).
Table 5. Absorption and retention of Cu, Zn and Fe in pigs fed different Cu sources.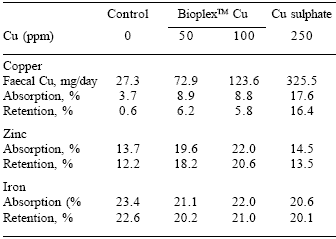
Carlson (2001)
ZincZinc is a component of many metabolic functions and plays a vital role in hormone secretion, especially those relating to growth, reproduction, immunocompetence and stress. It is involved in the process of keratin generation and in collagen and skin nucleic acid synthesis.
The natural content of Zn in cereals is about 20- 40 ppm. Oilseed co-products, fish meal and meat and bone meal all have higher content and may contain up to 100 ppm. However, Zn interacts with other minerals and Zn deficiency has been observed in high calcium diets. Phytic acid will reduce the availability, but this can be partly redressed by the use of phytase enzymes. A supplement of Zn is therefore required under most practical conditions. In terms of immunocompetence, Zn has a positive effect on both the immune response to pathogens and the prevention of disease by maintaining healthy epithelial tissue. In this respect, Zn oxide (ZnO) is usually added at high inclusion levels (2-3 kg/ton) to piglet diets because of its known pharmacological effects, increasing growth rate and reducing the incidence of bacterial diarrhoea. Carlson et al. (1998) also reported that high levels of ZnO altered duodenal morphology (deeper crypts and greater total thickness) and increased intestinal metallothionein concentration, which indicated that high amounts of Zn may also have an enteric effect on the pig. However, the availability of ZnO, compared with Zn sulphate and organic Zn, is low and there is increasing concern about the high content of Zn in slurry and the ensuing environmental impact. Consequently, producers are more and more looking at alternative sources of Zn.
A recent study by Carlson (2000) involved organic Zn (Bioplex
TM Zn) and ZnO in the 4-week period post weaning. Piglets were weaned at 21 days of age and allocated to different dietary treatments. There were seven treatments: control (no added Zn), ZnO (2000 ppm) and 50, 100, 200, 400 and 800 ppm organic Zn (Bioplex
TM Zn). Over the entire 28-day nursery study, dietary Zn treatment had no effect on growth performance, feed intake or feed efficiency (Table 6). During the first two weeks of the study, the piglets fed either 50 or 100 ppm Zn as Bioplex
TM Zn had the greatest average daily gain (P<0.01), compared to those on the other dietary Zn treatments. It was concluded that feeding 50 to 100 ppm Zn as Bioplex
TM Zn may replace 2000 ppm Zn as Zn oxide.
Mullan (2002, this volume) has recently shown that piglets fed 100 ppm Zn from BioplexTM Zn had the same growth rate as those fed 1500-2250 ppm Zn from Zn oxide, but those piglets fed 250 ppm Zn from Bioplex
TM Zn had superior growth rate (P<0.01). Piglets fed the diets containing both levels of Bioplex
TM Zn had significantly reduced levels of Zn in faeces compared with those fed ZnO; indeed it was no higher than that in the faeces of the control piglets fed no supplemental Zn. Wu et al. (2001), on the other hand, reported that piglets fed 2000 ppm Zn from ZnO had higher growth rates than those fed Bioplex
TM Zn. Those fed ZnO numerically retained the most Zn, but the proportion of Zn absorbed and retained in relation to Zn intake was less than that for the piglets fed either 200 or 400 ppm Bioplex
TM Zn. The piglets fed the ZnO also excreted more than four times as much Zn as those receiving organic (Bioplex
TM) Zn (Table 7). The absorption and retention of Cu and Fe was highest for piglets fed 2000 ppm ZnO.
Table 6. Effect of supplemental Zn on piglet performance.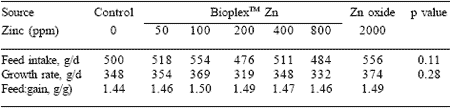
Carlson (2000)
Table 7. Absorption and retention of Zn, Cu and Fe in pigs fed different Zn sources.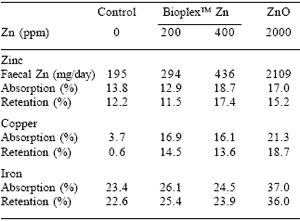
Wu et al. (2001)
ConclusionsTrace mineral nutrition has been a particularly neglected area of pig science and modern genotypes, with higher levels of productivity, may require higher levels than are currently recommended. However, it is not just a question of quantity, but very much a question of the source and bioavailability of the mineral. The benefits of including trace minerals at the level required by the modern animal, and in the most readily absorbed form, are measurable in increased performance, better health and welfare and in reduced environmental impact.
In this respect, organic minerals will play an increasing role in pig nutrition, not only for meeting the true requirements of the animal for optimal performance but also for causing minimal environmental impact. Indeed at the low levels recommended by many national organisations, it is likely that the only way these criteria can be achieved is through the use of organic minerals.
ReferencesAgricultural and Food Research Council (AFRC). 1990. Nutrient Requirements of Sows and Boars, Nutrition Abstracts and Reviews, Series B, 60. TCORN, Report No. 4:383.
Agricultural Research Council (ARC). 1981. The Nutrient Requirements of Pigs. Commonwealth Agricultural Bureaux, Slough.
Carlson, M.S. 2000. A closer look at inorganic and organic copper and zinc supplementation in nursery pig diets. In: Biotechnology in the Feed Industry: Proceedings of Alltech’s 16th Annual Symposium (T.P. Lyons and K.A. Jacques, eds), Nottingham University Press, Nottingham, UK, pp. 301-308.
Carlson, M.S. 2001. Dietary trace elements for the growing pig. In: Concepts in Pig Science (T.P. Lyons and D.J.A. Cole, eds), Nottingham University Press, Nottingham, pp. 147-156.
Carlson, M.S., S.L. Hoover, G.M. Hill, J.E. Link and R.J. Turk. 1998. Effect of pharmacological zinc on intestinal metallothionein concentration and morphology in nursery pigs. J. Anim. Sci. 76 (Suppl.1), p. 57 (Abstr).
Damgaard Poulsen, H. 1993. Minerals for sows. Significance of main effects and interactions on performance and biochemical traits. PhD Thesis: University of København.
Damgaard Poulsen, H. and D. Carlson. 2001. Bioavailability of zinc from different zinc sources. Paper N5.8. Proc. European Assoc. of Anim. Production Annual Meeting.
Edwards, H.M. and D.H. Baker. 1999. Bioavailability of zinc in several sources of zinc oxide, zinc sulphate and zinc metal. J. Anim. Sci. 77:2730.
Mahan, D.C. and E.A. Newton. 1995. Effect of initial breeding weight on macro- and micromineral composition over a three-parity period using a high-producing sow genotype. J. Anim. Sci. 73:151.
National Research Council. 1988. Nutrient Requirements of Swine. 9th Revised Edition. National Academy Press, Washington.
National Research Council. 1998. Nutrient Requirements of Swine. 10th Revised Edition. National Academy Press, Washington.
Richards, M.P. 1999. Zinc, copper and iron metabolism during porcine fetal development. Biological Trace Element Research 69:27.
Sandoval, M., P.R. Henry, C.B. Ammerman, R.D. Miles and R.C. Littell. 1997. Relative bioavailability of supplemental inorganic zinc sources for chicks. J. Anim. Sci. 75:3195.
Smits, R.J. and D.J. Henman. 2000. Practical experiences with Bioplexes in intensive pig production. In: Biotechnology in the Feed Industry, Proceedings of Alltech’s 16th Annual Symposium (T.P. Lyons and K.A. Jacques, eds), Nottingham University Press, Nottingham, pp. 293-300.
Stranks, M.H., B.C. Cooke, C.B. Fairbairn, N.G. Fowler, P.S. Kirby, K.J. McCracken, C.A. Morgan, F.G. Palmer and D.G. Peers. 1988. Nutrient allowance for growing pigs. Research and Development in Agriculture 5:71.
Van Lunen, T.A. and D.J.A. Cole. 1998. Growth and body composition of highly selected boars and gilts. Animal Science 67:107.
Wedekind, K.J., A.J. Lewis, M.A. Giesemann and P.S. Miller. 1994. Bioavailability of zinc from inorganic and organic sources for pigs fed cornsoybean meal diets. J. Anim. Sci. 72:2681.
Wu, C., A. Tsunoda, D.W. Bollinger, M.S. Carlson, T.L. Veum and G.W. Tibbetts. 2001. Reducing pharmacological levels of copper and zinc in nursery pig diets: response to zinc and copper proteinates. In: Biotechnology in the Feed Industry: Proceedings. of Alltech’s 17th Annual Symposium (T.P. Lyons and K.A. Jacques, eds), Nottingham University Press, Nottingham, pp. 285-295.